Abstract
The interaction of C3 and terminal complement components with three isogenic strains of Escherichia coli O111B4 varying in outer membrane and capsule composition was examined. Strains CL99 and 1-1, which possess O-antigen capsule and 74 to 77% coverage of lipid A core oligosaccharide, were sensitive to killing in pooled normal human serum (PNHS) or magnesium ethylene glycoltetraacetic acid PNHS in the presence but not the absence of antibody, although 1-1 contained 35% more lipopolysaccharide than CL99 and was slightly less sensitive to alternative pathway killing. In contrast, strain 1-2 lacks O-antigen capsule but contains 84% coverage and resists serum killing in the presence and absence of antibody in both PNHS and magnesium ethylene glycoltetraacetic acid PNHS. All three strains consumed C3 and C9 when incubated in PNHS, but consumption was most rapid with 1-2, which also bound the largest number of C3 molecules per CFU. Between 15 X 10(3) and 24 X 10(3) molecules of C9 per CFU bound to CL99 and 1-1 during incubation in 10% PNHS or 10% magnesium ethylene glycoltetraacetic acid PNHS, and binding was relatively stable. Binding and release of 3 X 10(3) to 8 X 10(3) molecules of C9 per CFU was observed for strain 1-2. The majority of C9 bound to CL99 and 1-1 in the presence of antibody distributed with the outer membrane after lysis of the organisms in a French press, whereas only 16.1 to 20.1% of C9 was deposited on these organisms in the absence of antibody, and 