Abstract
Ubiquitination plays a major role in regulating cell surface and intracellular localization of major histocompatibility complex class II molecules. Two E3 ligases, MARCH I and MARCH VIII, have been shown to polyubiquitinate lysine residue 225 in the cytoplasmic tail of I-Aβ and HLA-DRβ. We show that lysine residue 219 in the cytoplasmic tail of DRα is also subject to polyubiquitination. Each chain of the HLA-DR heterodimer is independently recognized and ubiquitinated, but DRβ is more extensively modified. In the cytoplasmic tail of DRβ lysine, residue 225 is the only residue that is absolutely required for ubiquitination; all other residues can be deleted or substituted without loss of function. In contrast, although lysine 219 is absolutely required for modification of DRα, other features of the DRα tail act to limit the extent of ubiquitination.
Major histocompatibility complex (MHC)3 class II molecules play an essential role in adaptive immune responses through the presentation of antigenic peptides to CD4-restricted T helper cells. MHC class II molecules are subject to complex post-translational control. During biosynthesis, the class II α and β chains assemble in the endoplasmic reticulum (ER) with the chaperone protein invariant chain (Ii). This prevents association with ER-derived peptides, facilitates folding of the class II molecule, and targets the complex to endocytic compartments, called MHC class II-containing compartments (1). Within the endocytic pathway, Ii is sequentially cleaved, leaving a short peptide fragment, CLIP, in the peptide binding groove (2). With guidance from the class II-related chaperone HLA-DM, CLIP is removed, and the class II molecules are loaded with an array of peptides derived from proteins that have accessed the endocytic pathway (3). There is considerable debate concerning the route of trafficking of class II to peptide loading compartments. Both direct targeting from the trans-Golgi network and indirect targeting from the cell surface have been proposed (4, 5). The precise nature of the peptide-loading compartment has been subject to extensive investigation. Both early and late endocytic compartments have been proposed as sites of class II peptide acquisition. However, it is generally concluded that the peptide loading compartment should contain the chaperone HLA-DM, MHC class II that is devoid of intact Ii, antigenic peptide, and appropriate proteases (6). Detailed localization of class II molecules within MHC class II-containing compartment-loading compartments has also been investigated, and although class II and DM are present on both limiting and internal membranes, their interaction and hence peptide loading is restricted to the internal membranes (7, 8). Once loaded with antigenic peptide, class II must access the cell surface for presentation to T cells, and again the mechanisms governing this are poorly understood.
MHC class II expression is a defining feature of professional antigen-presenting cells, and post-translational targeting is under stringent control, particularly in dendritic cells. When pattern recognition receptors on antigen-presenting cells encounter ligands such as lipopolysaccharide, subsequent signaling results in redistribution of the intracellular pool of class II to the cell surface. This is controlled through regulated ubiquitination of the class II β chain (9–11). In immature dendritic cells, class II is subject to constitutive ubiquitination by MARCH I (12), leading to reduced cell surface expression and predominant intracellular accumulation. Upon maturation, ubiquitination is reduced, and class II molecules accumulate at the cell surface. In B cells, MARCH I is also implicated in regulating surface class II expression, again through ubiquitination of the β chain. This suggests that ubiquitination may be a general mechanism for post-translational regulation of class II antigen presentation (13). Ubiquitination is a reversible post-translational modification that has varied consequences for the tagged protein. Diverse modifications are possible: single ubiquitin (Ub) molecules may be attached to a single lysine residue, monoubiquitination; or to multiple lysine residues within the same protein, multiubiquitination (14). Additionally, ubiquitin itself contains seven internal lysine residues that may provide substrates for further Ub attachment, generating functionally distinct polyubiquitin chains (14).
We investigated ubiquitination of the MHC class II molecule HLA-DR and showed that both chains of the heterodimer are modified by polyubiquitination. MARCH I and the related E3 ligase MARCH VIII (c-MIR), preferentially target lysine 225 in the DRβ chain but also ubiquitinate lysine 219 in the DRα tail. The transmembrane and cytoplasmic domains from each chain are sufficient for independent modification by the two MARCH proteins, but the relative levels of ubiquitination are determined by the cytoplasmic tails.
EXPERIMENTAL PROCEDURES
Cell Culture and Transfection—Mel JuSo, 293T, and HeLa cells were maintained in Dulbecco's modified Eagle's medium, 10% fetal calf serum, 10 mm sodium pyruvate, 10 mm nonessential amino acids, 2 mm glutamine, 100 units penicillin, and 100 units of streptomycin. Transfections were performed using Effectene (Qiagen), according to the manufacturer's recommendations. For stable transfectants, cells were incubated in the presence of the appropriate antibiotic (1 mg/ml Geneticin, 100 μg/ml Zeocin), and positive cells were sorted for either HLA-DR (L243) or CD8 (OKT8) using a MoFlo flow cytometer (Cytomation, Fort Collins, CO).
Flow Cytometry—Adherent cells were washed with PBS, harvested using Cell Dissociation Buffer (Sigma), and incubated for 30 min on ice, with anti-CD8 (OKT8), anti-HA (Miltenyi Biotec), anti-HLA-DR (L243), or anti-Ii (Serotec) antibodies, in FACS buffer (5% fetal calf serum, 2 mm EDTA in PBS). After washing, cells were incubated with RPE anti-mouse (Dako Ltd.), fixed in 3% formaldehyde and analyzed using a FACScan™ flow cytometer and Summit software (BD Biosciences).
