Abstract
Cells often acquire resistance to the antiproliferative agents methotrexate (MTX) or N-phosphonacetyl-L-aspartate (PALA) through amplification of genes encoding the target enzymes dihydrofolate reductase or carbamylphosphate synthetase/aspartate transcarbamylase/dihydroorotase (CAD), respectively. We showed previously that Syrian hamster BHK cells resistant to selective concentrations of PALA (approximately 3 x ID50) arise at a rate of approximately 10(-4) per cell per generation and contain amplifications of the CAD gene as ladder-like structures on one of the two B9 chromosomes, where CAD is normally located. We now find that BHK cells resistant to high concentrations of PALA (approximately 15 x ID50) appear only after prior exposure to selective concentrations of PALA for approximately 72 h. Furthermore, in contrast to untreated cells, BHK cells pretreated with selective concentrations of MTX give colonies in high concentrations of PALA, and cells pretreated with selective concentrations of PALA give colonies in high concentrations of MTX or 5-fluorouracil. As judged by measuring numbers of cells and metaphase cell pairs, BHK cells do not arrest completely when starved for pyrimidine nucleotides by treatment with selective concentrations of PALA for up to 72 h. We propose that DNA damage, caused when cells fail to stop DNA synthesis promptly under conditions of dNTP starvation, stimulates amplification throughout the genome by mechanisms--such as bridge-breakage-fusion cycles--that are triggered by broken DNA. Amplified CAD genes were analyzed by fluorescence in situ hybridization both in cells where amplification was induced by PALA pretreatment and in cells in which the amplification occurred spontaneously, before selection with PALA. The ladder-like structures that result from bridge-breakage-fusion cycles were observed in both cases.
Full text
PDF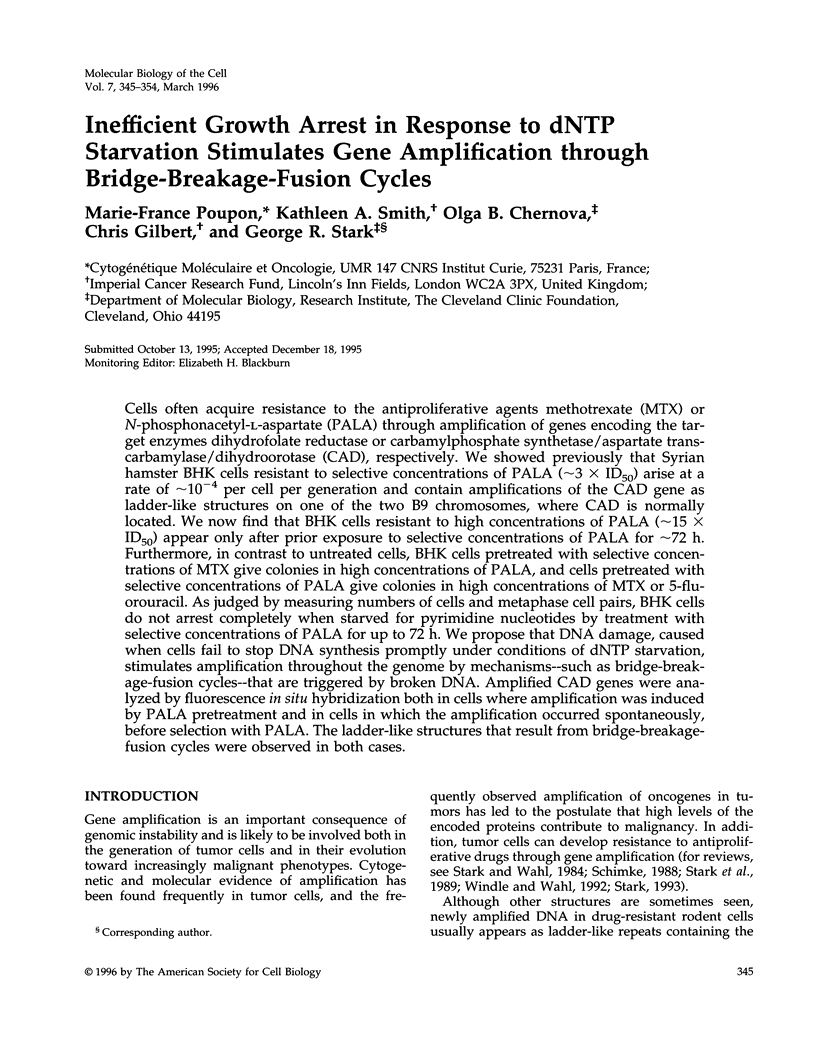
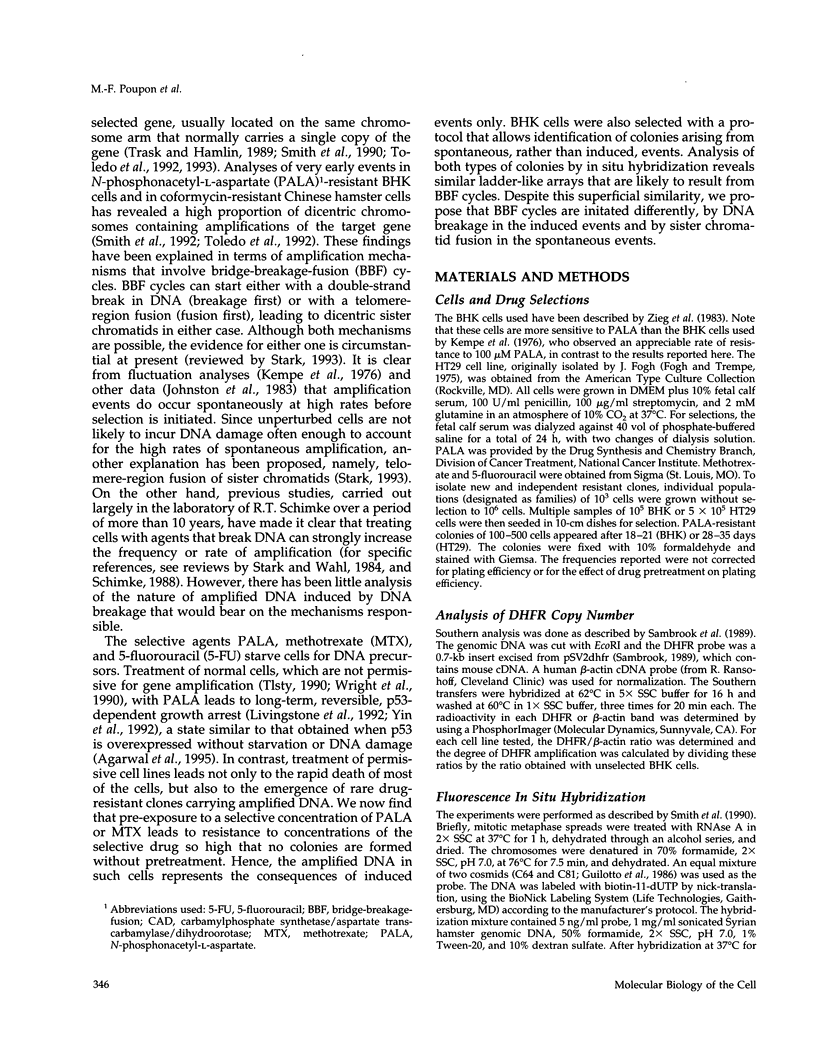
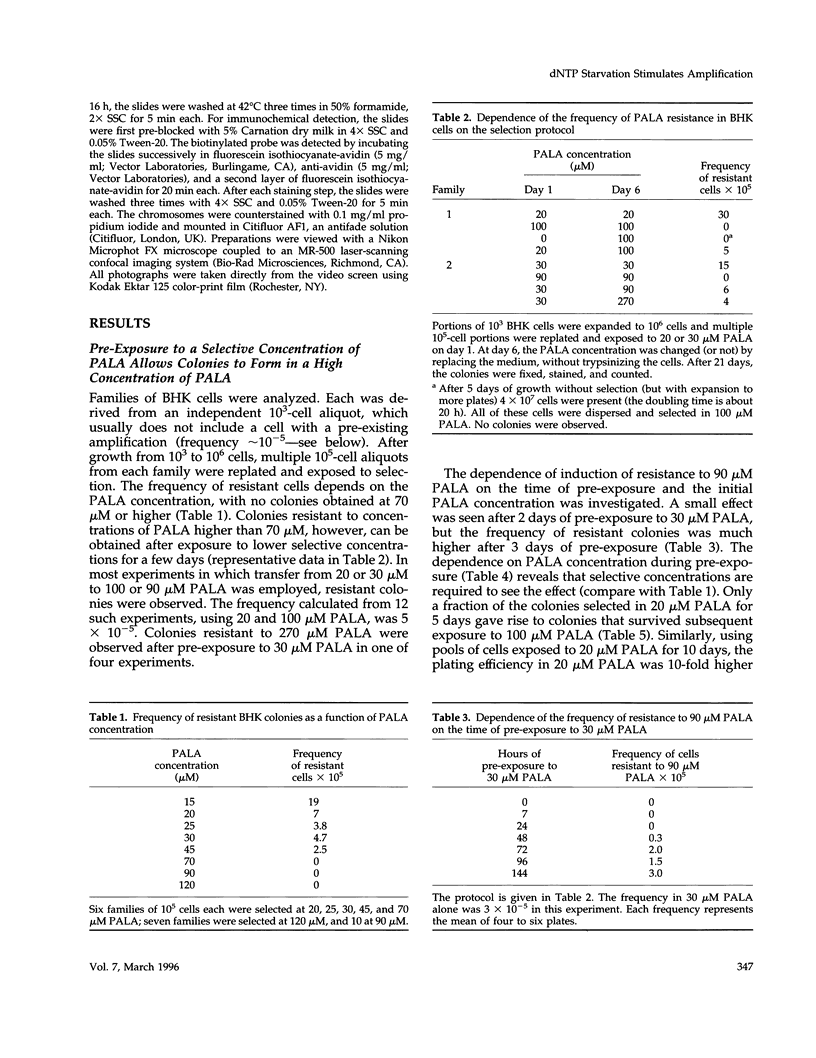
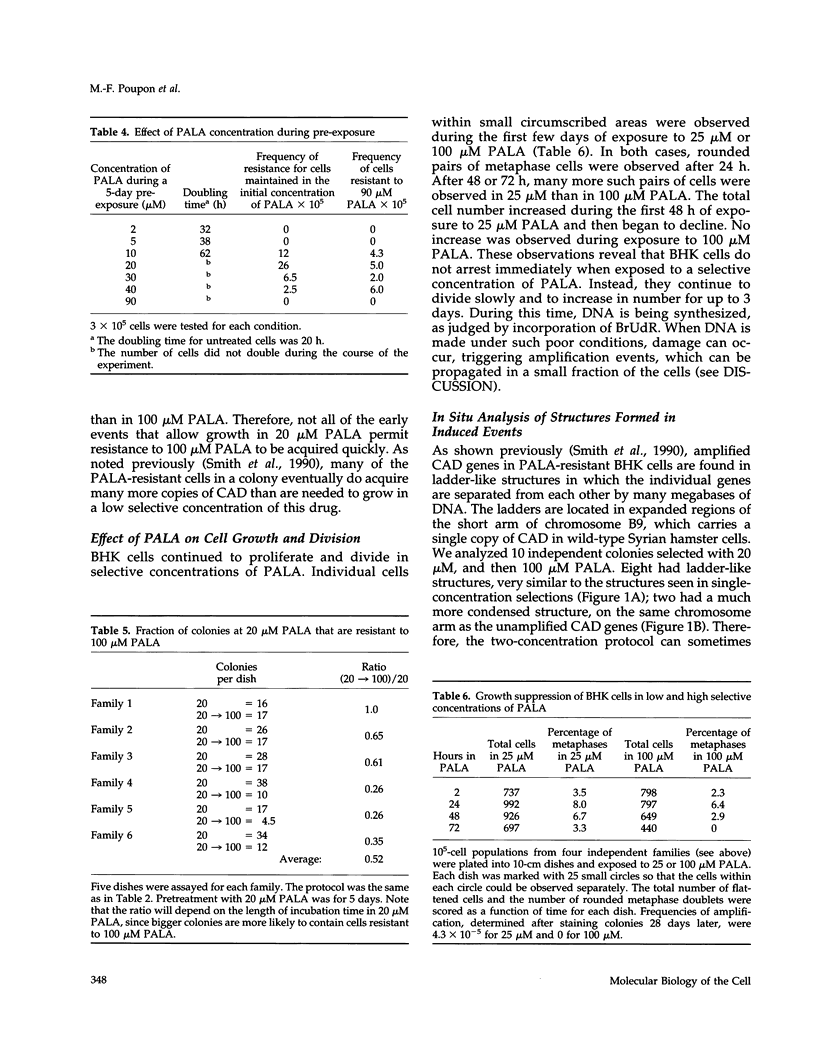
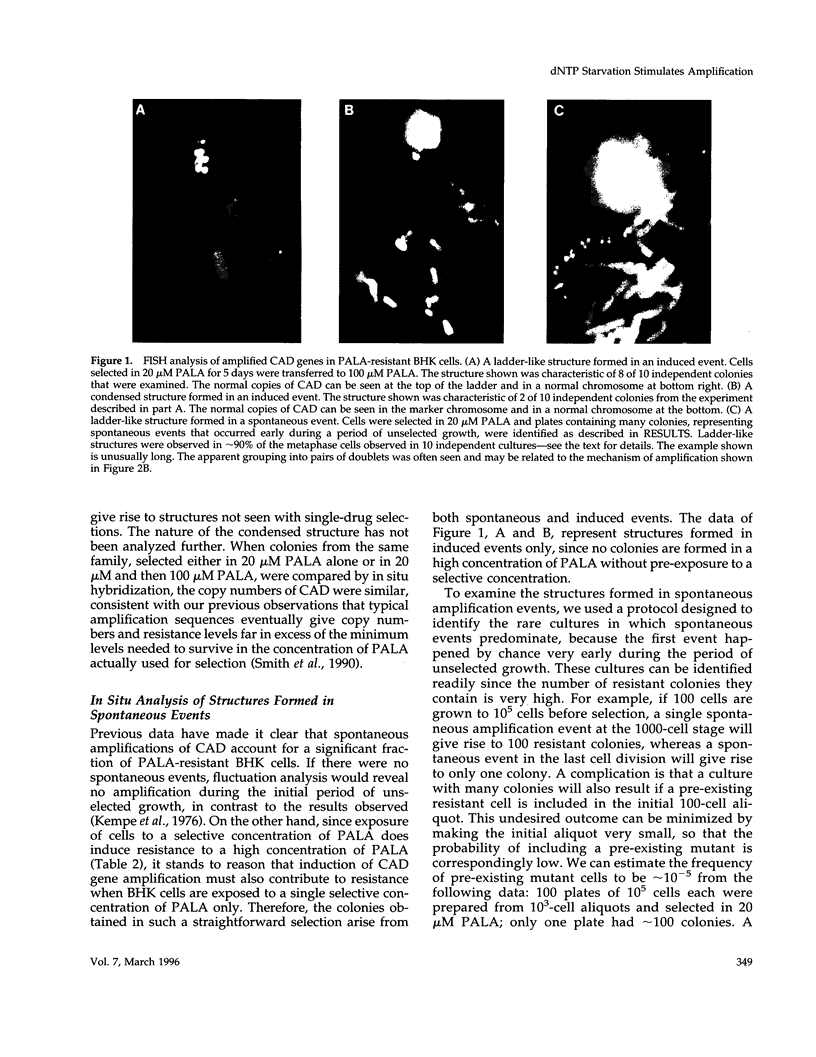
