Abstract
Despite advances in understanding the cell biology of glycoinositol phospholipid (GPI)-anchored proteins in cultured cells, the in vivo functions of GPI anchors have remained elusive. We have focused on Drosophila acetylcholinesterase (AChE) as a model GPI-anchored protein that can be manipulated in vivo with sophisticated genetic techniques. In Drosophila, AChE is found only as a GPI-anchored G2 form encoded by the Ace locus on the third chromosome. To pursue our goal of replacing wild-type GPI-anchored AChE with forms that have alternative anchor structures in transgenic files, we report the construction of two secreted forms of Drosophila AChE (SEC1 and SEC2) and a chimeric form (TM-AChE) anchored by the transmembrane and cytoplasmic domains of herpes simplex virus type 1 glycoprotein C. To confirm that the biochemical properties of these AChEs were unchanged from GPI-AChE except as predicted, we made stably transfected Drosophila Schneider Line 2(S2) cells expressing each of the four forms. TM-AChE, SEC1, and SEC2 had the same catalytic activity and quaternary structure as wild type. TM-AChE was expressed as an amphiphilic membrane-bound protein resistant to an enzyme that cleaves GPI-AChE (phosphatidylinositol-specific phospholipase C), and the same percentage of TM-AChE and GPI-AChE was on the cell surface according to immunofluorescence and pharmacological data. SEC1 and SEC2 were constructed by truncating the C-terminal signal peptide initially present in GPI-AChE: in