31.5 to 39.8% of C9 on strain 1-2 with or without antibody sedimented with the outer membrane. Between 4.6 X 10(3) and 5.5 X 10(3) molecules of C9 per CFU remained bound in a salt- and trypsin-resistant form to the outer membrane of organisms that were killed, whereas fewer than 1.4 X 10(3) molecules of C9 per CFU were bound to the outer membrane of organisms not killed by serum. These results indicate that C5b-9 that is bound to the outer membrane of E. coli O111B4 in a form resistant to salt or protease elution correlates with bacterial killing.
Full text
PDF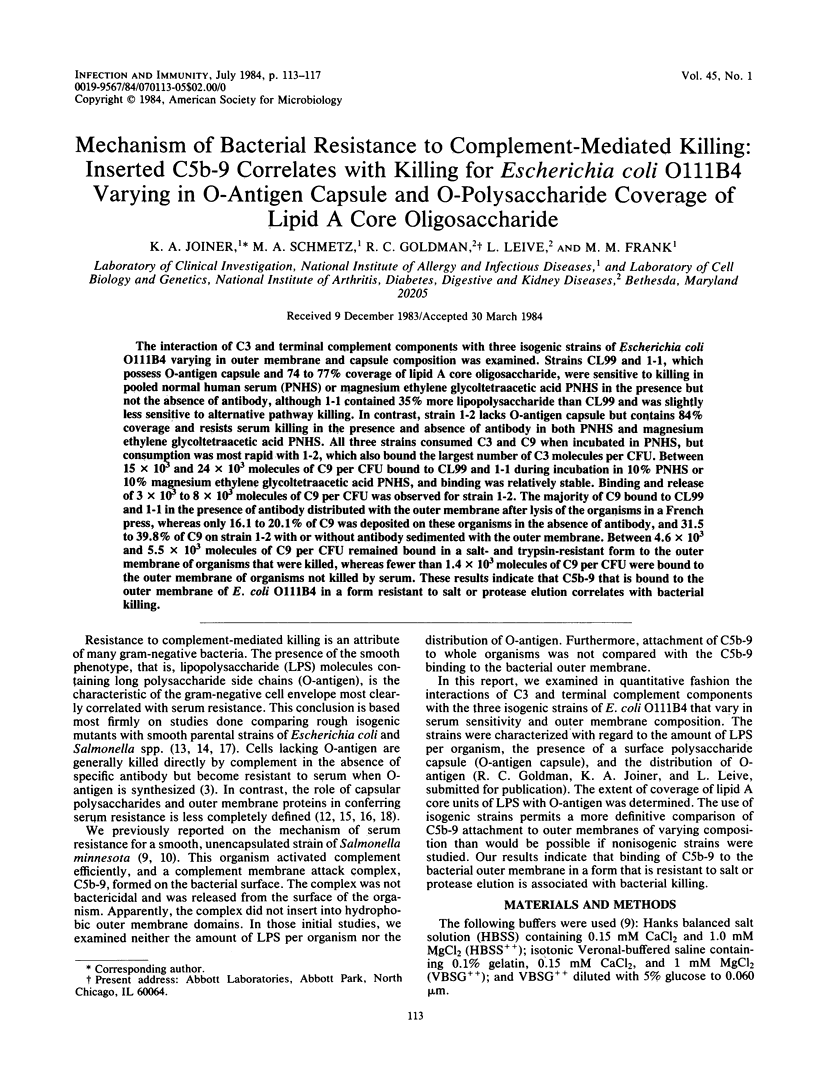
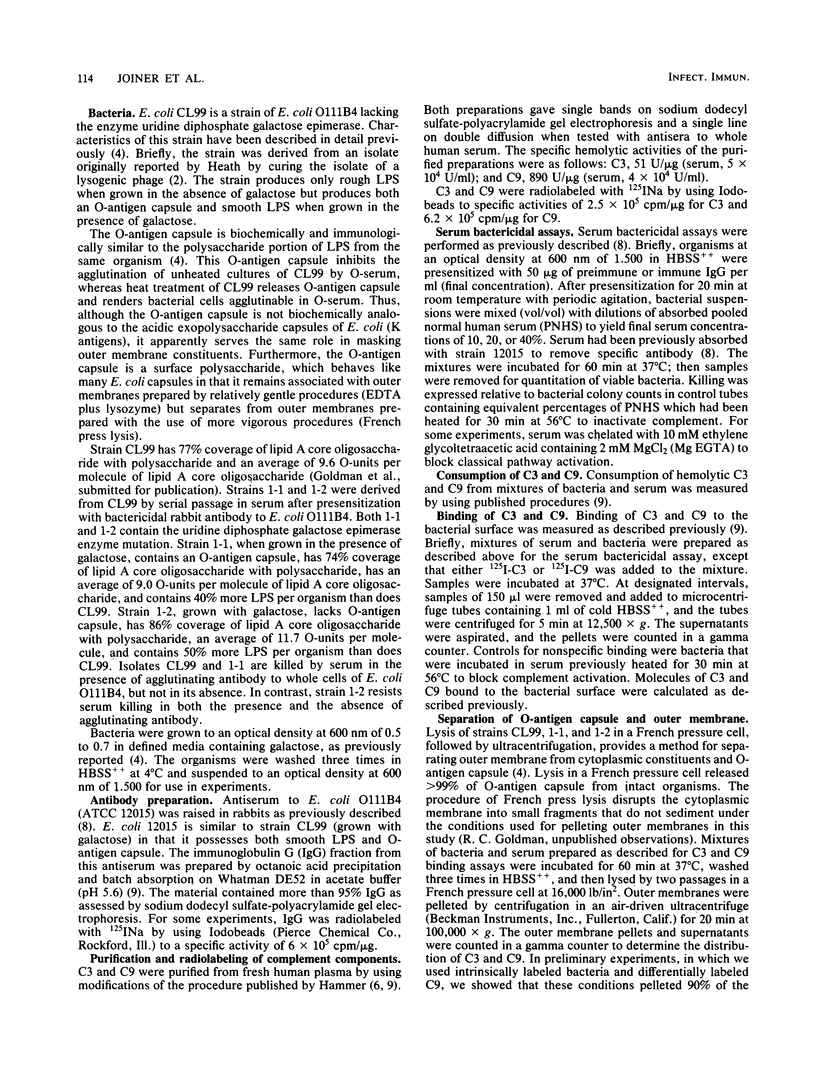
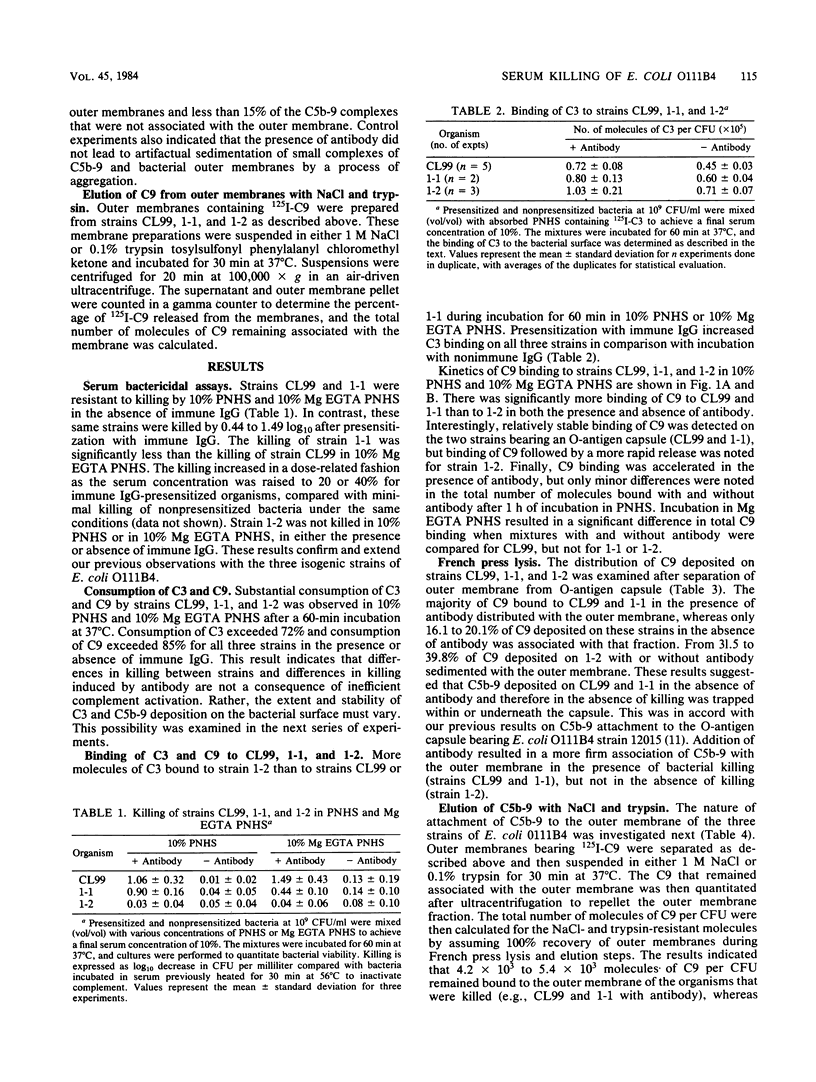
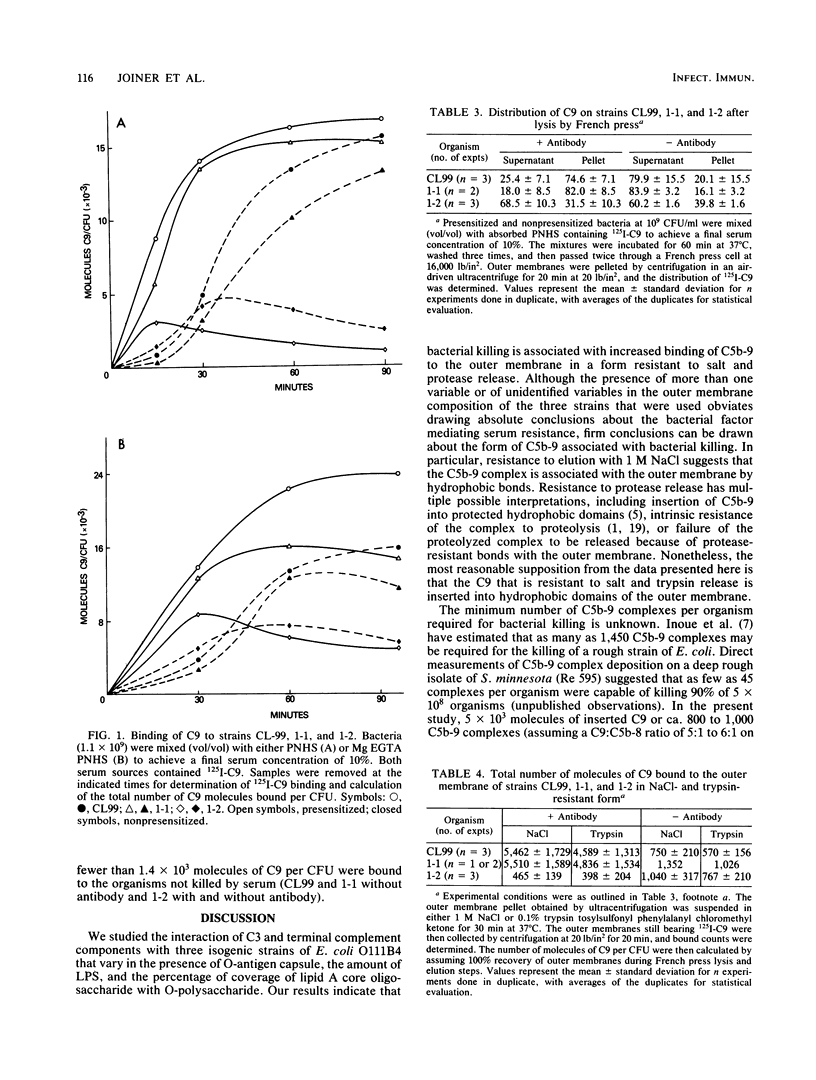
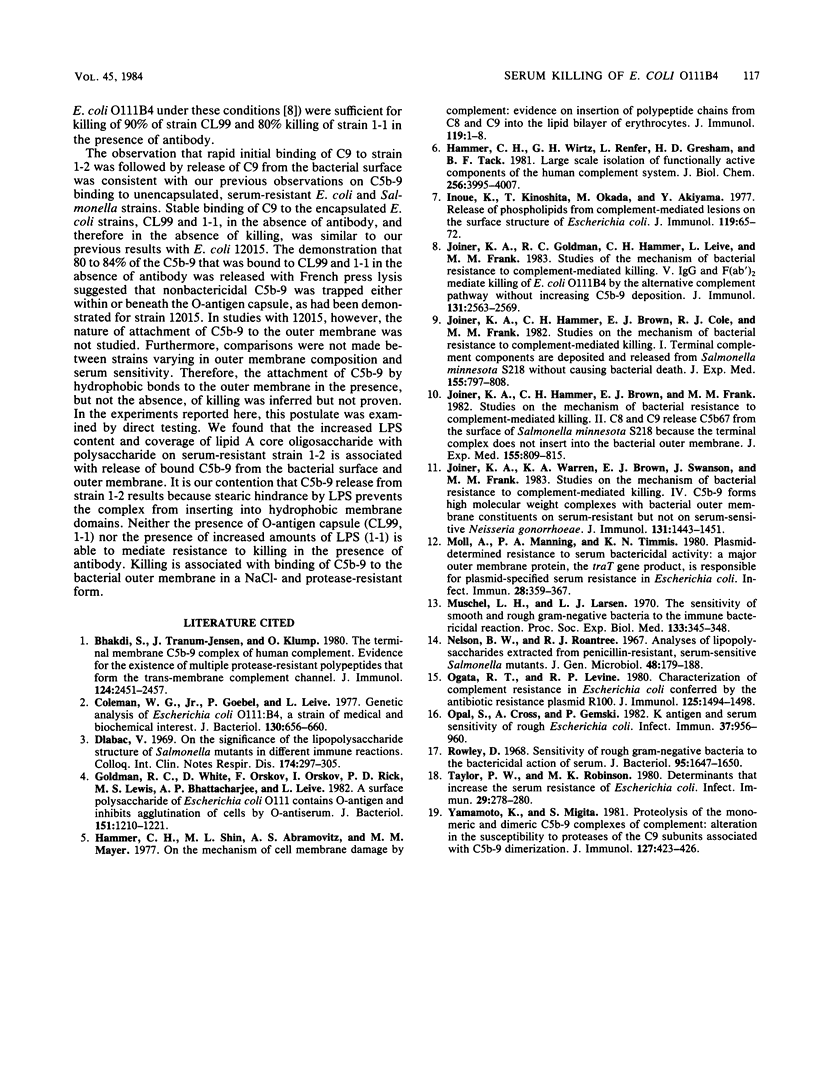
Selected References
These references are in PubMed. This may not be the complete list of references from this article.
- Bhakdi S., Tranum-Jensen J., Klump O. The terminal membrane C5b-9 complex of human complement. Evidence for the existence of multiple protease-resistant polypeptides that form the trans-membrane complement channel. J Immunol. 1980 May;124(5):2451–2457. [PubMed] [Google Scholar]
- Coleman W. G., Jr, Goebel P. J., Leive L. Genetic analysis of Escherichia coli O111:B4, a strain of medical and biochemical interest. J Bacteriol. 1977 May;130(2):656–660. doi: 10.1128/jb.130.2.656-660.1977. [DOI] [PMC free article] [PubMed] [Google Scholar]