Immunoprecipitation and Western Blot—Anti-HLA-DR (L243) antibody was directly conjugated to cyanogen bromide-activated-Sepharose 4B (Sigma), and anti-DRα antibody TAL1B5 was conjugated to horseradish peroxidase (Sigma), according to the manufacturer's instructions. Cells were harvested using Cell Dissociation Buffer (Sigma) and lysed in lysis buffer (PBS, 1% Nonidet P-40, 50 mm Tris (pH 7.5), 5 mm EDTA, 150 mm NaCl, protease inhibitor mixture (Roche Applied Science), and 5 mm iodoacetamide) for 30 min at 4 °C. Immunoprecipitations were performed with Sepharose-coupled anti-HLA-DR (L243) or CD8 (OKT8) preincubated with protein G-Sepharose 4B (Sigma). Lysates were washed extensively and incubated for 5 min at 95 °C in SDS-PAGE loading buffer prior to standard SDS-PAGE and transfer to Hybond™ ECL™ membrane (Amersham Biosciences). Membranes were blocked in PBS, 5% skim milk, 0.1% Tween 20 (Sigma) overnight at 4 °C before probing for MHC class II (HRP-TAL1B5) or ubiquitin (HRP-P4D1; Santa Cruz Biotechnology, Inc., Santa Cruz, CA). Detection was performed using ECL Plus™ Western blotting detection reagents (ECL plus; Amersham Biosciences).
Plasmid Constructs—All PCR was performed with KOD HiFi DNA polymerase according to the manufacturer's instructions (Calbiochem). Primers used for amplification are summarized in Table 1. The HA-tagged HA-DRB and HA-DRA constructs were generated by PCR amplification from parental DRBw53 (DRB4) and DRA*0101 constructs and cloned into HA-PMX-puro (a gift from Dr. Chiwen Chang, Cambridge, UK). Untagged DR3β chain constructs were amplified from a parental DR1*0303 sequence and cloned into pcDNA3.1/Neo. Untagged DRA constructs were cloned into pcDNA3.1/Zeo. The CD8-DRA and CD8-DRB reporter constructs were generated by overlap PCR amplification and cloned into pcDNA3.1/Neo. Untagged MARCH I was generated by PCR amplification from MARCH I-EGFPc1 and cloned into pcDNA3.1/Neo. All PCR-generated constructs were subject to DNA sequence analysis, to verify authenticity. MARCH I-EGFPc1 and MARCH VIII-EGFPc1 were gifts from Professor Paul Lehner (Cambridge, UK). Additional human MARCH VIII constructs named c-MIRwt and its catalytically inactive variant (c-MIRmt), bearing Cys-Ser mutations in the zinc binding domain, were a gift from Professor Satoshi Ishido (Yokohama, Japan).
TABLE 1.
Summary of primers used for PCR Primers used in construct generation are listed as sense and antisense sequences. Chimeric CD8-DRAB and -DRBA constructs were generated by overlap PCR in a single reaction using standard concentrations of 5′ and 3′ primers and one-tenth the concentration of the internal primers (int).
Confocal Microscopy—HeLa cells were grown on coverslips, and 24 h after transfection, they were fixed for 10 min with 4% paraformaldehyde. Fixed cells were washed in PBS, permeabilized with 0.1% saponin in PBS, and incubated in PBS containing 0.1% saponin and 5% horse serum with primary antibody: anti-HLA-DR (L243, IgG2a), anti-Ii (anti-CD74; Pharmingen (IgG2a), Serotec (IgG1)), anti-EEA1 (Transduction laboratories (IgG1)), or rabbit polyclonal anti-Lamp-1 (Abcam). Coverslips were washed in PBS containing 0.1% saponin and incubated with appropriate Alexa-conjugated secondary antibodies (Molecular Probes). Coverslips, mounted in Mowiol (Sigma), were viewed under a Zeiss LSM 510 laser-scanning confocal microscope. Images were analyzed using the LSM (Zeiss) and Adobe Photoshop software.
RESULTS
Substitution of Lysine 225 in DRβ Does Not Prevent MARCH-induced Down-regulation of HLA-DR—To investigate ubiquitination of MHC class II molecules, we generated HA-tagged DRα and DRβ chain constructs and stably expressed them in Mel JuSo cells. Fig. 1 summarizes the COOH-terminal amino acid composition of the constructs used in this study. Lines expressing similar levels of the tagged molecules were transiently transfected with cDNA vectors encoding the E3 ligases MARCH I, MARCH VIII, c-MIRwt, and c-MIRmt. Expression of HLA-DR was then examined by FACS. As shown in Fig. 2, expression of the active E3 ligases resulted in substantial down-regulation of wild-type HLA-DR, whereas expression of the catalytically inactive c-MIRmt had no effect. Interestingly, cells expressing HA-DRB-K225R showed substantial loss of surface class II, implying that residues in addition to DRB-Lys225 were subject to ubiquitination. This was unexpected, given that previous studies found ubiquitination of MHC class II to be entirely dependent upon a single lysine residue present in the cytoplasmic tail of the β chain (12). Down-regulation was not influenced by the DRβ-encoded COOH-terminal dileucine endocytosis signal or residues carboxyl-terminal of Lys225, since levels of HA-DRB-L235A,L236A and HA-DRB-Δ233 were indistinguishable from those of HA-DRB-K225R. Several explanations could account for our observations. If HLA-DR formed dimers or multimers, association with native DR could enable indirect down-regulation of the HA-tagged molecule. Alternatively, residues in the cytoplasmic tail of DRα could be ubiquitinated, possible targets being two lysine residues located at positions 215 and 219.
FIGURE 1.
Summary of the amino acid composition of constructs used in this study. The numbering of residues is taken from the mature protein after signal sequence removal. Single-letter amino codes are used. Sequences derived from CD8 are in italic type, and substituted residues are in boldface type.
FIGURE 2.