SEC1 the last 25 residues of this 34-residue peptide were deleted while in SEC2 the last 29 were deleted. Both SEC1 and SEC2 were efficiently secreted and are very stable in culture medium; with one cloned SEC1-expressing line, AChE accumulated to as high as 100 mg/liter. Surprisingly, 5-10% of SEC1 was attached to a GPI anchor, but SEC2 showed no GPI anchoring. Since no differences in catalytic activity were observed among the four AChEs, and since the same percentage of GPI-AChE and TM-AChE were on the cell surface, we contend that in vivo experiments in which GPI-AChE is replaced can be interpreted solely on the basis of the altered anchoring domain.
Full text
PDF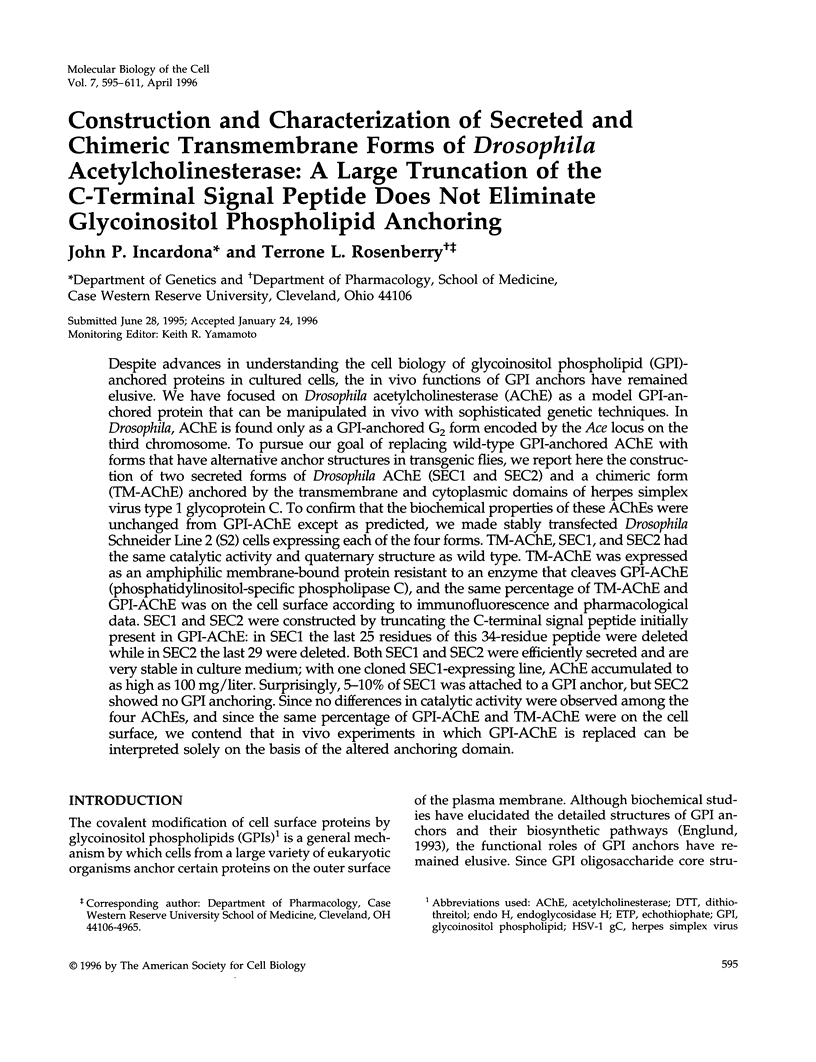
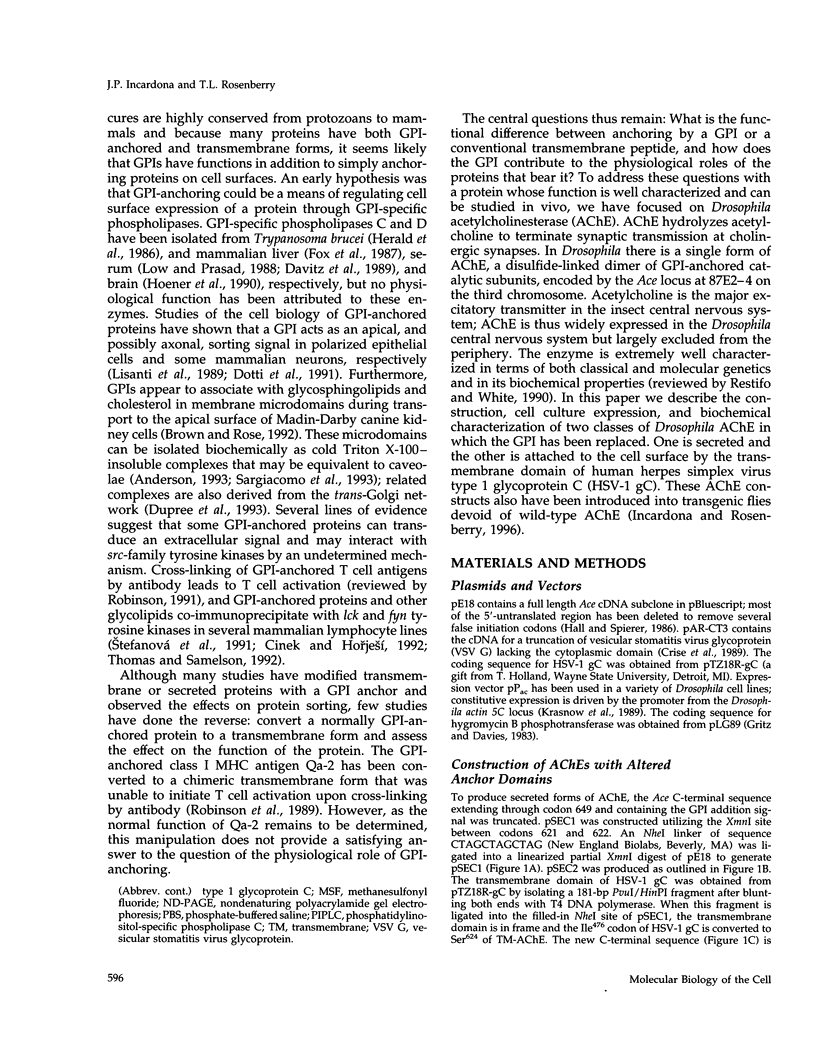
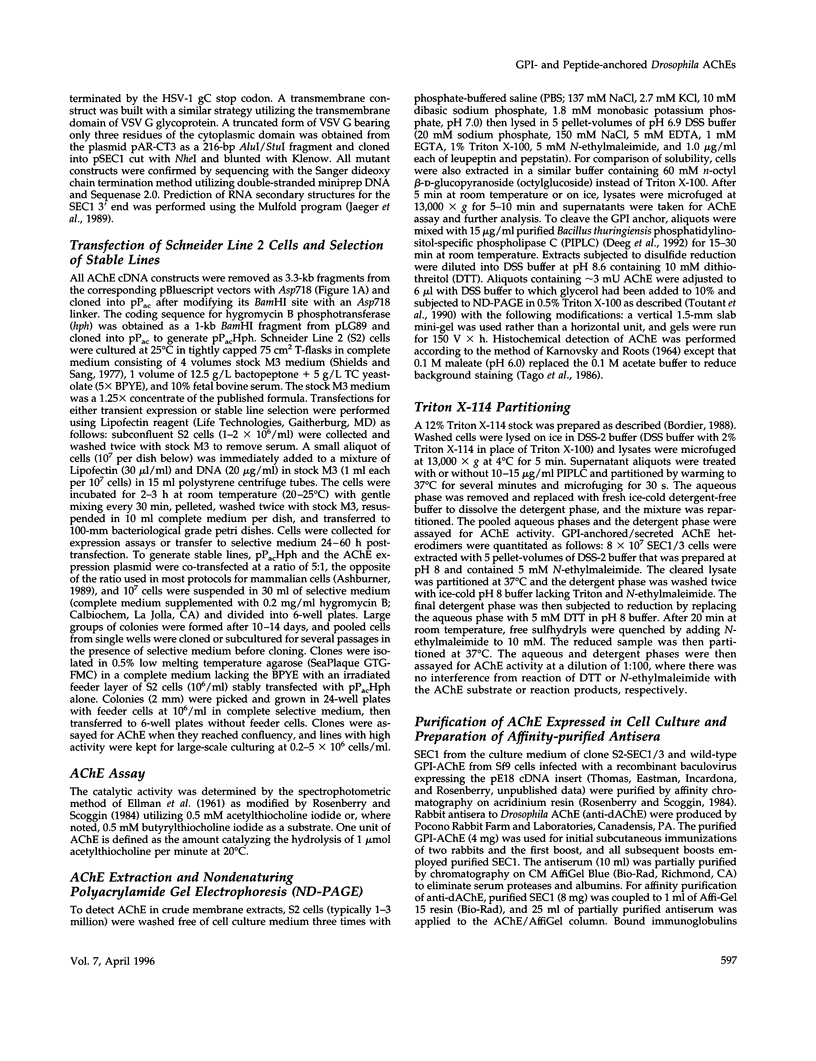
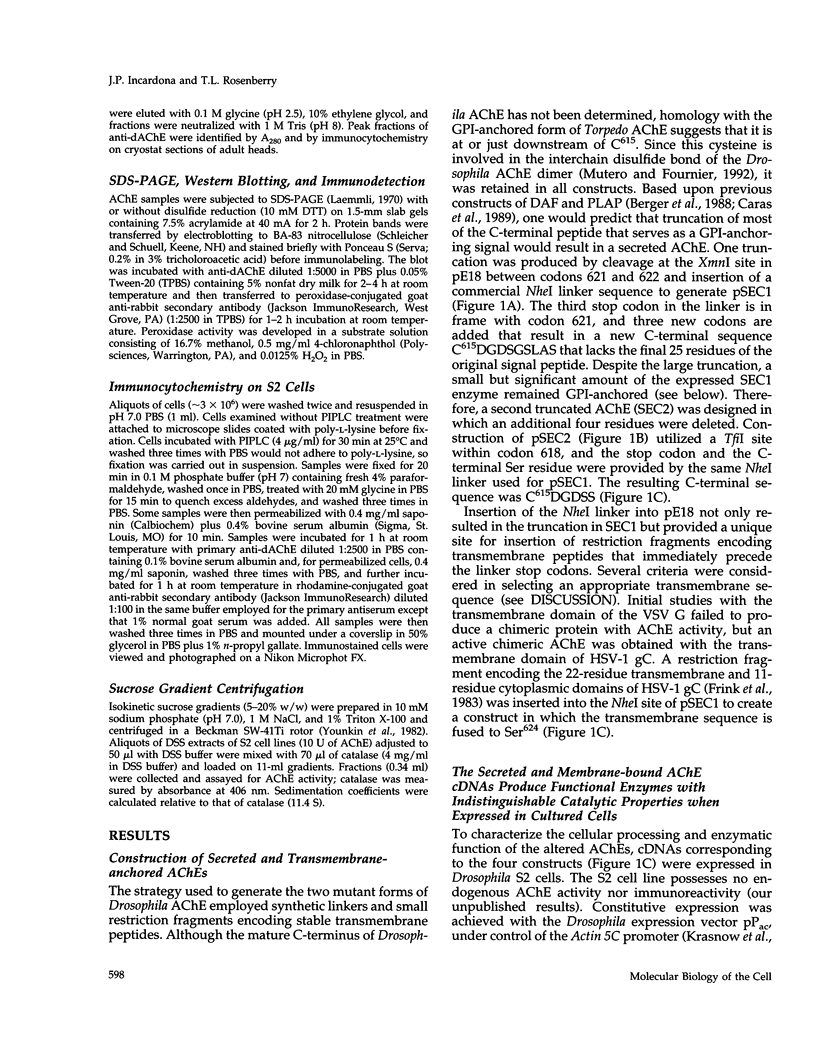
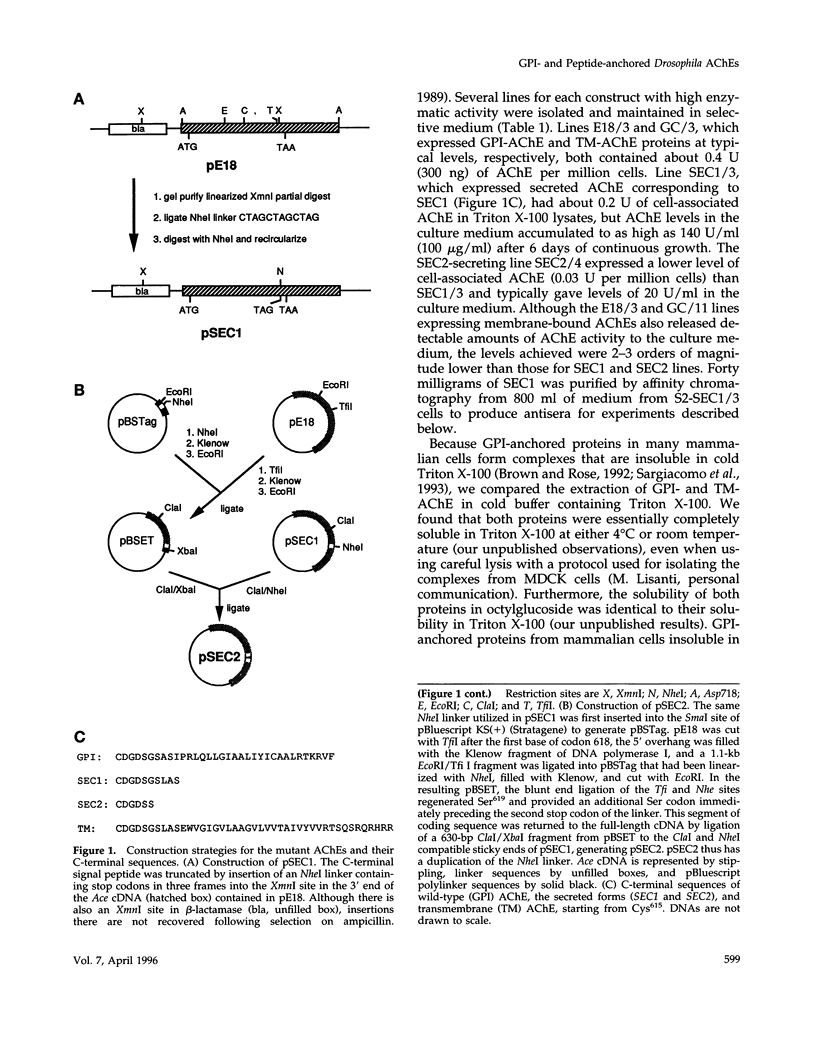
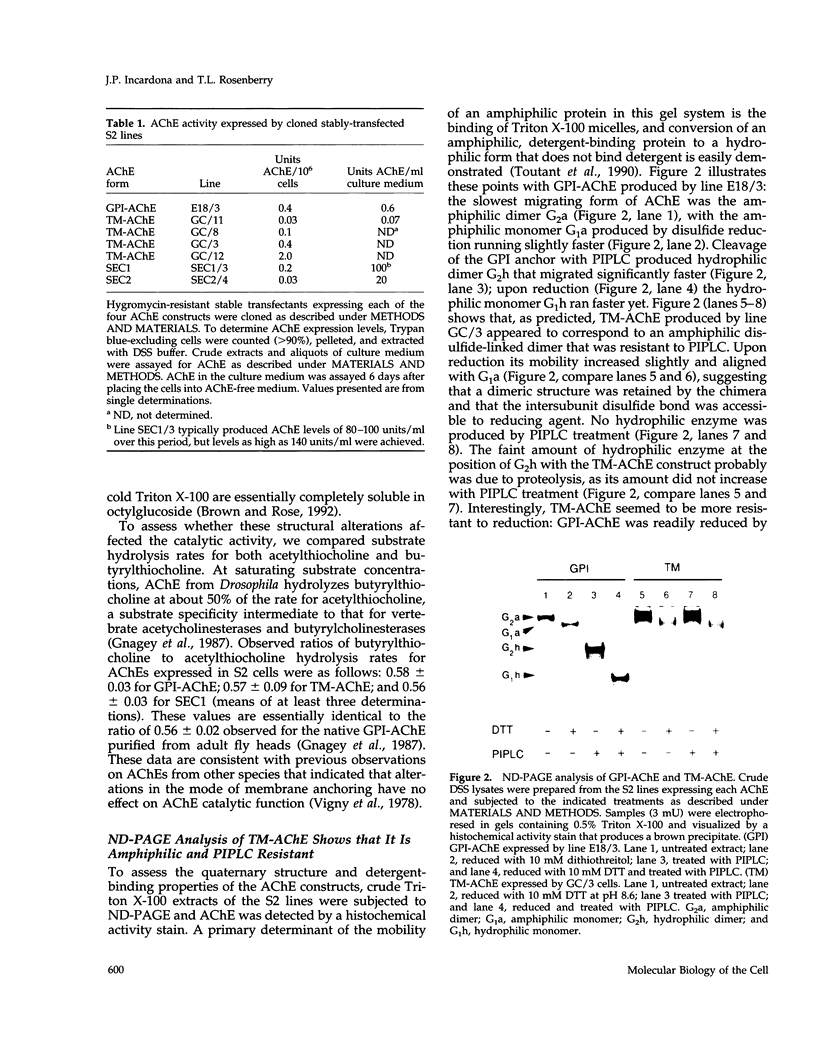
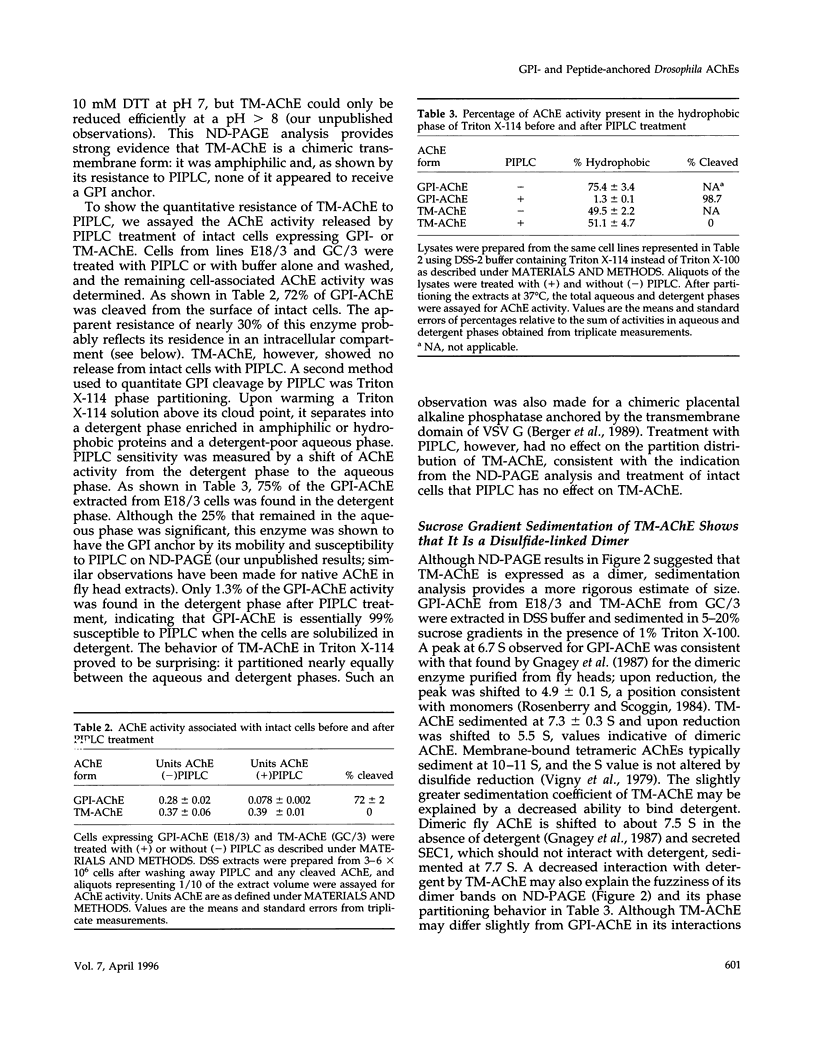
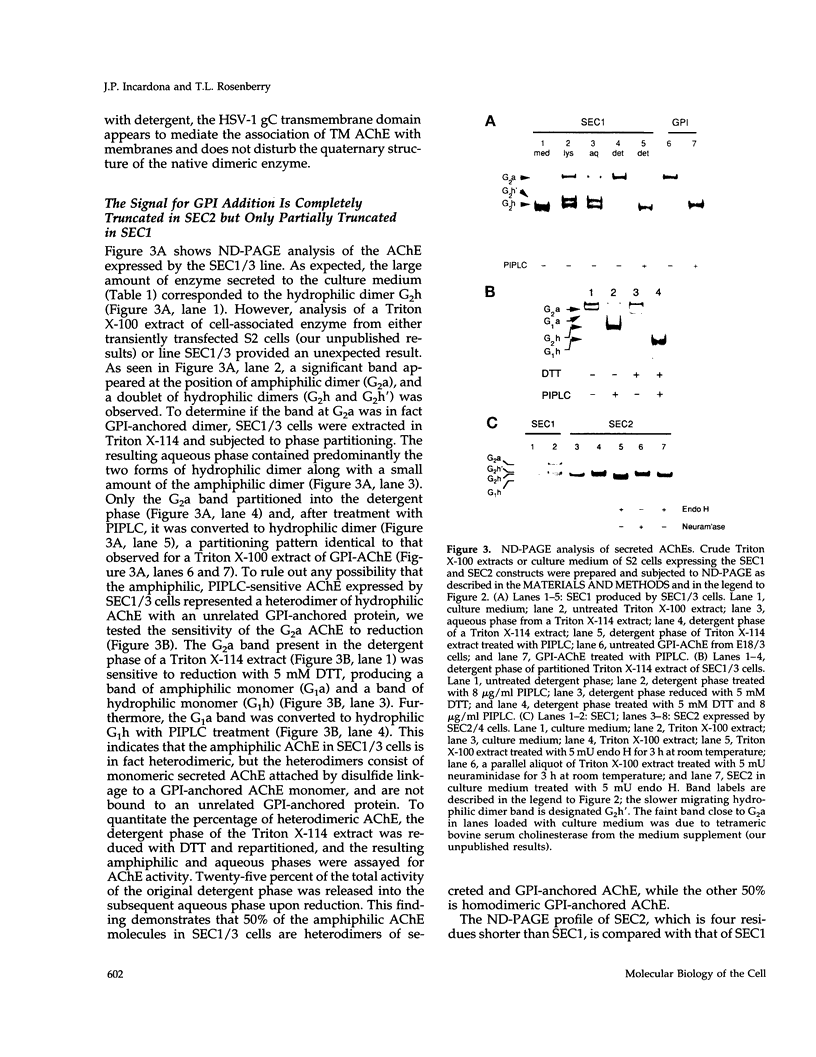