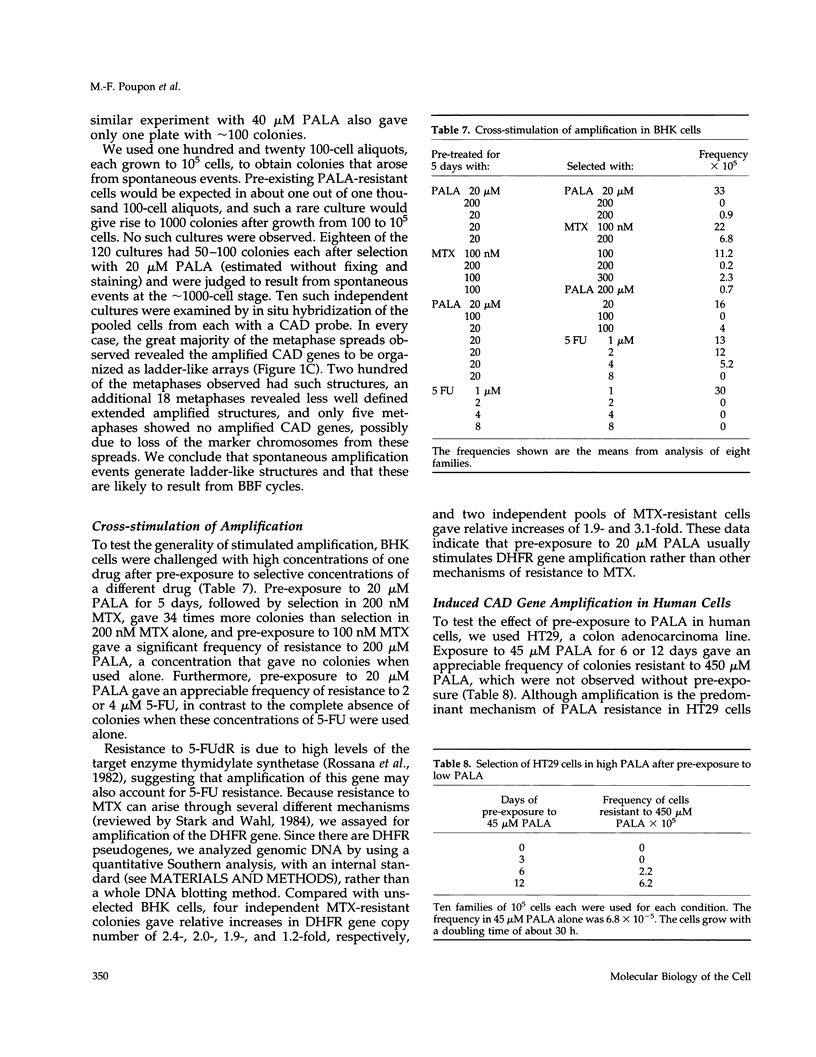
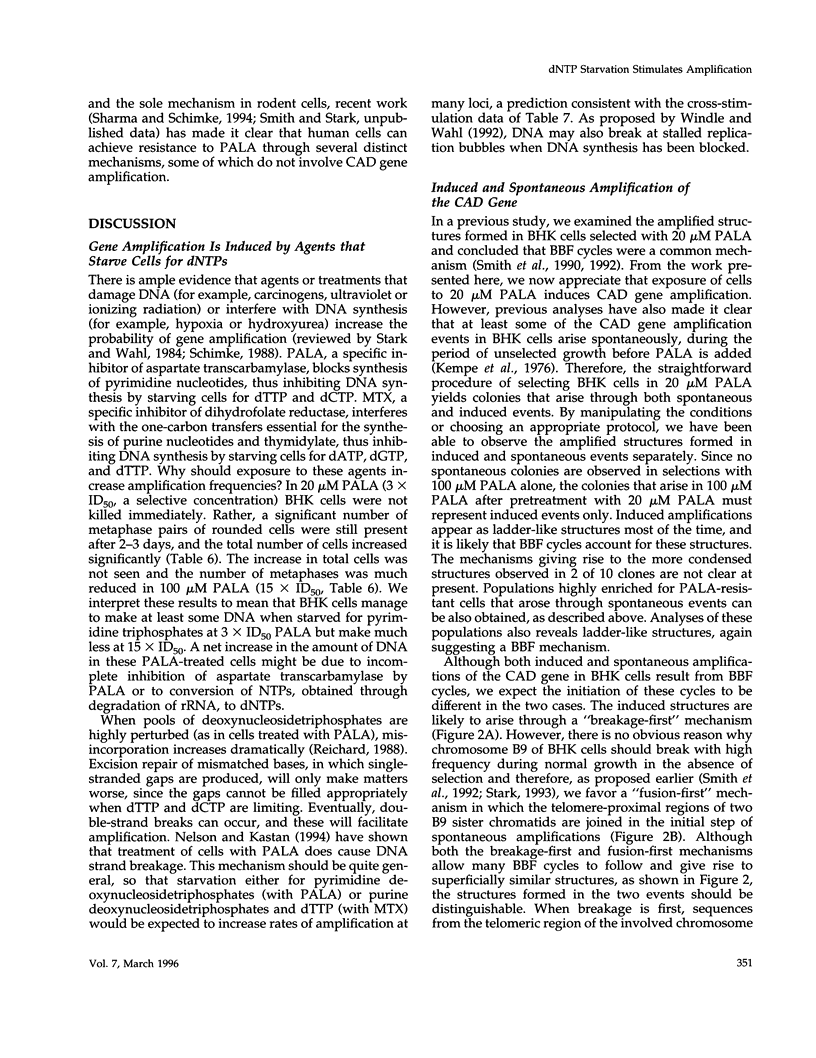
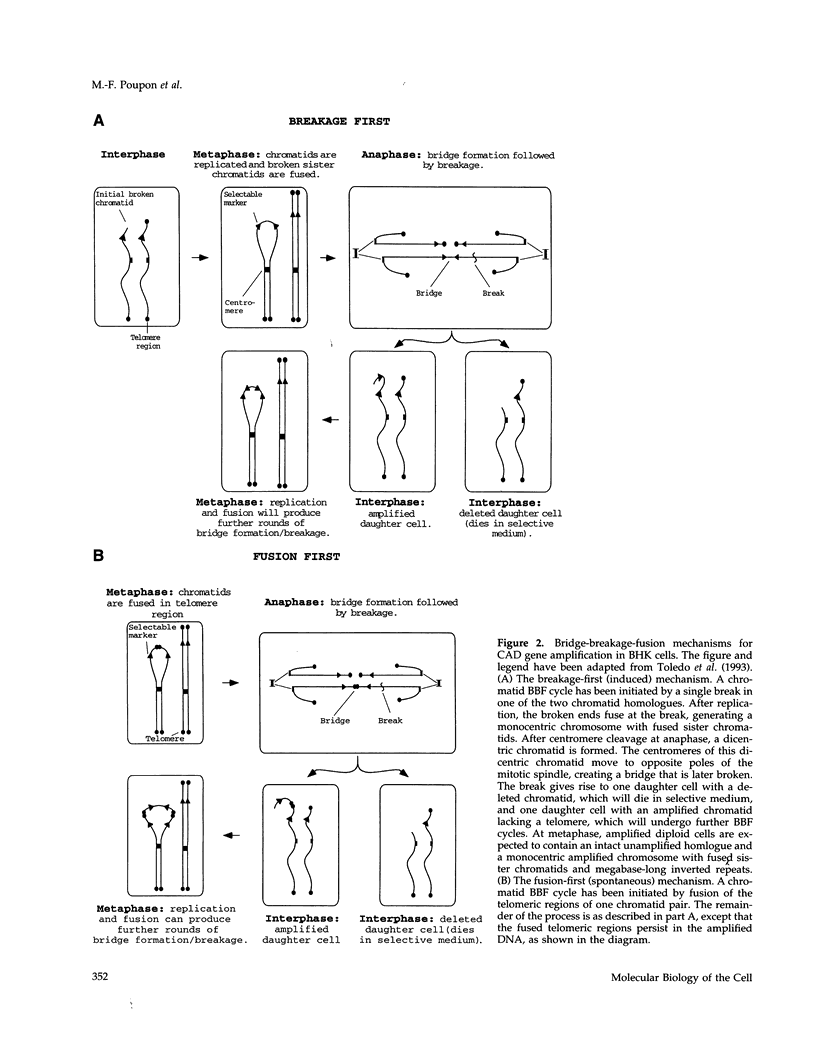
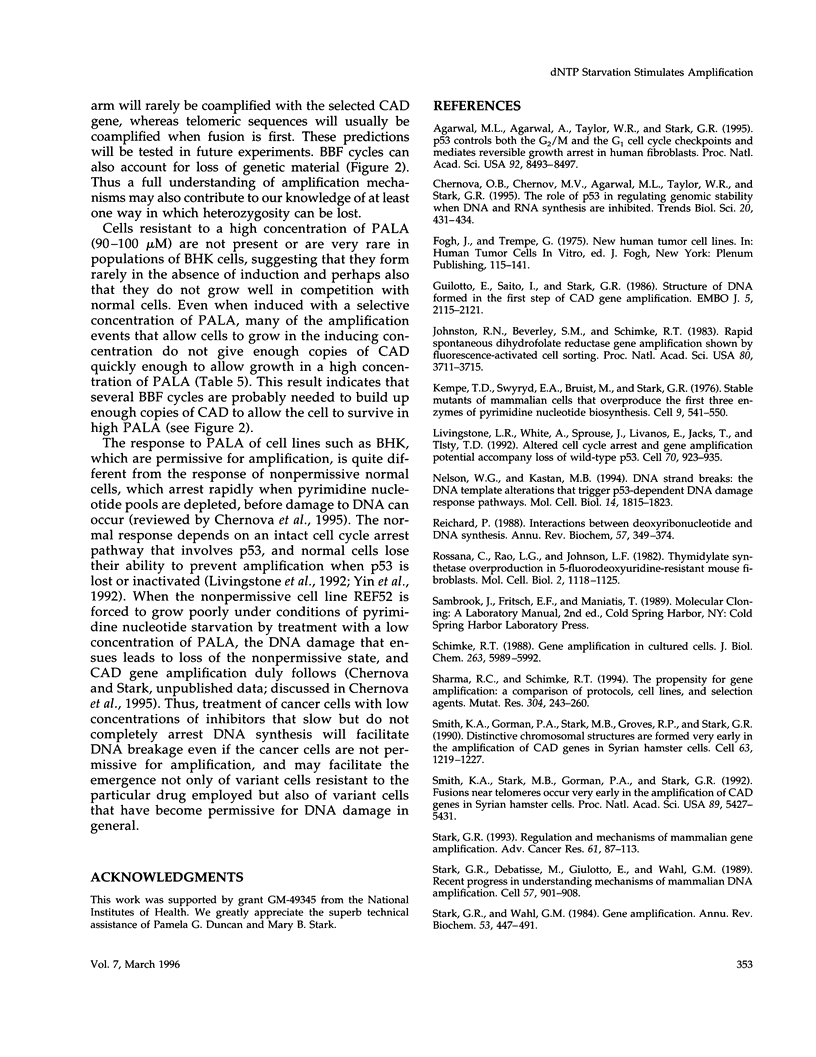
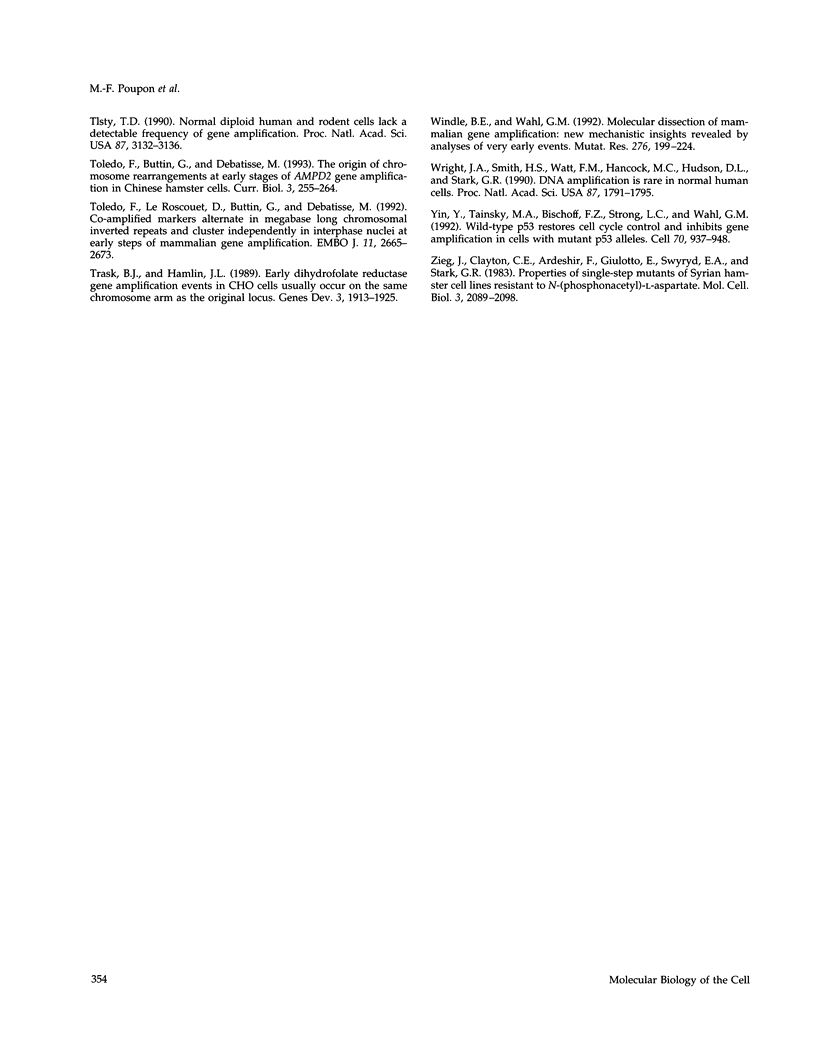
Images in this article
Selected References
These references are in PubMed. This may not be the complete list of references from this article.
- Agarwal M. L., Agarwal A., Taylor W. R., Stark G. R. p53 controls both the G2/M and the G1 cell cycle checkpoints and mediates reversible growth arrest in human fibroblasts. Proc Natl Acad Sci U S A. 1995 Aug 29;92(18):8493–8497. doi: 10.1073/pnas.92.18.8493. [DOI] [PMC free article] [PubMed] [Google Scholar]
- Chernova O. B., Chernov M. V., Agarwal M. L., Taylor W. R., Stark G. R. The role of p53 in regulating genomic stability when DNA and RNA synthesis are inhibited. Trends Biochem Sci. 1995 Oct;20(10):431–434. doi: 10.1016/s0968-0004(00)89094-5. [DOI] [PubMed] [Google Scholar]
- Giulotto E., Saito I., Stark G. R. Structure of DNA formed in the first step of CAD gene amplification. EMBO J. 1986 Sep;5(9):2115–2121. doi: 10.1002/j.1460-2075.1986.tb04474.x. [DOI] [PMC free article] [PubMed] [Google Scholar]
