Abstract
The synthesis and accumulation of Chlamydia trachomatis outer membrane proteins within infected HeLa 229 host cells were monitored by assessing the uptake of [35S]cysteine into chlamydial proteins during the 48-h growth cycle of a lymphogranuloma venereum strain, L2/434/Bu. Synthesis of the major outer membrane protein, a protein that accounts for about 60% of the outer membrane protein mass of elementary bodies (EB), was first detected between 12 and 18 h after infection. The uptake of [35S]cysteine into the 60,000-molecular-weight doublet (60K doublet) and 12.5K cysteine-rich proteins was not observed until 30 h after infection, when the intracellularly dividing reticulate bodies were beginning to transform into infectious EBs. By using a more sensitive immunoblotting method in conjunction with monoclonal antibodies specific for the 60K doublet proteins, synthesis of these proteins was detected even earlier, by 18 h after infection. These data suggest that the time and extent of synthesis of these outer membrane proteins are regulated by processes that coincide in time with the transformation of reticulate bodies into EBs. Additional studies were performed to determine the extent of disulfide cross-linking of outer membrane proteins during the growth cycle. Both the major outer membrane protein and the 12.5K protein became progressively cross-linked to about 60% during the last 24 h of the growth cycle, whereas the 60K doublet proteins were extensively cross-linked during most of the cycle. These data may indicate an intracellular cross-linking mechanism, possibly enzymatic, that exists in addition to an auto-oxidation mechanism that occurs upon host cell lysis and exposure to the extracellular environment.
Full text
PDF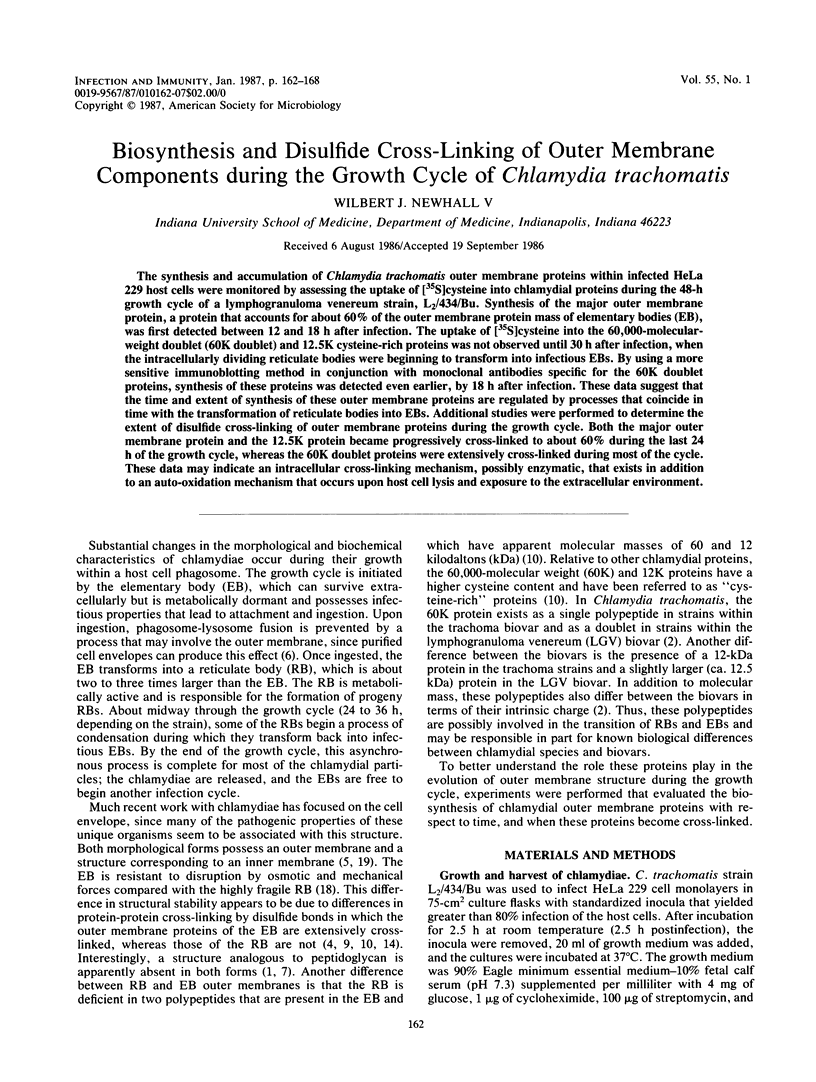
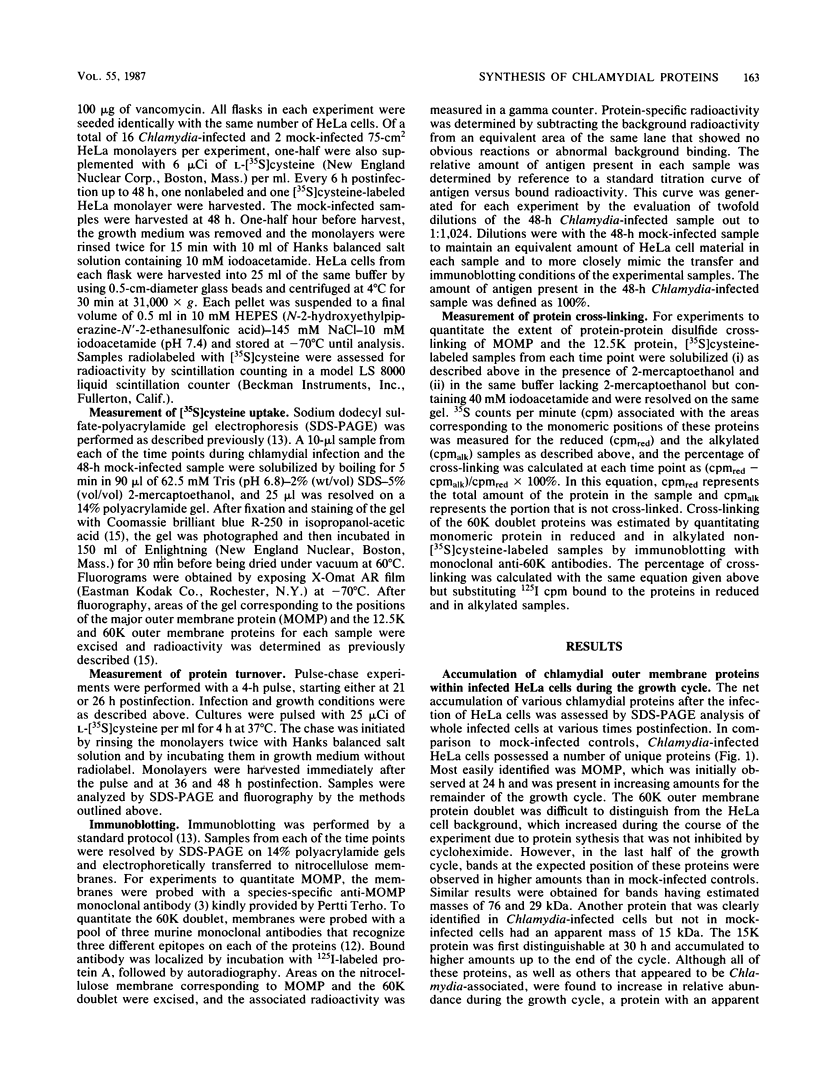
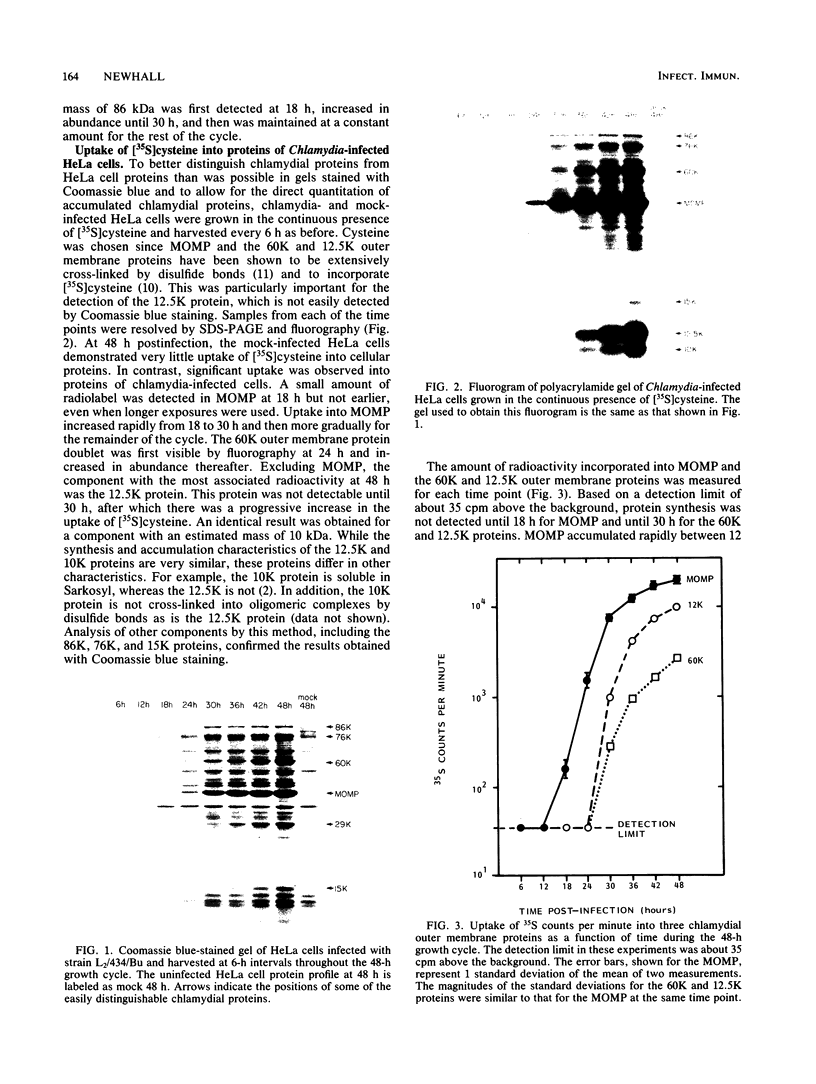
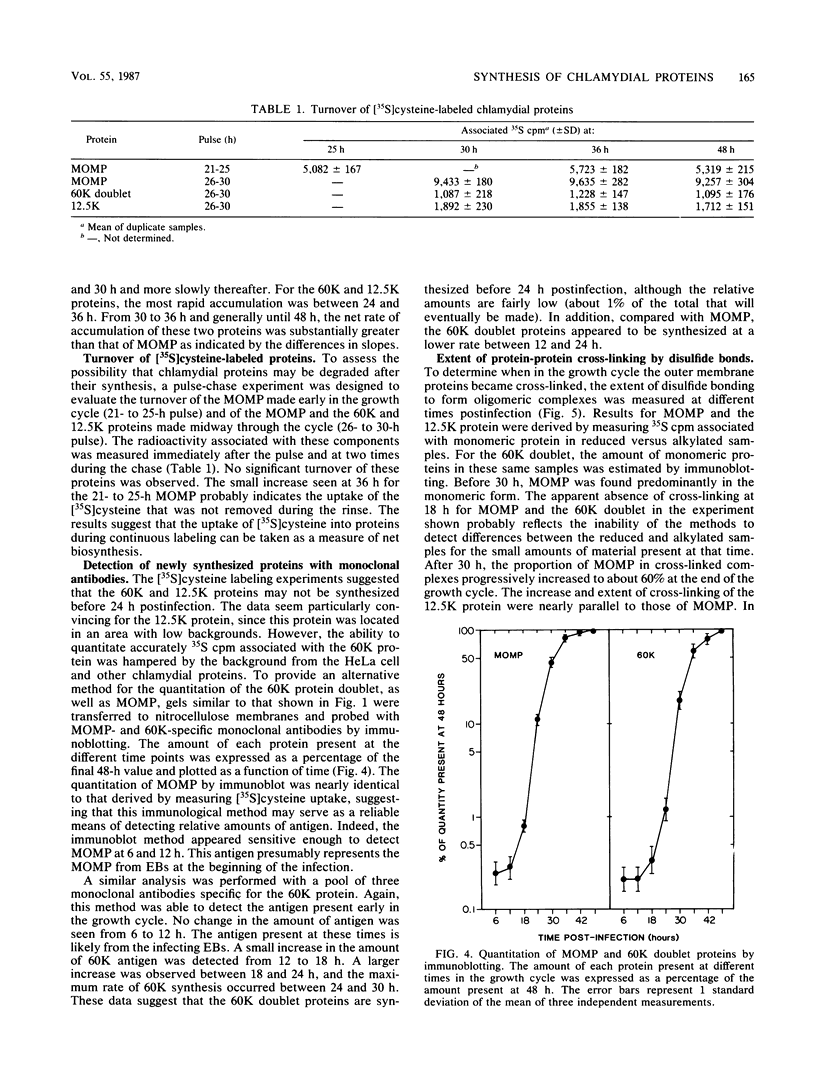
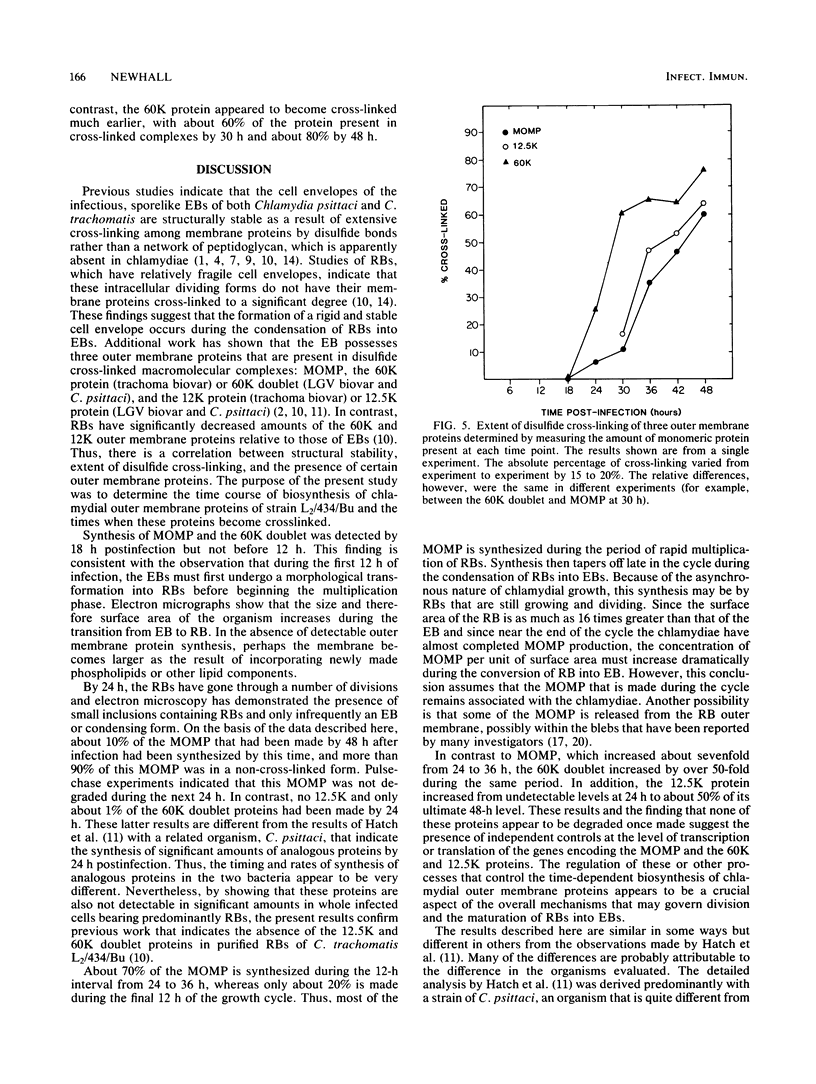
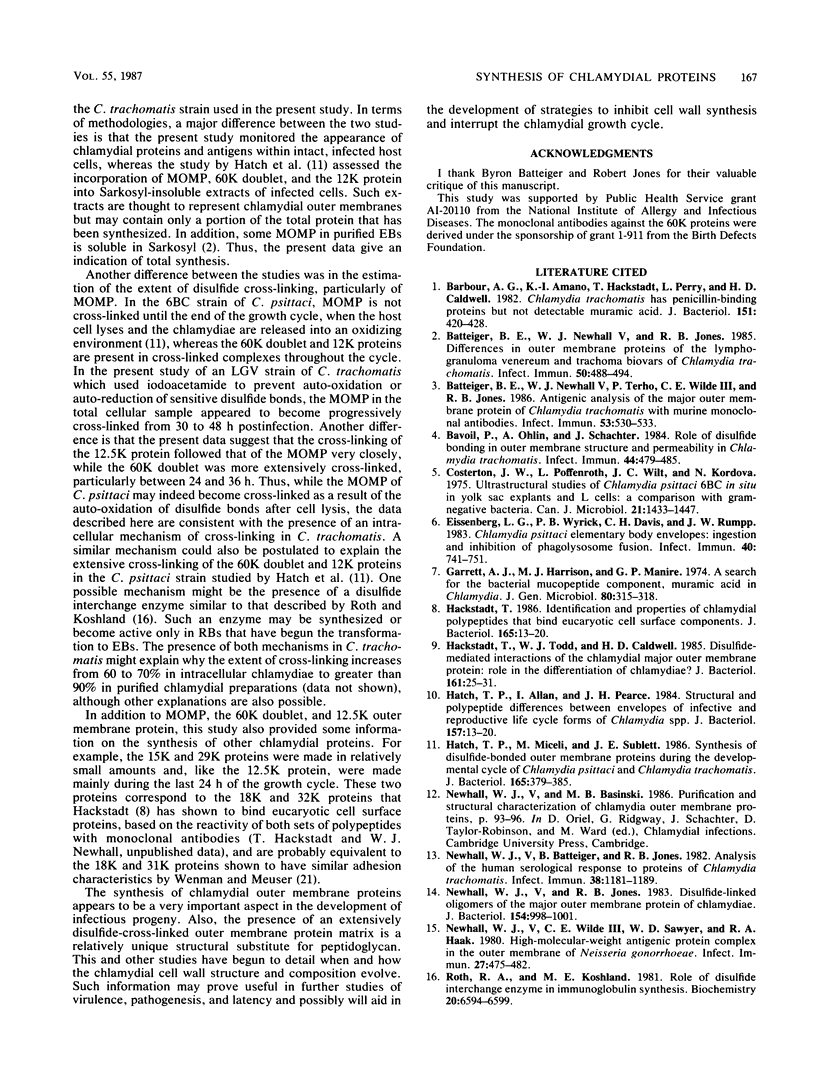
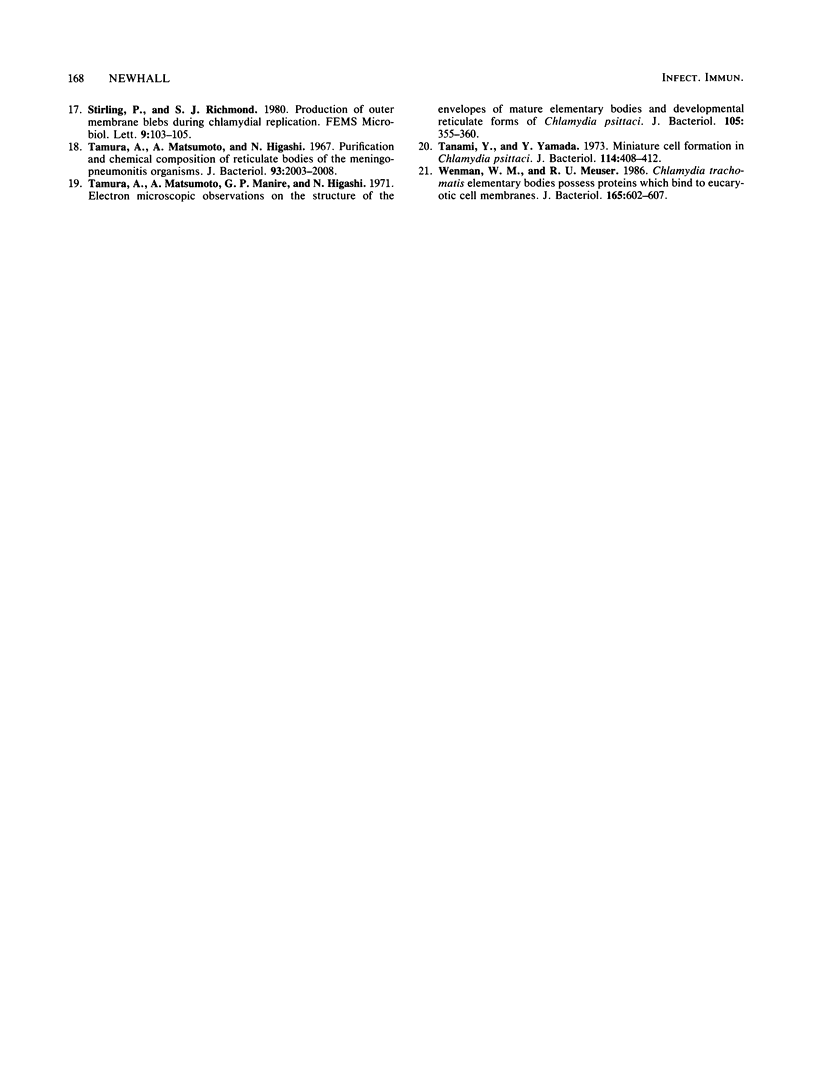
Images in this article