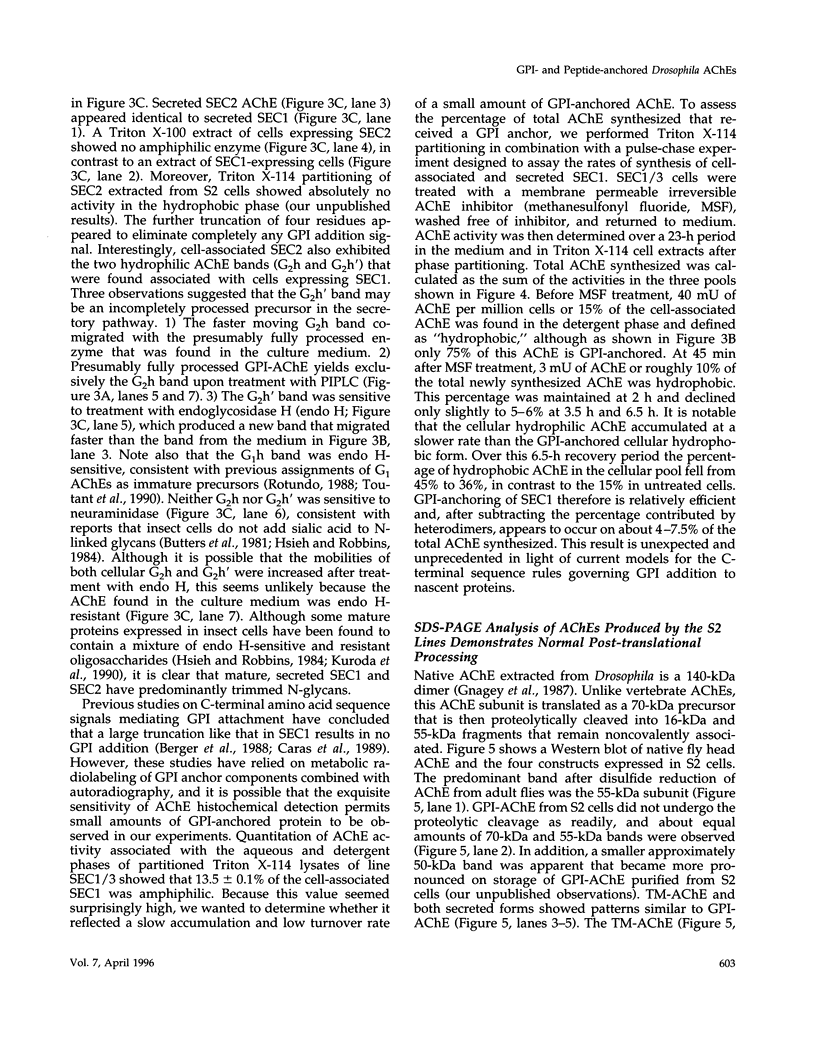
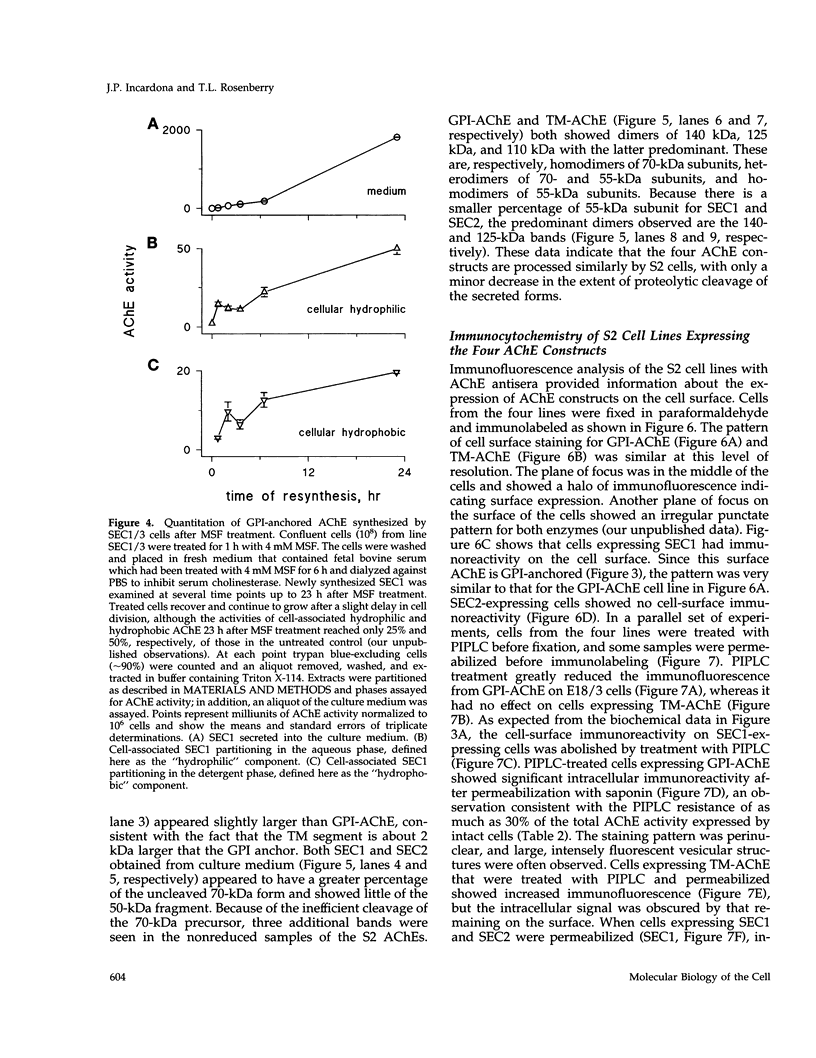
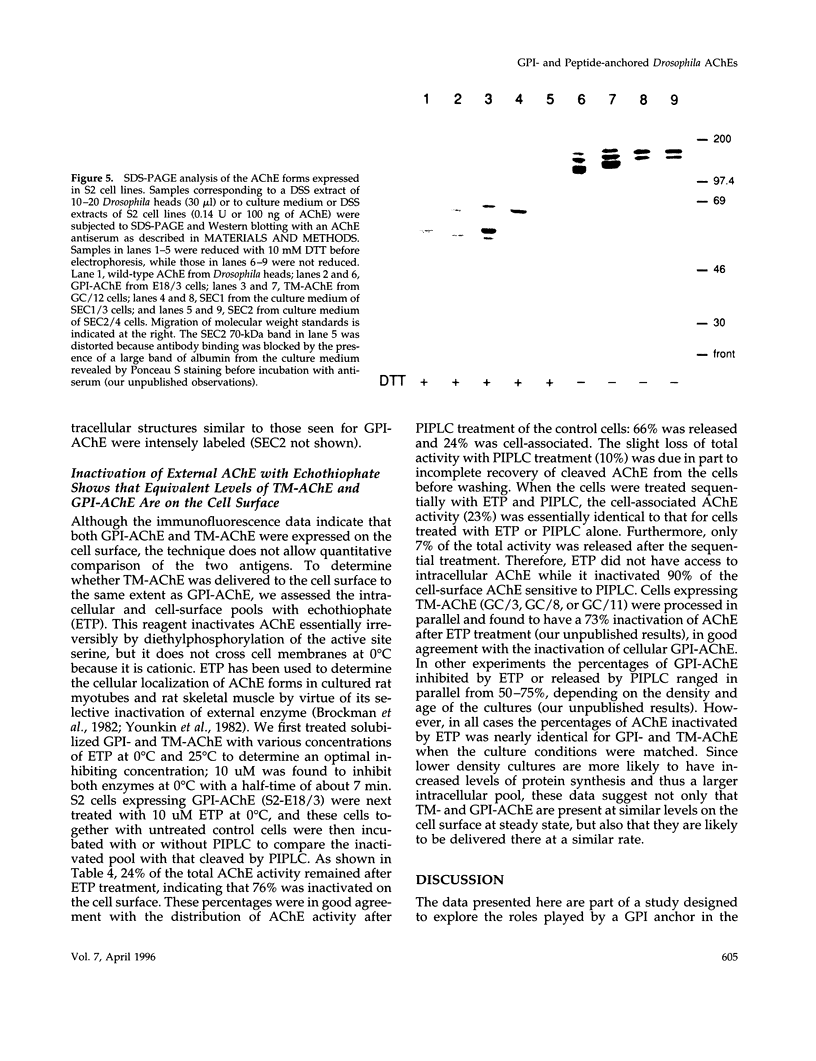
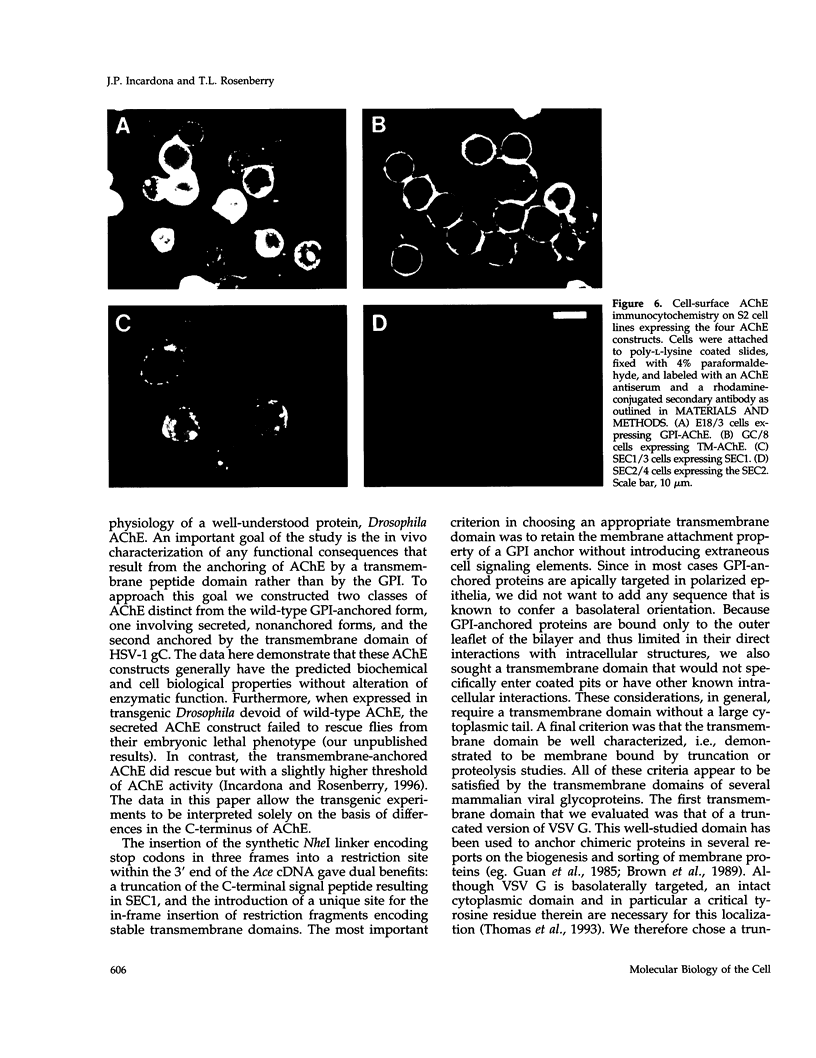
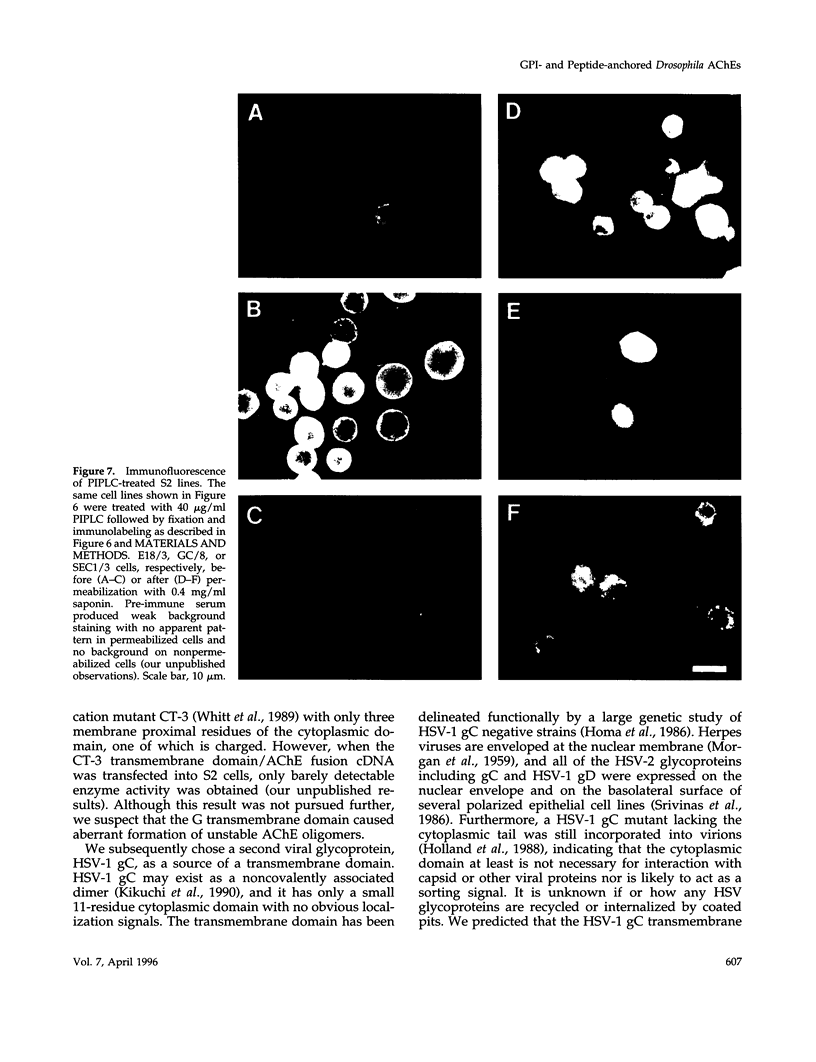
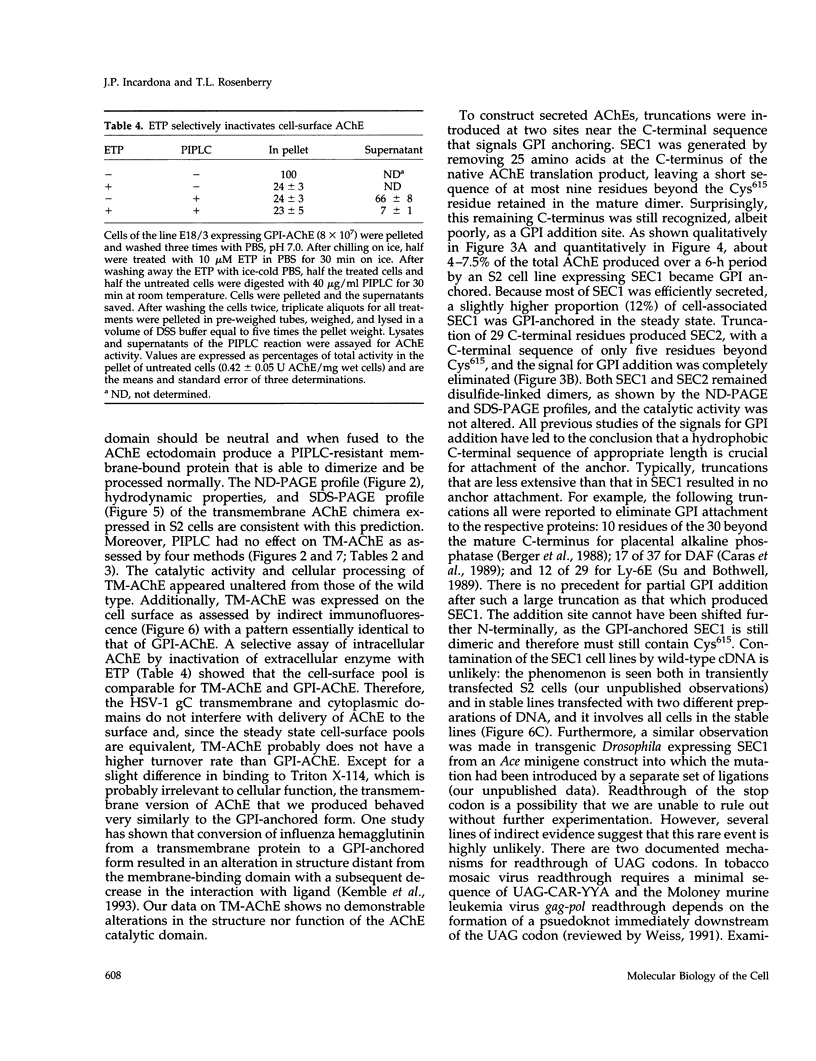
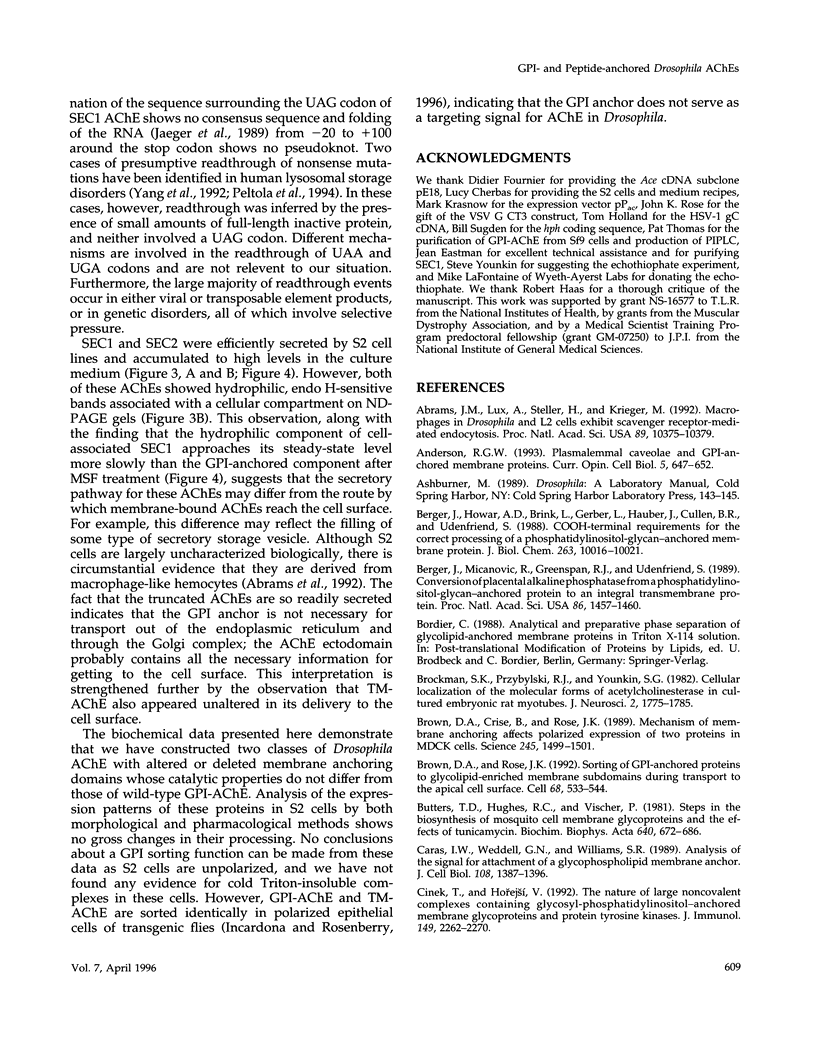
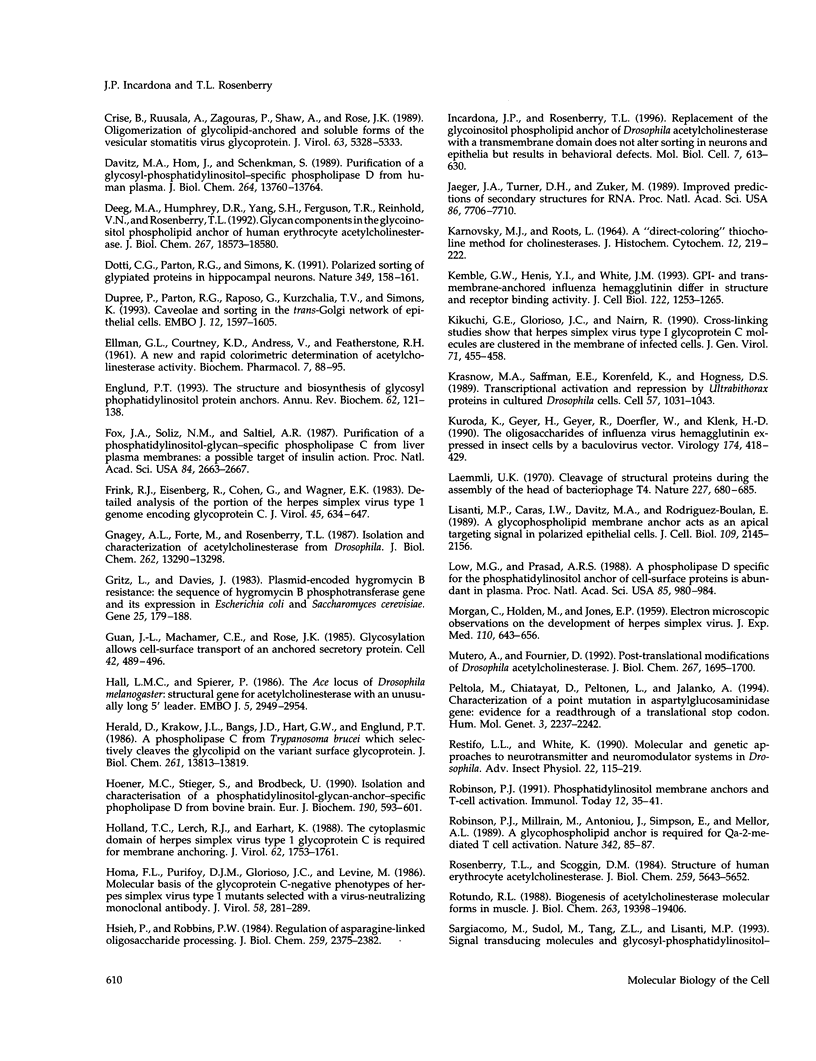
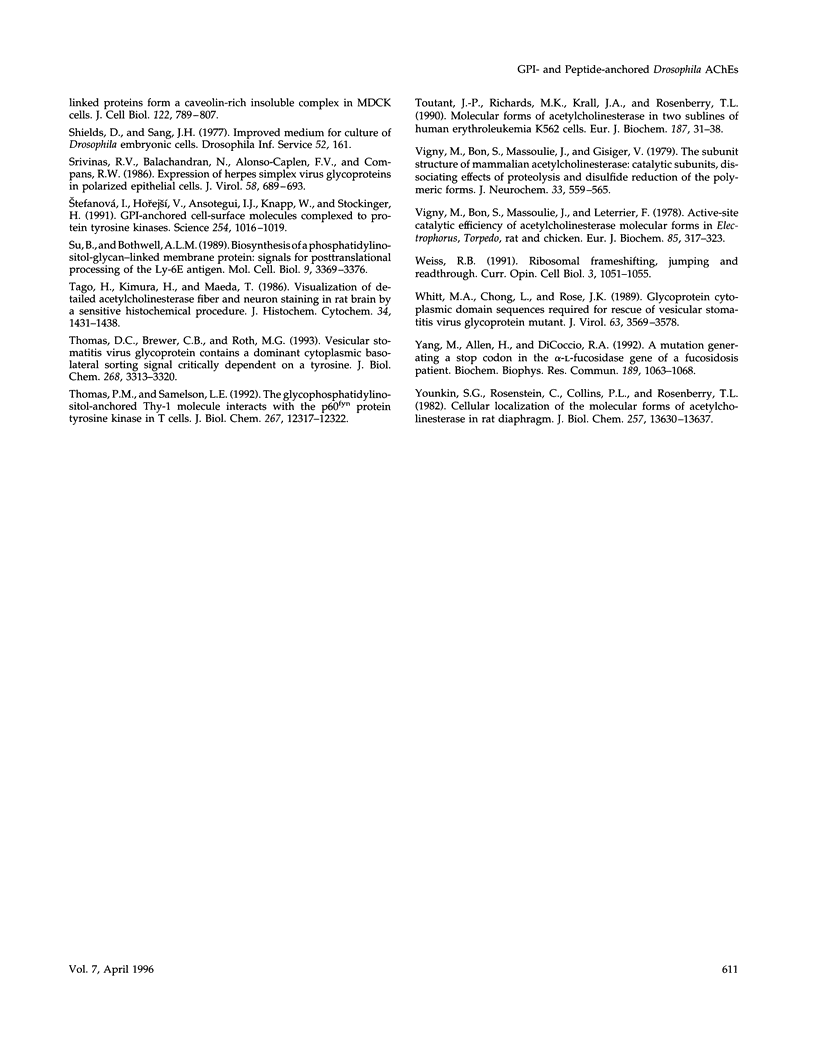
Images in this article
Selected References
These references are in PubMed. This may not be the complete list of references from this article.