MARCH-induced down-regulation of HA-DRB-K225R. Mel JuSo cells, stably expressing various HA-tagged DRα and DRβ constructs, were transiently transfected with MARCH I, MARCH VIII, c-MIRwt, and c-MIRmt, and surface HA expression was assessed by FACS. Dot plots show GFP (FL1) on the x axis and PE-HA (FL2) on the y axis. The R3 gate, which represents cells expressing the E3 ligase, as assessed by GFP expression, was set against IgG control at 0.1%. The IgG control was set using cells expressing GFP (R3), to enable direct comparison between with E3 ligase-expressing transfectants. Data are representative of at least three independent experiments. A, expression of MARCH I, MARCH VIII, and c-MIRwt induced down-regulation of surface HA-DRB, HA-DRB-K225R, HA-DRB-L235A,L236A, and HA-DRB-Δ225. No reduction in surface expression was observed in cells transfected with c-MIRmt. When compared with IgG control antibody staining, MARCH-induced down-regulation of HA-DRB-K225R and HA-DRB-Δ225 was substantial, but less than observed for HA-DRB and HA-DRB-L235A,L236A. B, MARCH I, MARCH VIII, and c-MIRwt induced down-regulation of surface HA-DRA and DRA-K219R. No reduction in surface expression was observed in cells transfected with c-MIRmt. Data are representative of at least three experiments.
Both HLA-DRα and HLA-DRβ Are Subject to Polyubiquitination—To explore down-regulation of DRB-K225R, we performed transient transfections in cells lacking endogenous MHC class II. As shown in Fig. 3, similar levels of surface HLA-DR expression were observed for all combinations of wild-type and mutated DRα and DRβ expressed in 293T cells. Co-expression of MARCH I, or c-MIR, induced down-regulation of all combinations, except for the pair comprising DRA-K219R/DRB-K225R, which remained unaffected. This is most clearly seen by comparison of c-MIRwt and its catalytically inactive counterpart, c-MIRmt. As expected, MARCH VIII behaved the same as c-MIRwt (data not shown). These results show that the down-regulation induced by MARCH I/c-MIR, previously seen with the tagged HA-DRB-K225R construct, was dependent upon lysine 219 of the DRα chain. We noted that the degree of surface down-regulation was greater in the presence of the wild-type β chain and was less when DRA/DRB-K225R were co-expressed, suggesting that ubiquitination of DRα was less efficient than ubiquitination of DRβ. Immunoprecipitation and Western blot analysis of lysates from these transfected cells were used to demonstrate ubiquitination directly. As shown in Fig. 3B, a ladder of bands in the 36–70 kDa size range was observed in lysates from cells transfected with DRA/DRB (lanes 1 and 5). A similar ladder was associated with cells transfected with DRA-K219R/DRB (lanes 3 and 7), suggesting that ubiquitination was predominantly associated with the β chain of the DR dimer. In cells transfected with DRA/DRB-K225R, a weaker ladder of ubiquitinated products was observed, which partially overlapped with that described previously (lanes 2 and 6). Importantly, no ubiquitinated products were observed in cells expressing DRA-K219R/DRB-K225R (lanes 4 and 8). Therefore, down-regulation of surface class II correlated with polyubiquitination of both DRα and DRβ by MARCH I (lanes 1–4) and MARCH VIII (lanes 5–8). These results also show that the additional lysine at position 215 of DRα is not subject to ubiquitination. We were unable to explain previous studies that failed to detect Ub of DRα and questioned if this could be due to the presence of a valine to leucine polymorphism at position 217 in the DRα cytoplasmic tail. However, both alleles of DRα (DRA*0101(valine) and DRA*0102(leucine)) were equally susceptible to MARCH-induced down-regulation (data not shown). We were unable to directly visualize ubiquitinated DRα with the DRα-specific antibody TAL1B5, although unmodified DRα was readily visualized (Fig. 3B, lower panels). This antibody recognizes the cytoplasmic tail of DRα, and although binding does not appear to be influenced by the K219R substitution (Fig. 3B), it is possible that steric hindrance, due to covalent attachment of Ub, could influence the detection of modified products. Alternatively, the proportion of DRα modified may be below the level of detection. The latter explanation is unlikely, since, even after extensive overexposure, there was no hint of a ubiquitinated product. The conclusion of these studies is that lysine residues in both DRα and DRβ are polyubiquitinated by both MARCH I and MARCH VIII but that DRβ is the predominant substrate. We found no evidence of ubiquitination of nonlysine residues on either the α or β chain.
FIGURE 3.
Lysine residues Lys219 and Lys225 on HLA-DRα and HLA-DRβ, respectively, are subject to polyubiquitination. A, 293T cells were transiently co-transfected with wild-type or mutated forms of HLA-DRα and HLA-DRβ, together with either MARCH I, c-MIRwt, or c-MIRmt. The levels of surface HLA-DR were assessed by FACS using PE-L243 in the FL2 channel. E3 ligase expression was monitored indirectly by measuring GFP expression in the FL1 channel. All combinations of DRα and DRβ resulted in MARCH I- and c-MIRwt (MARCH VIII)-induced DR down-regulation, except for DRA-K219R/DRB-K225R. No change in surface HLA-DR expression was seen in the presence of c-MIRmt. Data are representative of at least three independent experiments. B, HLA-DR was immunoprecipitated (IP) from lysates of the transfected cells depicted above using L243 monoclonal antibody directly conjugated to Sepharose. After standard SDS-PAGE separation and Western transfer (WB), DRα was detected with HRP-TAL1B5, and ubiquitinated HLA-DR was detected with the anti-ubiquitin antibody HRP-P4D1. Lanes 1–4 were transfected with MARCH I; lanes 5–8 were transfected with MARCH VIII. Lanes 1 and 5 expressed DRA and DRB; lanes 2 and 6 expressed DRA and DRB-K225R; lanes 3 and 7 expressed DRA-K219R and DRB; and lanes 4 and 8 expressed DRA-K219R and DRB-K225R. The upper panels show ubiquitinated HLA-DR, as detected with HRP-P4D1, the lower panels show DRα as detected by HRP-TAL1B5. No additional bands suggestive of ubiquitinated DRα were evident in the lower panels, even after long exposure. The upper panel shows that both DRα and DRβ are subject to polyubiquitination. The signal for DRβ is stronger than for DRα. Data are representative of at least three independent experiments.