- Goldman R. C., White D., Orskov F., Orskov I., Rick P. D., Lewis M. S., Bhattacharjee A. K., Leive L. A surface polysaccharide of Escherichia coli O111 contains O-antigen and inhibits agglutination of cells by O-antiserum. J Bacteriol. 1982 Sep;151(3):1210–1221. doi: 10.1128/jb.151.3.1210-1221.1982. [DOI] [PMC free article] [PubMed] [Google Scholar]
- Hammer C. H., Shin M. L., Abramovitz A. S., Mayer M. M. On the mechanism of cell membrane damage by complement: evidence on insertion of polypeptide chains from C8 and C9 into the lipid bilayer of erythrocytes. J Immunol. 1977 Jul;119(1):1–8. [PubMed] [Google Scholar]
- Hammer C. H., Wirtz G. H., Renfer L., Gresham H. D., Tack B. F. Large scale isolation of functionally active components of the human complement system. J Biol Chem. 1981 Apr 25;256(8):3995–4006. [PubMed] [Google Scholar]
- Inoue K., Kinoshita T., Okada M., Akiyama Y. Release of phospholipids from complement-mediated lesions on the surface structure of Escherichia coli. J Immunol. 1977 Jul;119(1):65–72. [PubMed] [Google Scholar]
- Joiner K. A., Goldman R. C., Hammer C. H., Leive L., Frank M. M. Studies of the mechanism of bacterial resistance to complement-mediated killing. V. IgG and F(ab')2 mediate killing of E. coli 0111B4 by the alternative complement pathway without increasing C5b-9 deposition. J Immunol. 1983 Nov;131(5):2563–2569. [PubMed] [Google Scholar]