- Abrams J. M., Lux A., Steller H., Krieger M. Macrophages in Drosophila embryos and L2 cells exhibit scavenger receptor-mediated endocytosis. Proc Natl Acad Sci U S A. 1992 Nov 1;89(21):10375–10379. doi: 10.1073/pnas.89.21.10375. [DOI] [PMC free article] [PubMed] [Google Scholar]
- Anderson R. G. Plasmalemmal caveolae and GPI-anchored membrane proteins. Curr Opin Cell Biol. 1993 Aug;5(4):647–652. doi: 10.1016/0955-0674(93)90135-d. [DOI] [PubMed] [Google Scholar]
- Berger J., Howard A. D., Brink L., Gerber L., Hauber J., Cullen B. R., Udenfriend S. COOH-terminal requirements for the correct processing of a phosphatidylinositol-glycan anchored membrane protein. J Biol Chem. 1988 Jul 15;263(20):10016–10021. [PubMed] [Google Scholar]
- Berger J., Micanovic R., Greenspan R. J., Udenfriend S. Conversion of placental alkaline phosphatase from a phosphatidylinositol-glycan-anchored protein to an integral transmembrane protein. Proc Natl Acad Sci U S A. 1989 Mar;86(5):1457–1460. doi: 10.1073/pnas.86.5.1457. [DOI] [PMC free article] [PubMed] [Google Scholar]
- Brockman S. K., Przybylski R. J., Younkin S. G. Cellular localization of the molecular forms of acetylcholinesterase in cultured embryonic rat myotubes. J Neurosci. 1982 Dec;2(12):1775–1785. doi: 10.1523/JNEUROSCI.02-12-01775.1982. [DOI] [PMC free article] [PubMed] [Google Scholar]
- Brown D. A., Crise B., Rose J. K. Mechanism of membrane anchoring affects polarized expression of two proteins in MDCK cells. Science. 1989 Sep 29;245(4925):1499–1501. doi: 10.1126/science.2571189. [DOI] [PubMed] [Google Scholar]
- Brown D. A., Rose J. K. Sorting of GPI-anchored proteins to glycolipid-enriched membrane subdomains during transport to the apical cell surface. Cell. 1992 Feb 7;68(3):533–544. doi: 10.1016/0092-8674(92)90189-j. [DOI] [PubMed] [Google Scholar]
- Butters T. D., Hughes R. C., Vischer P. Steps in the biosynthesis of mosquito cell membrane glycoproteins and the effects of tunicamycin. Biochim Biophys Acta. 1981 Feb 6;640(3):672–686. doi: 10.1016/0005-2736(81)90097-3. [DOI] [PubMed] [Google Scholar]
- Caras I. W., Weddell G. N., Williams S. R. Analysis of the signal for attachment of a glycophospholipid membrane anchor. J Cell Biol. 1989 Apr;108(4):1387–1396. doi: 10.1083/jcb.108.4.1387. [DOI] [PMC free article] [PubMed] [Google Scholar]
- Cinek T., Horejsí V. The nature of large noncovalent complexes containing glycosyl-phosphatidylinositol-anchored membrane glycoproteins and protein tyrosine kinases. J Immunol. 1992 Oct 1;149(7):2262–2270. [PubMed] [Google Scholar]
- Crise B., Ruusala A., Zagouras P., Shaw A., Rose J. K. Oligomerization of glycolipid-anchored and soluble forms of the vesicular stomatitis virus glycoprotein. J Virol. 1989 Dec;63(12):5328–5333. doi: 10.1128/jvi.63.12.5328-5333.1989. [DOI] [PMC free article] [PubMed] [Google Scholar]
- Davitz M. A., Hom J., Schenkman S. Purification of a glycosyl-phosphatidylinositol-specific phospholipase D from human plasma. J Biol Chem. 1989 Aug 15;264(23):13760–13764. [PubMed] [Google Scholar]
- Deeg M. A., Humphrey D. R., Yang S. H., Ferguson T. R., Reinhold V. N., Rosenberry T. L. Glycan components in the glycoinositol phospholipid anchor of human erythrocyte acetylcholinesterase. Novel fragments produced by trifluoroacetic acid. J Biol Chem. 1992 Sep 15;267(26):18573–18580. [PubMed] [Google Scholar]
- Dotti C. G., Parton R. G., Simons K. Polarized sorting of glypiated proteins in hippocampal neurons. Nature. 1991 Jan 10;349(6305):158–161. doi: 10.1038/349158a0. [DOI] [PubMed] [Google Scholar]
- Dupree P., Parton R. G., Raposo G., Kurzchalia T. V., Simons K. Caveolae and sorting in the trans-Golgi network of epithelial cells. EMBO J. 1993 Apr;12(4):1597–1605. doi: 10.1002/j.1460-2075.1993.tb05804.x. [DOI] [PMC free article] [PubMed] [Google Scholar]
- ELLMAN G. L., COURTNEY K. D., ANDRES V., Jr, FEATHER-STONE R. M. A new and rapid colorimetric determination of acetylcholinesterase activity. Biochem Pharmacol. 1961 Jul;7:88–95. doi: 10.1016/0006-2952(61)90145-9. [DOI] [PubMed] [Google Scholar]
- Englund P. T. The structure and biosynthesis of glycosyl phosphatidylinositol protein anchors. Annu Rev Biochem. 1993;62:121–138. doi: 10.1146/annurev.bi.62.070193.001005. [DOI] [PubMed] [Google Scholar]
- Fox J. A., Soliz N. M., Saltiel A. R. Purification of a phosphatidylinositol-glycan-specific phospholipase C from liver plasma membranes: a possible target of insulin action. Proc Natl Acad Sci U S A. 1987 May;84(9):2663–2667. doi: 10.1073/pnas.84.9.2663. [DOI] [PMC free article] [PubMed] [Google Scholar]
- Frink R. J., Eisenberg R., Cohen G., Wagner E. K. Detailed analysis of the portion of the herpes simplex virus type 1 genome encoding glycoprotein C. J Virol. 1983 Feb;45(2):634–647. doi: 10.1128/jvi.45.2.634-647.1983. [DOI] [PMC free article] [PubMed] [Google Scholar]
- Gnagey A. L., Forte M., Rosenberry T. L. Isolation and characterization of acetylcholinesterase from Drosophila. J Biol Chem. 1987 Sep 25;262(27):13290–13298. [PubMed] [Google Scholar]
- Gritz L., Davies J. Plasmid-encoded hygromycin B resistance: the sequence of hygromycin B phosphotransferase gene and its expression in Escherichia coli and Saccharomyces cerevisiae. Gene. 1983 Nov;25(2-3):179–188. doi: 10.1016/0378-1119(83)90223-8. [DOI] [PubMed] [Google Scholar]
- Guan J. L., Machamer C. E., Rose J. K. Glycosylation allows cell-surface transport of an anchored secretory protein. Cell. 1985 Sep;42(2):489–496. doi: 10.1016/0092-8674(85)90106-0. [DOI] [PubMed] [Google Scholar]
- Hall L. M., Spierer P. The Ace locus of Drosophila melanogaster: structural gene for acetylcholinesterase with an unusual 5' leader. EMBO J. 1986 Nov;5(11):2949–2954. doi: 10.1002/j.1460-2075.1986.tb04591.x. [DOI] [PMC free article] [PubMed] [Google Scholar]
- Hereld D., Krakow J. L., Bangs J. D., Hart G. W., Englund P. T. A phospholipase C from Trypanosoma brucei which selectively cleaves the glycolipid on the variant surface glycoprotein. J Biol Chem. 1986 Oct 15;261(29):13813–13819. [PubMed] [Google Scholar]
- Hoener M. C., Stieger S., Brodbeck U. Isolation and characterization of a phosphatidylinositol-glycan-anchor-specific phospholipase D from bovine brain. Eur J Biochem. 1990 Jul 5;190(3):593–601. doi: 10.1111/j.1432-1033.1990.tb15614.x. [DOI] [PubMed] [Google Scholar]
- Holland T. C., Lerch R. J., Earhart K. The cytoplasmic domain of herpes simplex virus type 1 glycoprotein C is required for membrane anchoring. J Virol. 1988 May;62(5):1753–1761. doi: 10.1128/jvi.62.5.1753-1761.1988. [DOI] [PMC free article] [PubMed] [Google Scholar]
- Homa F. L., Purifoy D. J., Glorioso J. C., Levine M. Molecular basis of the glycoprotein C-negative phenotypes of herpes simplex virus type 1 mutants selected with a virus-neutralizing monoclonal antibody. J Virol. 1986 May;58(2):281–289. doi: 10.1128/jvi.58.2.281-289.1986. [DOI] [PMC free article] [PubMed] [Google Scholar]
- Hsieh P., Robbins P. W. Regulation of asparagine-linked oligosaccharide processing. Oligosaccharide processing in Aedes albopictus mosquito cells. J Biol Chem. 1984 Feb 25;259(4):2375–2382. [PubMed] [Google Scholar]
- Incardona J. P., Rosenberry T. L. Replacement of the glycoinositol phospholipid anchor of Drosophila acetylcholinesterase with a transmembrane domain does not alter sorting in neurons and epithelia but results in behavioral defects. Mol Biol Cell. 1996 Apr;7(4):613–630. doi: 10.1091/mbc.7.4.613. [DOI] [PMC free article] [PubMed] [Google Scholar]