Polyubiquitination of DRα and DRβ Is Controlled by Their Transmembrane and Cytoplasmic Tail Domains—To investigate which regions of the HLA-DR molecule were important for recognition by the MARCH proteins, we used CD8 as a reporter molecule (15). As shown in Fig. 4, constructs containing the transmembrane and cytoplasmic tails of DRα or DRβ (CD8-DRA and CD8-DRB) showed reduced surface expression in the presence of either E3 ligase, whereas control cells transfected with c-MIRmt (inactive MARCH VIII) were unaffected. Substitution of lysines for arginine confirmed that down-regulation was dependent upon lysines 219 and 225 of the α and β chains, respectively. We repeatedly observed that down-regulation was more pronounced for CD8-DRB compared with CD8-DRA, again implying that the β chain was more efficiently ubiquitinated. This was not due to the presence of the DRβ-encoded dileucine endocytosis signal, since CD8-DRB-L235A,L236A behaved like the wild-type. To explore the differential ubiquitination of DRα and DRβ, we first extended the CD8-DRA construct to incorporate five residues from the stalk region of DRα (CD8-DRAextra), since the corresponding region was present in CD8-DRB (see Fig. 1). Previous studies, using murine and human MHC class I chimeras, suggest that the juxtamembrane region of class I is critical for recognition by MIR2, a herpesvirus-encoded E3 ligase that is related to MARCH I and MARCH VIII (16). MARCH-induced down-regulation of CD8-DRAextra was slightly more than observed for CD8-DRA but did not reach the levels observed for CD8-DRB (data not shown). The juxtamembrane region of DRα is therefore not required for recognition by MARCH I or MARCH VIII, unlike the viral homologue, MIR2.
FIGURE 4.
Transmembrane and cytoplasmic domains from both DRα and DRβ are independently targeted by MARCH I and MARCH VIII. CD8 reporter constructs comprising the CD8 extracellular domain and DRα or DRβ transmembrane and cytoplasmic tails were stably expressed in Mel JuSo cells (A) or transiently expressed in 293T cells (B). Dot plots show GFP (FL1) on the x axis and anti-CD8 (PE-OKT8) binding (FL2) on the y axis. The R3 gate, which represents cells expressing the E3 ligase, as assessed by GFP expression, was set against IgG control at 0.1%. The IgG control was set using cells expressing GFP (R3), to enable direct comparison between E3 ligase-expressing transfectants. Data are representative of at least three independent experiments. A, a CD8 chimera containing the transmembrane and cytoplasmic tail of DRβ is subject to MARCH I- and MARCH VIII-induced down-regulation. This was abolished by substitution of lysine residue Lys225 for arginine (CD8-DRB-K225R). The dileucine motif at positions 235 and 236 was not required for MARCH-induced down-regulation, since the behavior of CD8-DRB-L235A,L236A was indistinguishable from that of CD8-DRB. B, CD8-DRA was also targeted by MARCH I and MARCH VIII, and this was dependent upon a lysine residue, Lys219, in its cytoplasmic tail. The extent of surface down-regulation of CD8-DRA was less than for CD8-DRB. Data are representative of at least three independent experiments.
The Extent of Surface Down-regulation Correlates with the Presence of the DRα and DRβ Cytoplasmic Tails—Domain swaps, where the cytoplasmic tail of DRβ was placed carboxylterminal of the DRα transmembrane region (CD8-DRAB (see Fig. 1)), resulted in more efficient down-regulation by MARCH VIII (Fig. 5A). In a reciprocal exchange, CD8-DRBA behaved like CD8-DRA. Ubiquitination of these reporter molecules was readily visualized after immunoprecipitation and Western blot, and all constructs showed a ladder of bands in the 30–60 kDa range, consistent with polyubiquitination (Fig. 5B). In agreement with their different degree of down-regulation from the cell surface, constructs containing the cytoplasmic tail of DRβ (CD8-DRB and CD8-DRAB) were more extensively ubiquitinated than those bearing the corresponding region of DRα (CD8-DRA and CD8-DRBA). These results show that both DRα and DRβ can be independently recognized by the MARCH I and MARCH VIII E3 ligases and demonstrate that the extent of ubiquitination correlates with the presence of the DRα or DRβ cytoplasmic tails. An explanation of these results is that the extensive ubiquitination of DRβ is due to features associated with the cytoplasmic tail of DRβ that enhance ubiquitination. Alternatively, features associated with the tail of DRα could act to limit its ubiquitination.
FIGURE 5.
Efficient ubiquitination correlates with the presence of the DRβ cytoplasmic tail. To determine which regions of DRα and DRβ were important for ubiquitination, 293T cells were transfected with CD8-DRA, CD8-DRB, CD8-DRAB, and CD8-DRBA, together with c-MIRwt or c-MIRmt. CD8-DRAB was generated by replacing the cytoplasmic tail of CD8-DRA with that from DRβ. CD8-DRBA is the reciprocal exchange, involving CD8-DRB and DRA. Data are representative of at least three independent experiments. A, FACS analysis of the 293T cell transfectants, described above, stained with the anti-CD8 antibody, PE-OKT8. Similar levels of surface CD8 expression and control IgG staining were seen in all four transfectants. Greater c-MIR-induced down-regulation was seen in CD8-DRB and CD8-DRAB transfectants compared with CD8-DRA and CD8-DRBA. No down-regulation was seen in the presence of c-MIRmt; in fact, surface CD8 appears to increase. B, CD8 chimeras were immunoprecipitated from lysates of the transfected cells depicted above using OKT8 and protein A-Sepharose. After standard SDS-PAGE separation and Western transfer, the presence of the ubiquitinated CD8-chimeras was detected with the anti-ubiquitin antibody HRP-P4D1. Lane 1, CD8-DRA; lane 2, CD8-DRB; lane 3, CD8-DRAB; lane 4, CD8-DRBA. All molecules were subject to polyubiquitination, and the strength of signal was highest for CD8-DRB and CD8-DRAB and lowest for CD8-DRA and CD8-DRBA.