- Joiner K. A., Hammer C. H., Brown E. J., Cole R. J., Frank M. M. Studies on the mechanism of bacterial resistance to complement-mediated killing. I. Terminal complement components are deposited and released from Salmonella minnesota S218 without causing bacterial death. J Exp Med. 1982 Mar 1;155(3):797–808. doi: 10.1084/jem.155.3.797. [DOI] [PMC free article] [PubMed] [Google Scholar]
- Joiner K. A., Hammer C. H., Brown E. J., Frank M. M. Studies on the mechanism of bacterial resistance to complement-mediated killing. II. C8 and C9 release C5b67 from the surface of Salmonella minnesota S218 because the terminal complex does not insert into the bacterial outer membrane. J Exp Med. 1982 Mar 1;155(3):809–819. doi: 10.1084/jem.155.3.809. [DOI] [PMC free article] [PubMed] [Google Scholar]
- Joiner K. A., Warren K. A., Brown E. J., Swanson J., Frank M. M. Studies on the mechanism of bacterial resistance to complement-mediated killing. IV. C5b-9 forms high molecular weight complexes with bacterial outer membrane constituents on serum-resistant but not on serum-sensitive Neisseria gonorrhoeae. J Immunol. 1983 Sep;131(3):1443–1451. [PubMed] [Google Scholar]
- Moll A., Manning P. A., Timmis K. N. Plasmid-determined resistance to serum bactericidal activity: a major outer membrane protein, the traT gene product, is responsible for plasmid-specified serum resistance in Escherichia coli. Infect Immun. 1980 May;28(2):359–367. doi: 10.1128/iai.28.2.359-367.1980. [DOI] [PMC free article] [PubMed] [Google Scholar]