- Johnston R. N., Beverley S. M., Schimke R. T. Rapid spontaneous dihydrofolate reductase gene amplification shown by fluorescence-activated cell sorting. Proc Natl Acad Sci U S A. 1983 Jun;80(12):3711–3715. doi: 10.1073/pnas.80.12.3711. [DOI] [PMC free article] [PubMed] [Google Scholar]
- Kempe T. D., Swyryd E. A., Bruist M., Stark G. R. Stable mutants of mammalian cells that overproduce the first three enzymes of pyrimidine nucleotide biosynthesis. Cell. 1976 Dec;9(4 Pt 1):541–550. doi: 10.1016/0092-8674(76)90036-2. [DOI] [PubMed] [Google Scholar]
- Livingstone L. R., White A., Sprouse J., Livanos E., Jacks T., Tlsty T. D. Altered cell cycle arrest and gene amplification potential accompany loss of wild-type p53. Cell. 1992 Sep 18;70(6):923–935. doi: 10.1016/0092-8674(92)90243-6. [DOI] [PubMed] [Google Scholar]
- Nelson W. G., Kastan M. B. DNA strand breaks: the DNA template alterations that trigger p53-dependent DNA damage response pathways. Mol Cell Biol. 1994 Mar;14(3):1815–1823. doi: 10.1128/mcb.14.3.1815. [DOI] [PMC free article] [PubMed] [Google Scholar]