A Minimal DRβ Cytoplasmic Tail, 222RAAK225, Is a More Efficient Substrate for MARCH I- and MARCH VIII-induced Ubiquitination than the DRα Tail—We investigated the minimal requirements for ubiquitination of DRβ by sequential truncation and alanine substitution of CD8-DRB. Truncation of the entire tail, up to but not including lysine 225 (CD8-DRBΔ226), had no discernable effect on the extent of down-regulation compared with the wild-type (Fig. 6A). Additionally, residues adjacent to lysine 225 (CD8-DRB-222AAAKAAAA229, CD8-DRB-222RAAK225) could be substituted for alanine without loss of regulation by MARCH I or MARCH VIII. Therefore, lysine 225 appeared to be the key single residue in the cytoplasmic tail of DRβ. Other residues could be either removed or substituted with negligible effect (Fig. 6A). The lysine residue could also be substituted for cysteine and still function as a substrate for MARCH I and MARCH VIII (Fig. 6; CD8-DRB-K225C).
FIGURE 6.
A minimal CD8-DRB cytoplasmic tail, RAAK, is an efficient target for ubiquitination. 293T cells were transfected with CD8-DRB or CD8-DRA reporter molecules containing deletions and substitutions in the DRβ cytoplasmic tail, coupled with either c-MIRwt or c-MIRmt. After 24 h, cells were subjected to FACS analysis with PE-OKT8. Dot plots show c-MIR expression on the x axis (FL1), as monitored by GFP expression and CD8 expression on the y axis (FL2). Histograms showing CD8 expression are presented. Data are representative of at least three independent experiments. A, all CD8-DRB constructs were efficiently down-regulated by c-MIR, including a “minimal” cytoplasmic tail CD8-DRB-222RAAK225. In addition, substitution of lysine 225 for cysteine resulted in significant, if reduced, down-regulation. B, CD8-DRA-215AAAAKAAA222 showed enhanced down-regulation in the presence of c-MIRwt compared with CD8-DRA, suggesting that elements in the tail of DRα have an antagonistic effect upon ubiquitination of DRα. 293T cells were transfected with CD8-DRA-215AAAAKAAA222 or CD8-DRA, together with either c-MIRwt or c-MIRmt and cell surface CD8 determined by FACS. The plot shows mean fluorescence values for cell surface CD8-DRA-215AAAAKAAA222 and CD8-DRA in the presence of c-MIRwt, expressed as a percentage of the expression observed in the presence of c-MIRmt. In each of three independent experiments, there is less CD8-DRA-215AAAAKAAA222 at the plasma membrane compared with CD8-DRA. CD8-DRA-215AAAAKAAA222 is therefore more efficiently removed from the cell surface compared with CD8-DRA.
In contrast to the above, substitutions in the cytoplasmic tail of DRα actually enhanced MARCH-induced surface down-regulation. Fig. 6B compares down-regulation of CD8-DRA and CD8-DRA-215AAAAKAAA222 in the presence of c-MIRwt. Fig. 6B displays FACS profile data as the percentage of the molecules remaining at the cell surface in the presence of c-MIRwt compared with c-MIRmt. In all three independent experiments, the percentage of CD8-DRA-215AAAAKAAA222 remaining at the cell surface was less than for CD8-DRA (Fig. 6B).
In summary, a minimal DRβ cytoplasmic tail (222RAAK225) afforded greater down-regulation than the normal DRα tail, 215KGLRKSNAAERRGPL229. Substitution of residues surrounding the ubiquitinated lysine in DRα enhanced its down-regulation. This suggests that sequences in the DRα tail act to limit the extent of down-regulation and, by implication, ubiquitination.
Intracellular HLA-DR Co-localizes with MARCH I in Late Endocytic Compartments—To investigate if localization of MARCH proteins and class II correlated with ubiquitination, intracellular distribution was investigated by confocal microscopy. As shown in Fig. 7 (A–D), MARCH I localized predominantly to EEA1-positive early endosomes and Lamp1-positive late endosomal compartments and was also present at the cell surface, as has previously been reported (12). Intracellular class II was not associated with early endosomes (Fig. 7, A and B) but localized to Lamp1-positive late endosomal compartments, some of which were also positive for MARCH I (Fig. 7, C and D). This distribution of class II was not influenced by lysine to arginine substitutions in the various DR constructs (Fig. 7, compare A and C with B and D). All construct combinations showed a comparable intracellular distribution. Similar results were also obtained for localization of class II and MARCH VIII (data not shown). The localization of class II and MARCH I, in Lamp1-positive compartments, did not correlate with the extent of ubiquitination, since both DRA/DRB and DRA-K219R/DRB-K225R showed a similar degree of colocalization with the MARCH proteins, but the latter is not ubiquitinated.
FIGURE 7.