- Jaeger J. A., Turner D. H., Zuker M. Improved predictions of secondary structures for RNA. Proc Natl Acad Sci U S A. 1989 Oct;86(20):7706–7710. doi: 10.1073/pnas.86.20.7706. [DOI] [PMC free article] [PubMed] [Google Scholar]
- KARNOVSKY M. J., ROOTS L. A "DIRECT-COLORING" THIOCHOLINE METHOD FOR CHOLINESTERASES. J Histochem Cytochem. 1964 Mar;12:219–221. doi: 10.1177/12.3.219. [DOI] [PubMed] [Google Scholar]
- Kemble G. W., Henis Y. I., White J. M. GPI- and transmembrane-anchored influenza hemagglutinin differ in structure and receptor binding activity. J Cell Biol. 1993 Sep;122(6):1253–1265. doi: 10.1083/jcb.122.6.1253. [DOI] [PMC free article] [PubMed] [Google Scholar]
- Kikuchi G. E., Glorioso J. C., Nairn R. Cross-linking studies show that herpes simplex virus type 1 glycoprotein C molecules are clustered in the membrane of infected cells. J Gen Virol. 1990 Feb;71(Pt 2):455–458. doi: 10.1099/0022-1317-71-2-455. [DOI] [PubMed] [Google Scholar]
- Krasnow M. A., Saffman E. E., Kornfeld K., Hogness D. S. Transcriptional activation and repression by Ultrabithorax proteins in cultured Drosophila cells. Cell. 1989 Jun 16;57(6):1031–1043. doi: 10.1016/0092-8674(89)90341-3. [DOI] [PubMed] [Google Scholar]
- Kuroda K., Geyer H., Geyer R., Doerfler W., Klenk H. D. The oligosaccharides of influenza virus hemagglutinin expressed in insect cells by a baculovirus vector. Virology. 1990 Feb;174(2):418–429. doi: 10.1016/0042-6822(90)90095-9. [DOI] [PubMed] [Google Scholar]
- Laemmli U. K. Cleavage of structural proteins during the assembly of the head of bacteriophage T4. Nature. 1970 Aug 15;227(5259):680–685. doi: 10.1038/227680a0. [DOI] [PubMed] [Google Scholar]
- Lisanti M. P., Caras I. W., Davitz M. A., Rodriguez-Boulan E. A glycophospholipid membrane anchor acts as an apical targeting signal in polarized epithelial cells. J Cell Biol. 1989 Nov;109(5):2145–2156. doi: 10.1083/jcb.109.5.2145. [DOI] [PMC free article] [PubMed] [Google Scholar]
- Low M. G., Prasad A. R. A phospholipase D specific for the phosphatidylinositol anchor of cell-surface proteins is abundant in plasma. Proc Natl Acad Sci U S A. 1988 Feb;85(4):980–984. doi: 10.1073/pnas.85.4.980. [DOI] [PMC free article] [PubMed] [Google Scholar]
- MORGAN C., ROSE H. M., HOLDEN M., JONES E. P. Electron microscopic observations on the development of herpes simplex virus. J Exp Med. 1959 Oct 1;110:643–656. doi: 10.1084/jem.110.4.643. [DOI] [PMC free article] [PubMed] [Google Scholar]
- Mutero A., Fournier D. Post-translational modifications of Drosophila acetylcholinesterase. In vitro mutagenesis and expression in Xenopus oocytes. J Biol Chem. 1992 Jan 25;267(3):1695–1700. [PubMed] [Google Scholar]
- Peltola M., Chiatayat D., Peltonen L., Jalanko A. Characterization of a point mutation in aspartylglucosaminidase gene: evidence for a readthrough of a translational stop codon. Hum Mol Genet. 1994 Dec;3(12):2237–2242. doi: 10.1093/hmg/3.12.2237. [DOI] [PubMed] [Google Scholar]
- Robinson P. J., Millrain M., Antoniou J., Simpson E., Mellor A. L. A glycophospholipid anchor is required for Qa-2-mediated T cell activation. Nature. 1989 Nov 2;342(6245):85–87. doi: 10.1038/342085a0. [DOI] [PubMed] [Google Scholar]
- Robinson P. J. Phosphatidylinositol membrane anchors and T-cell activation. Immunol Today. 1991 Jan;12(1):35–41. doi: 10.1016/0167-5699(91)90110-F. [DOI] [PubMed] [Google Scholar]
- Rosenberry T. L., Scoggin D. M. Structure of human erythrocyte acetylcholinesterase. Characterization of intersubunit disulfide bonding and detergent interaction. J Biol Chem. 1984 May 10;259(9):5643–5652. [PubMed] [Google Scholar]
- Rotundo R. L. Biogenesis of acetylcholinesterase molecular forms in muscle. Evidence for a rapidly turning over, catalytically inactive precursor pool. J Biol Chem. 1988 Dec 25;263(36):19398–19406. [PubMed] [Google Scholar]
- Sargiacomo M., Sudol M., Tang Z., Lisanti M. P. Signal transducing molecules and glycosyl-phosphatidylinositol-linked proteins form a caveolin-rich insoluble complex in MDCK cells. J Cell Biol. 1993 Aug;122(4):789–807. doi: 10.1083/jcb.122.4.789. [DOI] [PMC free article] [PubMed] [Google Scholar]
- Srinivas R. V., Balachandran N., Alonso-Caplen F. V., Compans R. W. Expression of herpes simplex virus glycoproteins in polarized epithelial cells. J Virol. 1986 May;58(2):689–693. doi: 10.1128/jvi.58.2.689-693.1986. [DOI] [PMC free article] [PubMed] [Google Scholar]
- Stefanová I., Horejsí V., Ansotegui I. J., Knapp W., Stockinger H. GPI-anchored cell-surface molecules complexed to protein tyrosine kinases. Science. 1991 Nov 15;254(5034):1016–1019. doi: 10.1126/science.1719635. [DOI] [PubMed] [Google Scholar]
- Su B., Bothwell A. L. Biosynthesis of a phosphatidylinositol-glycan-linked membrane protein: signals for posttranslational processing of the Ly-6E antigen. Mol Cell Biol. 1989 Aug;9(8):3369–3376. doi: 10.1128/mcb.9.8.3369. [DOI] [PMC free article] [PubMed] [Google Scholar]
- Tago H., Kimura H., Maeda T. Visualization of detailed acetylcholinesterase fiber and neuron staining in rat brain by a sensitive histochemical procedure. J Histochem Cytochem. 1986 Nov;34(11):1431–1438. doi: 10.1177/34.11.2430009. [DOI] [PubMed] [Google Scholar]
- Thomas D. C., Brewer C. B., Roth M. G. Vesicular stomatitis virus glycoprotein contains a dominant cytoplasmic basolateral sorting signal critically dependent upon a tyrosine. J Biol Chem. 1993 Feb 15;268(5):3313–3320. [PubMed] [Google Scholar]
- Thomas P. M., Samelson L. E. The glycophosphatidylinositol-anchored Thy-1 molecule interacts with the p60fyn protein tyrosine kinase in T cells. J Biol Chem. 1992 Jun 15;267(17):12317–12322. [PubMed] [Google Scholar]
- Toutant J. P., Richards M. K., Krall J. A., Rosenberry T. L. Molecular forms of acetylcholinesterase in two sublines of human erythroleukemia K562 cells. Sensitivity or resistance to phosphatidylinositol-specific phospholipase C and biosynthesis. Eur J Biochem. 1990 Jan 12;187(1):31–38. doi: 10.1111/j.1432-1033.1990.tb15274.x. [DOI] [PubMed] [Google Scholar]
- Vigny M., Bon S., Massoulié J., Gisiger V. The subunit structure of mammalian acetylcholinesterase: catalytic subunits, dissociating effect of proteolysis and disulphide reduction on the polymeric forms. J Neurochem. 1979 Aug;33(2):559–562. doi: 10.1111/j.1471-4159.1979.tb05188.x. [DOI] [PubMed] [Google Scholar]
- Vigny M., Bon S., Massoulié J., Leterrier F. Active-site catalytic efficiency of acetylcholinesterase molecular forms in Electrophorus, torpedo, rat and chicken. Eur J Biochem. 1978 Apr 17;85(2):317–323. doi: 10.1111/j.1432-1033.1978.tb12241.x. [DOI] [PubMed] [Google Scholar]
- Weiss R. B. Ribosomal frameshifting, jumping and readthrough. Curr Opin Cell Biol. 1991 Dec;3(6):1051–1055. doi: 10.1016/0955-0674(91)90128-L. [DOI] [PMC free article] [PubMed] [Google Scholar]
- Whitt M. A., Chong L., Rose J. K. Glycoprotein cytoplasmic domain sequences required for rescue of a vesicular stomatitis virus glycoprotein mutant. J Virol. 1989 Sep;63(9):3569–3578. doi: 10.1128/jvi.63.9.3569-3578.1989. [DOI] [PMC free article] [PubMed] [Google Scholar]
- Yang M., Allen H., DiCioccio R. A. A mutation generating a stop codon in the alpha-L-fucosidase gene of a fucosidosis patient. Biochem Biophys Res Commun. 1992 Dec 15;189(2):1063–1068. doi: 10.1016/0006-291x(92)92312-l. [DOI] [PubMed] [Google Scholar]
- Younkin S. G., Rosenstein C., Collins P. L., Rosenberry T. L. Cellular localization of the molecular forms of acetylcholinesterase in rat diaphragm. J Biol Chem. 1982 Nov 25;257(22):13630–13637. [PubMed] [Google Scholar]