Selected References
These references are in PubMed. This may not be the complete list of references from this article.
- Barbour A. G., Amano K., Hackstadt T., Perry L., Caldwell H. D. Chlamydia trachomatis has penicillin-binding proteins but not detectable muramic acid. J Bacteriol. 1982 Jul;151(1):420–428. doi: 10.1128/jb.151.1.420-428.1982. [DOI] [PMC free article] [PubMed] [Google Scholar]
- Batteiger B. E., Newhall W. J., 5th, Jones R. B. Differences in outer membrane proteins of the lymphogranuloma venereum and trachoma biovars of Chlamydia trachomatis. Infect Immun. 1985 Nov;50(2):488–494. doi: 10.1128/iai.50.2.488-494.1985. [DOI] [PMC free article] [PubMed] [Google Scholar]
- Batteiger B. E., Newhall W. J., 5th, Terho P., Wilde C. E., 3rd, Jones R. B. Antigenic analysis of the major outer membrane protein of Chlamydia trachomatis with murine monoclonal antibodies. Infect Immun. 1986 Sep;53(3):530–533. doi: 10.1128/iai.53.3.530-533.1986. [DOI] [PMC free article] [PubMed] [Google Scholar]
- Bavoil P., Ohlin A., Schachter J. Role of disulfide bonding in outer membrane structure and permeability in Chlamydia trachomatis. Infect Immun. 1984 May;44(2):479–485. doi: 10.1128/iai.44.2.479-485.1984. [DOI] [PMC free article] [PubMed] [Google Scholar]
- Costerton J. W., Poffenroth L., Wilt J. C., Kordová N. Ultrastructural studies of Chlamydia psittaci 6BC in situ in yolk sac explants and L cells: a comparison with gram-negative bacteria. Can J Microbiol. 1975 Oct;21(10):1433–1447. doi: 10.1139/m75-215. [DOI] [PubMed] [Google Scholar]
- Eissenberg L. G., Wyrick P. B., Davis C. H., Rumpp J. W. Chlamydia psittaci elementary body envelopes: ingestion and inhibition of phagolysosome fusion. Infect Immun. 1983 May;40(2):741–751. doi: 10.1128/iai.40.2.741-751.1983. [DOI] [PMC free article] [PubMed] [Google Scholar]
- Garrett A. J., Harrison M. J., Manire G. P. A search for the bacterial mucopeptide component, muramic acid, in Chlamydia. J Gen Microbiol. 1974 Jan;80(1):315–318. doi: 10.1099/00221287-80-1-315. [DOI] [PubMed] [Google Scholar]