Intracellular distribution of MHC class II and MARCH I. HeLa cells were transiently transfected with wild-type and mutated DRα and DRβ constructs, together with MARCH I, in the presence or absence of Ii. Intracellular distribution was analyzed by confocal microscopy. A and B show colocalization of MARCH I and EEA1 (arrows), in cells transfected with either DRA/DRB (A) or DRA-K219R/DRB-K225R (B). The merged image shows DR in blue, EEA1 in red, and MARCH I in green; co-localized MARCH and EEA1 is yellow. C and D show colocalization of MARCH I and Lamp-1 (arrows), in cells transfected with either DRA/DRB (C) or DRA-K219R/DRB-K225R (D). The merged images show DR in blue, Lamp-1 in red, and MARCH Iin green. Note that the majority of class II is present in Lamp-1-positive compartments (purple), some of which co-localize with MARCH I. E–G show localization of class II in the presence of Ii. The merged image shows DR or EEA1 in blue, Ii in red, and MARCH I in green. E and F show colocalization of Ii and MARCH I (arrows) in cells transfected with DRA/DRB (E) or DRA-K219R/DRB-K225R (F). In both cases, Ii shows good colocalization with MARCH I, whereas DR is mainly in MARCH I-negative vesicles. G shows a high degree of colocalization between Ii and EEA1. Together, this shows that Ii is in MARCH I-positive, EEA1-positive early endosomes. Bar, 10 μm.
We also investigated how the distribution of class II was influenced by the presence of Ii. As shown in Fig. 7, G panels, Ii localized to EEA1-positive early endosomes, some of which co-localized with MARCH I. Ii was not present in Lamp1-positive late endosomes (data not shown). Mature DRA/DRB and DRA-K219R/DRB-K225R was not present in EEA1-positive early endosomes (Fig. 7, E and F panels). The majority of mature class II did not colocalize with Ii. This is consistent with a need for Ii chain removal for binding of L243, a process that occurs upon trafficking to late endosomal compartments.
From this we conclude that, in the presence or absence of Ii, the majority of intracellular class II is in Lamp1-positive late endosomal compartments. This distribution appears independent of ubiquitination, since we observe a similar localization for all DR constructs irrespective of their ability to be ubiquitinated.
Ubiquitination of Class II Occurs in Both the Presence and Absence of Ii—To investigate if association with Ii influenced the pattern of ubiquitination of class II, we performed transient transfections in the presence of Ii. As shown in Fig. 8A, co-transfection of DRA/DRB and Ii resulted in expression of both Ii- and L243-reactive HLA-DR at the cell surface, as expected. The presence of MARCH I or MARCH VIII led to a large reduction in surface expression of wild-type DR (Fig. 8B). Cells transfected with Ii/DRA-K219R/DRB showed a similar reduction. Cells transfected with Ii/DRA/DRB-K225R showed a less marked reduction in surface expression, as we have previously observed in the absence of Ii. In cells transfected with Ii/DRA-K219R/DRB-K225R, surface class II remained high in the presence of either MARCH I or MARCH VIII. We investigated ubiquitination of class II in these transfectants and observed that the pattern of polyubiquitinated products was similar to that observed in the absence of Ii (Figs. 3B and 8C). Therefore, although the overall level of class II increased in the presence of Ii, consistent with its role as a class II chaperone, the pattern of ubiquitination remained unchanged.
FIGURE 8.
Ubiquitination of MHC class II in the presence and absence of Ii. To determine if ubiquitination was influenced by the presence of Ii, 293T cells were transiently co-transfected with Ii and wild-type or mutated forms of HLA-DRα and HLA-DRβ, together with either MARCH I or MARCH VIII. A, FACS analysis of 293T cells transfected with DRA/DRB and Ii demonstrates that the majority of transfected cells express both class II and Ii. B, 293T cells were transiently co-transfected with Ii and wild-type or mutated forms of DRα and DRβ, together with either MARCH I or MARCH VIII. FACS profiles of surface L243-reactive class II expression demonstrate that all combinations of DRα and DRβ are down-regulated by MARCH I and MARCH VIII, except for DRA-K219R/DRB-K225R, as previously seen in the absence of Ii (Fig. 3). C, HLA-DR was immunoprecipitated (IP) from lysates of the transfected cells depicted above, using L243 monoclonal antibody directly conjugated to Sepharose. After standard SDS-PAGE separation and Western transfer (WB), DRα was detected with HRP-TAL1B5 (bottom), and ubiquitinated HLA-DR was detected with the anti-ubiquitin antibody HRP-P4D1 (top). Lanes 1–4 were transfected with MARCH I, and lanes 5–8 were transfected with MARCH VIII. Lanes 1 and 5 expressed DRA and DRB; lanes 2 and 6 expressed DRA and DRB-K225R; lanes 3 and 7 expressed DRA-K219R and DRB; and lanes 4 and 8 expressed DRA-K219R and DRB-K225R. Comparison with Fig. 3C shows that the pattern of ubiquitination of DRα and DRβ is similar in the presence or absence of Ii. Data are representative of two independent experiments.
DISCUSSION
Regulation of MHC class II antigen presentation is a complex, tightly controlled process. Transcriptionally, it is achieved through a master regulator, CIITA; however, post-transcriptional regulation is also important. In murine dendritic cells, for example, surface localization is controlled through ubiquitination of a single lysine residue present in the cytoplasmic tail of IA-β (9, 11). The E3 ligase responsible for the maturation-dependent stabilization of HLA-DR, in human dendritic cells, has been identified as a member of the MARCH family of E3 ligases (12). Here we show that, unlike the murine IA molecule, HLA-DR is subject to MARCH-induced polyubiquitination on both the α and β chains. Ubiquitination of the α chain alone is sufficient for efficient MARCH I- and MARCH VIII-induced down-regulation of HLA-DR from the cell surface. The β chain-encoded lysine is conserved in HLA-DP, -DQ, and -DR and is also conserved across species, between humans and mice. In contrast, the α chain lysine is not present in HLA-DP, -DQ, or IA but is conserved across species, between DRα and IE-α, implying that it may have an isotype-specific function. Although polyubiquitination is mainly associated with proteasomal degradation, it also functions as an endocytosis signal. Ubiquitination can control internalization, either directly from the cell surface, or at later stages of the endocytic pathway (17). We removed the dileucine motif from HLA-DRβ and observed that MARCH driven down-regulation of HA-DRB and HA-DRB-L235A,L236A was indistinguishable. Therefore, down-regulation was not dependent upon prior internalization driven by the dileucine endocytosis signal (18). This is consistent with localization of MARCH I to the plasma membrane (Fig. 7) and implicates this as a possible location where ubiquitination occurs (12).