- Reichard P. Interactions between deoxyribonucleotide and DNA synthesis. Annu Rev Biochem. 1988;57:349–374. doi: 10.1146/annurev.bi.57.070188.002025. [DOI] [PubMed] [Google Scholar]
- Rossana C., Gollakota Rao L., Johnson L. F. Thymidylate synthetase overproduction in 5-fluorodeoxyuridine-resistant mouse fibroblasts. Mol Cell Biol. 1982 Sep;2(9):1118–1125. doi: 10.1128/mcb.2.9.1118. [DOI] [PMC free article] [PubMed] [Google Scholar]
- Schimke R. T. Gene amplification in cultured cells. J Biol Chem. 1988 May 5;263(13):5989–5992. [PubMed] [Google Scholar]
- Sharma R. C., Schimke R. T. The propensity for gene amplification: a comparison of protocols, cell lines, and selection agents. Mutat Res. 1994 Jan 16;304(2):243–260. doi: 10.1016/0027-5107(94)90217-8. [DOI] [PubMed] [Google Scholar]
- Smith K. A., Gorman P. A., Stark M. B., Groves R. P., Stark G. R. Distinctive chromosomal structures are formed very early in the amplification of CAD genes in Syrian hamster cells. Cell. 1990 Dec 21;63(6):1219–1227. doi: 10.1016/0092-8674(90)90417-d. [DOI] [PubMed] [Google Scholar]