- Hackstadt T. Identification and properties of chlamydial polypeptides that bind eucaryotic cell surface components. J Bacteriol. 1986 Jan;165(1):13–20. doi: 10.1128/jb.165.1.13-20.1986. [DOI] [PMC free article] [PubMed] [Google Scholar]
- Hackstadt T., Todd W. J., Caldwell H. D. Disulfide-mediated interactions of the chlamydial major outer membrane protein: role in the differentiation of chlamydiae? J Bacteriol. 1985 Jan;161(1):25–31. doi: 10.1128/jb.161.1.25-31.1985. [DOI] [PMC free article] [PubMed] [Google Scholar]
- Hatch T. P., Allan I., Pearce J. H. Structural and polypeptide differences between envelopes of infective and reproductive life cycle forms of Chlamydia spp. J Bacteriol. 1984 Jan;157(1):13–20. doi: 10.1128/jb.157.1.13-20.1984. [DOI] [PMC free article] [PubMed] [Google Scholar]
- Hatch T. P., Miceli M., Sublett J. E. Synthesis of disulfide-bonded outer membrane proteins during the developmental cycle of Chlamydia psittaci and Chlamydia trachomatis. J Bacteriol. 1986 Feb;165(2):379–385. doi: 10.1128/jb.165.2.379-385.1986. [DOI] [PMC free article] [PubMed] [Google Scholar]