Both MARCH I and MARCH VIII localized, as expected, to both early and late endosomes, together with expression at the cell surface (12). In both the presence and absence of Ii, class II co-localized with MARCH I in Lamp1-positive compartments and at the cell surface, implicating these locations as possible sites of ubiquitination. Intracellular colocalization with MARCH I did not appear to be influenced by ubiquitination, since wild-type DR and DRA-K219R/DRB-K225R remained predominantly in Lamp1-positive compartments and showed a similar degree of colocalization with MARCH I.
Ub contains seven internal lysine residues that can themselves be attached to additional Ub monomers to generate Ub chains. The nature of the polyubiquitin linkage is important. UbLys-48 polyubiquitination is mainly associated with proteasomal degradation, and UbLys-63 polyubiquitination is linked to numerous functions, including cell surface internalization and late endosomal/lysosomal targeting (19, 20). We attempted to determine the nature of the linkage using UbK48R and UbK63R expression constructs, as has been shown for MHC class I (21). Analogous to that study, we generated stable MARCH I transfectants in Mel JuSo cells that expressed reduced levels of surface MHC class II. We were unable to rescue surface class II expression through expression of UbK48R and UbK63R, probably due to insufficient levels of expression of the mutant Ub constructs (data not shown). Receptor Ub may determine the internalization pathway used (22), since it may allow UbLys-63-tagged receptors access to the interior of the multivesicular body (23). Given the importance of localization of MHC class II molecules to antigen-processing compartments, it will be of interest to confirm the nature of the Ub linkage on DRα and DRβ and its role in MVB localization. We are attempting to do this using a lentivirus expression system. Ubiquitin chains are proposed to contain only one type of linkage (24). Ubiquitination of DRα would allow the DR heterodimer to be modified by distinct linkage types affording differential regulation.
We investigated ubiquitination of the individual HLA-DR α and β chains by the MARCH proteins using CD8 as a reporter molecule. Constructs comprising the transmembrane and cytoplasmic domains of DRα and DRβ were independently recognized by both E3 ligases, and down-regulation was lysine-dependent. However, similar to the situation with the DR dimer, CD8-DRB was more extensively ubiquitinated than CD8-DRA. This difference correlated with the cytoplasmic tail domains. When expressed with the DRα transmembrane domain, the DRβ tail was extensively ubiquitinated, and when expressed with the DRβ transmembrane domain, the DRα tail was less extensively ubiquitinated. We considered whether this disparity was due to the DRβ tail facilitating ubiquitination or the DRα tail antagonizing ubiquitination. Since sequential truncations, up to lysine 225, and substitution of adjacent residues for alanine had a minimal effect on MARCH-induced down-regulation of CD8-DRB, we concluded that sequence elements in the DRβ tail were unlikely to facilitate ubiquitination. Although lysine 225 is clearly required, it may not be part of a recognition motif (25). Consistent with the view that features associated with the DRα tail led to reduced Ub, we found that substitution of the tail of DRα for a synthetic tail 215AAAAKAAA222 actually enhanced surface down-regulation in a CD8-DRA reporter assay. It is clear that ubiquitination can occur on lysine residues present in synthetic tails, implying that the sequence context of the lysine is of minor importance. However, even short tails can contain important additional information, as is seen with the 7-amino acid peptide of carboxypeptidase, which, when attached to the cytoplasmic tail of a reporter molecule, allowed sorting of a then ubiquitinated protein into multivesicular body vesicles (17, 26). It will be of interest to identify which elements in the DRα tail are involved in regulating ubiquitination and how this is achieved. The consequence of ubiquitination of DRα also remains to be determined; in particular, it will be important to determine if it confers isotype-specific regulation of HLA-DR compared with HLA-DP and -DQ.
Acknowledgments
We thank Professor Satoshi Ishido (Yokohama, Japan), Professor Paul Lehner, and Dr. Chiwen Chang (Cambridge, UK) for providing cDNA constructs and Professor Paul Lehner (Cambridge, UK) for critical discussion.
This work was supported by the Wellcome Trust and the Medical Research Council. Partial funding was obtained from the National Institute for Health Research Cambridge Biomedical Research Centre. The costs of publication of this article were defrayed in part by the payment of page charges. This article must therefore be hereby marked “advertisement” in accordance with 18 U.S.C. Section 1734 solely to indicate this fact.
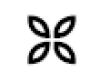
Author's Choice—Final version full access.
Footnotes
The abbreviations used are: MHC, major histocompatibility complex; Ub, ubiquitin; Ii, invariant chain; ER, endoplasmic reticulum; E3, ubiquitin-protein isopeptide ligase; PBS, phosphate-buffered saline; HA, hemagglutinin; FACS, fluorescence-activated cell sorting; HRP, horseradish peroxidase; GFP, green fluorescent protein.