- Smith K. A., Stark M. B., Gorman P. A., Stark G. R. Fusions near telomeres occur very early in the amplification of CAD genes in Syrian hamster cells. Proc Natl Acad Sci U S A. 1992 Jun 15;89(12):5427–5431. doi: 10.1073/pnas.89.12.5427. [DOI] [PMC free article] [PubMed] [Google Scholar]
- Stark G. R., Debatisse M., Giulotto E., Wahl G. M. Recent progress in understanding mechanisms of mammalian DNA amplification. Cell. 1989 Jun 16;57(6):901–908. doi: 10.1016/0092-8674(89)90328-0. [DOI] [PubMed] [Google Scholar]
- Stark G. R. Regulation and mechanisms of mammalian gene amplification. Adv Cancer Res. 1993;61:87–113. doi: 10.1016/s0065-230x(08)60956-2. [DOI] [PubMed] [Google Scholar]
- Stark G. R., Wahl G. M. Gene amplification. Annu Rev Biochem. 1984;53:447–491. doi: 10.1146/annurev.bi.53.070184.002311. [DOI] [PubMed] [Google Scholar]
- Tlsty T. D. Normal diploid human and rodent cells lack a detectable frequency of gene amplification. Proc Natl Acad Sci U S A. 1990 Apr;87(8):3132–3136. doi: 10.1073/pnas.87.8.3132. [DOI] [PMC free article] [PubMed] [Google Scholar]
- Toledo F., Buttin G., Debatisse M. The origin of chromosome rearrangements at early stages of AMPD2 gene amplification in Chinese hamster cells. Curr Biol. 1993 May 1;3(5):255–264. doi: 10.1016/0960-9822(93)90175-n. [DOI] [PubMed] [Google Scholar]
- Toledo F., Le Roscouet D., Buttin G., Debatisse M. Co-amplified markers alternate in megabase long chromosomal inverted repeats and cluster independently in interphase nuclei at early steps of mammalian gene amplification. EMBO J. 1992 Jul;11(7):2665–2673. doi: 10.1002/j.1460-2075.1992.tb05332.x. [DOI] [PMC free article] [PubMed] [Google Scholar]
- Trask B. J., Hamlin J. L. Early dihydrofolate reductase gene amplification events in CHO cells usually occur on the same chromosome arm as the original locus. Genes Dev. 1989 Dec;3(12A):1913–1925. doi: 10.1101/gad.3.12a.1913. [DOI] [PubMed] [Google Scholar]