- Newhall W. J., Batteiger B., Jones R. B. Analysis of the human serological response to proteins of Chlamydia trachomatis. Infect Immun. 1982 Dec;38(3):1181–1189. doi: 10.1128/iai.38.3.1181-1189.1982. [DOI] [PMC free article] [PubMed] [Google Scholar]
- Newhall W. J., Jones R. B. Disulfide-linked oligomers of the major outer membrane protein of chlamydiae. J Bacteriol. 1983 May;154(2):998–1001. doi: 10.1128/jb.154.2.998-1001.1983. [DOI] [PMC free article] [PubMed] [Google Scholar]
- Newhall W. J., Wilde C. E., 3rd, Sawyer W. D., Haak R. A. High-molecular-weight antigenic protein complex in the outer membrane of Neisseria gonorrhoeae. Infect Immun. 1980 Feb;27(2):475–482. doi: 10.1128/iai.27.2.475-482.1980. [DOI] [PMC free article] [PubMed] [Google Scholar]
- Roth R. A., Koshland M. E. Role of disulfide interchange enzyme in immunoglobulin synthesis. Biochemistry. 1981 Nov 10;20(23):6594–6599. doi: 10.1021/bi00526a012. [DOI] [PubMed] [Google Scholar]
- Tamura A., Matsumoto A., Higashi N. Purification and chemical composition of reticulate bodies of the meningopneumonitis organisms. J Bacteriol. 1967 Jun;93(6):2003–2008. doi: 10.1128/jb.93.6.2003-2008.1967. [DOI] [PMC free article] [PubMed] [Google Scholar]
- Tamura A., Matsumoto A., Manire G. P., Higashi N. Electron microscopic observations on the structure of the envelopes of mature elementary bodies and developmental reticulate forms of Chlamydia psittaci. J Bacteriol. 1971 Jan;105(1):355–360. doi: 10.1128/jb.105.1.355-360.1971. [DOI] [PMC free article] [PubMed] [Google Scholar]
- Tanami Y., Yamada Y. Miniature cell formation in Chlamydia psittaci. J Bacteriol. 1973 Apr;114(1):408–412. doi: 10.1128/jb.114.1.408-412.1973. [DOI] [PMC free article] [PubMed] [Google Scholar]
- Wenman W. M., Meuser R. U. Chlamydia trachomatis elementary bodies possess proteins which bind to eucaryotic cell membranes. J Bacteriol. 1986 Feb;165(2):602–607. doi: 10.1128/jb.165.2.602-607.1986. [DOI] [PMC free article] [PubMed] [Google Scholar]