- Muschel L. H., Larsen L. J. The sensitivity of smooth and rough gram-negative bacteria to the immune bactericidal reaction. Proc Soc Exp Biol Med. 1970 Jan;133(1):345–348. doi: 10.3181/00379727-133-34472. [DOI] [PubMed] [Google Scholar]
- Nelson B. W., Roantree R. J. Analyses of lipopolysaccharides extracted from penicillin-resistant, serum-sensitive salmonella mutants. J Gen Microbiol. 1967 Aug;48(2):179–188. doi: 10.1099/00221287-48-2-179. [DOI] [PubMed] [Google Scholar]
- Ogata R. T., Levine R. P. Characterization of complement resistance in Escherichia coli conferred by the antibiotic resistance plasmid R100. J Immunol. 1980 Oct;125(4):1494–1498. [PubMed] [Google Scholar]
- Opal S., Cross A., Gemski P. K antigen and serum sensitivity of rough Escherichia coli. Infect Immun. 1982 Sep;37(3):956–960. doi: 10.1128/iai.37.3.956-960.1982. [DOI] [PMC free article] [PubMed] [Google Scholar]
- Rowley D. Sensitivity of rough gram-negative bacteria to the bactericidal action of serum. J Bacteriol. 1968 May;95(5):1647–1650. doi: 10.1128/jb.95.5.1647-1650.1968. [DOI] [PMC free article] [PubMed] [Google Scholar]
- Taylor P. W., Robinson M. K. Determinants that increase the serum resistance of Escherichia coli. Infect Immun. 1980 Jul;29(1):278–280. doi: 10.1128/iai.29.1.278-280.1980. [DOI] [PMC free article] [PubMed] [Google Scholar]
- Yamamoto K., Migita S. Proteolysis of the monomeric and dimeric C5b-9 complexes of complement: alteration in the susceptibility to proteases of the C9 subunits associated with C5b-9 dimerization. J Immunol. 1981 Aug;127(2):423–426. [PubMed] [Google Scholar]