- Windle B. E., Wahl G. M. Molecular dissection of mammalian gene amplification: new mechanistic insights revealed by analyses of very early events. Mutat Res. 1992 May;276(3):199–224. doi: 10.1016/0165-1110(92)90009-x. [DOI] [PubMed] [Google Scholar]
- Wright J. A., Smith H. S., Watt F. M., Hancock M. C., Hudson D. L., Stark G. R. DNA amplification is rare in normal human cells. Proc Natl Acad Sci U S A. 1990 Mar;87(5):1791–1795. doi: 10.1073/pnas.87.5.1791. [DOI] [PMC free article] [PubMed] [Google Scholar]
- Yin Y., Tainsky M. A., Bischoff F. Z., Strong L. C., Wahl G. M. Wild-type p53 restores cell cycle control and inhibits gene amplification in cells with mutant p53 alleles. Cell. 1992 Sep 18;70(6):937–948. doi: 10.1016/0092-8674(92)90244-7. [DOI] [PubMed] [Google Scholar]
- Zieg J., Clayton C. E., Ardeshir F., Giulotto E., Swyryd E. A., Stark G. R. Properties of single-step mutants of Syrian hamster cell lines resistant to N-(phosphonacetyl)-L-aspartate. Mol Cell Biol. 1983 Nov;3(11):2089–2098. doi: 10.1128/mcb.3.11.2089. [DOI] [PMC free article] [PubMed] [Google Scholar]