References
- 1.Peters, P. J., Neefjes, J. J., Oorschot, V., Ploegh, H. L., and Geuze, H. J. (1991) Nature 349 669-676 [DOI] [PubMed] [Google Scholar]
- 2.Cresswell, P. (1992) Curr. Opin. Immunol. 4 87-92 [DOI] [PubMed] [Google Scholar]
- 3.Denzin, L. K., and Cresswell, P. (1995) Cell 82 155-165 [DOI] [PubMed] [Google Scholar]
- 4.Watts, C. (2004) Nat. Immunol. 5 685-692 [DOI] [PubMed] [Google Scholar]
- 5.Hiltbold, E. M., and Roche, P. A. (2002) Curr. Opin. Immunol. 14 30-35 [DOI] [PubMed] [Google Scholar]
- 6.Neefjes, J. (1999) Eur. J. Immunol. 29 1421-1425 [DOI] [PubMed] [Google Scholar]
- 7.van Lith, M., van Ham, M., Griekspoor, A., Tjin, E., Verwoerd, D., Calafat, J., Janssen, H., Reits, E., Pastoors, L., and Neefjes, J. (2001) J. Immunol. 167 884-892 [DOI] [PubMed] [Google Scholar]
- 8.Zwart, W., Griekspoor, A., Kuijl, C., Marsman, M., van Rheenen, J., Janssen, H., Calafat, J., van Ham, M., Janssen, L., van Lith, M., Jalink, K., and Neefjes, J. (2005) Immunity 22 221-233 [DOI] [PubMed] [Google Scholar]
- 9.Shin, J. S., Ebersold, M., Pypaert, M., Delamarre, L., Hartley, A., and Mellman, I. (2006) Nature 444 115-118 [DOI] [PubMed] [Google Scholar]
- 10.Ohmura-Hoshino, M., Matsuki, Y., Aoki, M., Goto, E., Mito, M., Uematsu, M., Kakiuchi, T., Hotta, H., and Ishido, S. (2006) J. Immunol. 177 341-354 [DOI] [PubMed] [Google Scholar]
- 11.van Niel, G., Wubbolts, R., Ten Broeke, T., Buschow, S. I., Ossendorp, F. A., Melief, C. J., Raposo, G., van Balkom, B. W., and Stoorvogel, W. (2006) Immunity 25 885-894 [DOI] [PubMed] [Google Scholar]
- 12.De Gassart, A., Camosseto, V., Thibodeau, J., Ceppi, M., Catalan, N., Pierre, P., and Gatti, E. (2008) Proc. Natl. Acad. Sci. U. S. A. 105 3491-3496 [DOI] [PMC free article] [PubMed] [Google Scholar]
- 13.Matsuki, Y., Ohmura-Hoshino, M., Goto, E., Aoki, M., Mito-Yoshida, M., Uematsu, M., Hasegawa, T., Koseki, H., Ohara, O., Nakayama, M., Toyooka, K., Matsuoka, K., Hotta, H., Yamamoto, A., and Ishido, S. (2007) EMBO J. 26 846-854 [DOI] [PMC free article] [PubMed] [Google Scholar]
- 14.Pickart, C. M., and Fushman, D. (2004) Curr. Opin. Chem. Biol. 8 610-616 [DOI] [PubMed] [Google Scholar]
- 15.Goto, E., Mito-Yoshida, M., Uematsu, M., Aoki, M., Matsuki, Y., Ohmura-Hoshino, M., Hotta, H., Miyagishi, M., and Ishido, S. (2008) PLoS ONE 3 1490. [DOI] [PMC free article] [PubMed] [Google Scholar]
- 16.Coscoy, L., Sanchez, D. J., and Ganem, D. (2001) J. Cell Biol. 155 1265-1273 [DOI] [PMC free article] [PubMed] [Google Scholar]
- 17.Katzmann, D. J., Odorizzi, G., and Emr, S. D. (2002) Nat. Rev. Mol. Cell. Biol. 3 893-905 [DOI] [PubMed] [Google Scholar]
- 18.Zhong, G., Romagnoli, P., and Germain, R. N. (1997) J. Exp. Med. 185 429-438 [DOI] [PMC free article] [PubMed] [Google Scholar]
- 19.Nakatsu, F., Sakuma, M., Matsuo, Y., Arase, H., Yamasaki, S., Nakamura, N., Saito, T., and Ohno, H. (2000) J. Biol. Chem. 275 26213-26219 [DOI] [PubMed] [Google Scholar]
- 20.Galan, J. M., and Haguenauer-Tsapis, R. (1997) EMBO J. 16 5847-5854 [DOI] [PMC free article] [PubMed] [Google Scholar]
- 21.Duncan, L. M., Piper, S., Dodd, R. B., Saville, M. K., Sanderson, C. M., Luzio, J. P., and Lehner, P. J. (2006) EMBO J. 25 1635-1645 [DOI] [PMC free article] [PubMed] [Google Scholar]
- 22.Mukherjee, K., Siddiqi, S. A., Hashim, S., Raje, M., Basu, S. K., and Mukhopadhyay, A. (2000) J. Cell Biol. 148 741-753 [DOI] [PMC free article] [PubMed] [Google Scholar]
- 23.Komada, M., and Kitamura, N. (2005) J. Biochem. (Tokyo) 137 1-8 [DOI] [PubMed] [Google Scholar]
- 24.Peng, J., Schwartz, D., Elias, J. E., Thoreen, C. C., Cheng, D., Marsischky, G., Roelofs, J., Finley, D., and Gygi, S. P. (2003) Nat. Biotechnol. 21 921-926 [DOI] [PubMed] [Google Scholar]
- 25.Ciechanover, A. (1998) EMBO J. 17 7151-7160 [DOI] [PMC free article] [PubMed] [Google Scholar]
- 26.Katzmann, D. J., Babst, M., and Emr, S. D. (2001) Cell 106 145-155 [DOI] [PubMed] [Google Scholar]