Abstract
The feedback inhibition of glutamine synthetase was investigated by use of partially purified enzyme preparations from Salmonella typhimurium, Micrococcus sodonensis, Pseudomonas fluorescens, Bacillus cereus, Bacillus licheniformis, Clostridium pasteurianum, Rhodospirillum rubrum, Neurospora crassa, Candida utilis, and Chlorella pyrenoidosa. Inhibition analyses indicated that the enzyme of each organism can be effectively regulated with mixtures of end products from the diverse pathways of glutamine metabolism. When tested individually, tryptophan, histidine, alanine, glycine, glutamine, 5′-adenylate (AMP), cytidine-5′-triphosphate, carbamyl phosphate, and glucosamine-6-phosphate gave limited inhibition. In most cases, the inhibitors were independent in their action, and cumulative degrees of inhibition were obtained with mixtures of these end products. In contrast, with the glutamine synthetases of the two Bacillus species, the simultaneous presence of AMP and histidine (or AMP and glutamine) gave inhibition greater than the sum of the amounts of inhibition caused by either inhibitor alone. Also, alanine and carbamyl phosphate acted synergistically to inhibit the enzyme from N. crassa. The remarkable similarity in the overall patterns of end-product inhibition observed with the enzymes from different sources indicates that these diverse organisms have evolved comparable mechanisms for the regulation of glutamine metabolism. Nevertheless, the enzymes from different sources do differ significantly in their physical and catalytic properties, as was demonstrated by dissimilarities in their purification behaviors, specificity for nucleotide substrate, ability to catalyze the glutamyl transfer reaction, and ability to utilize Mn++ and Mg++ as activators for the biosynthetic reaction.
Full text
PDF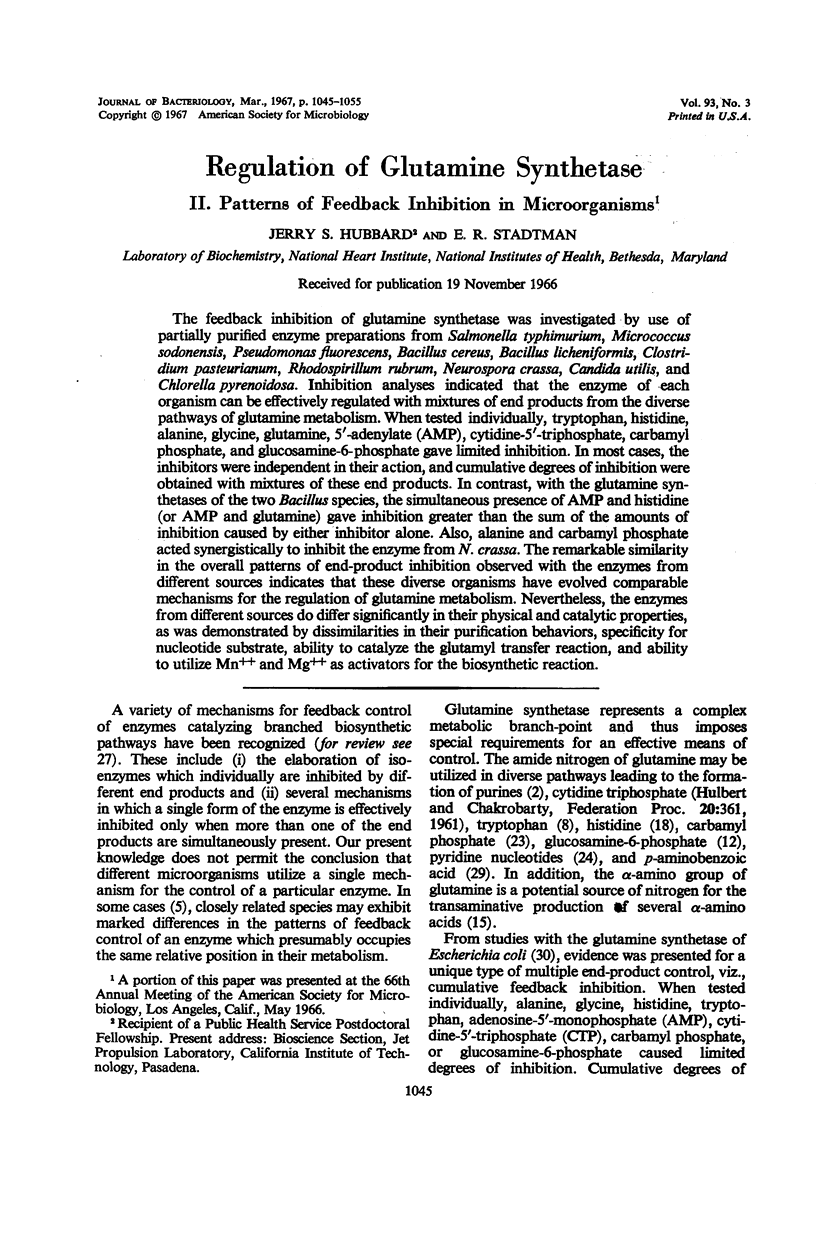
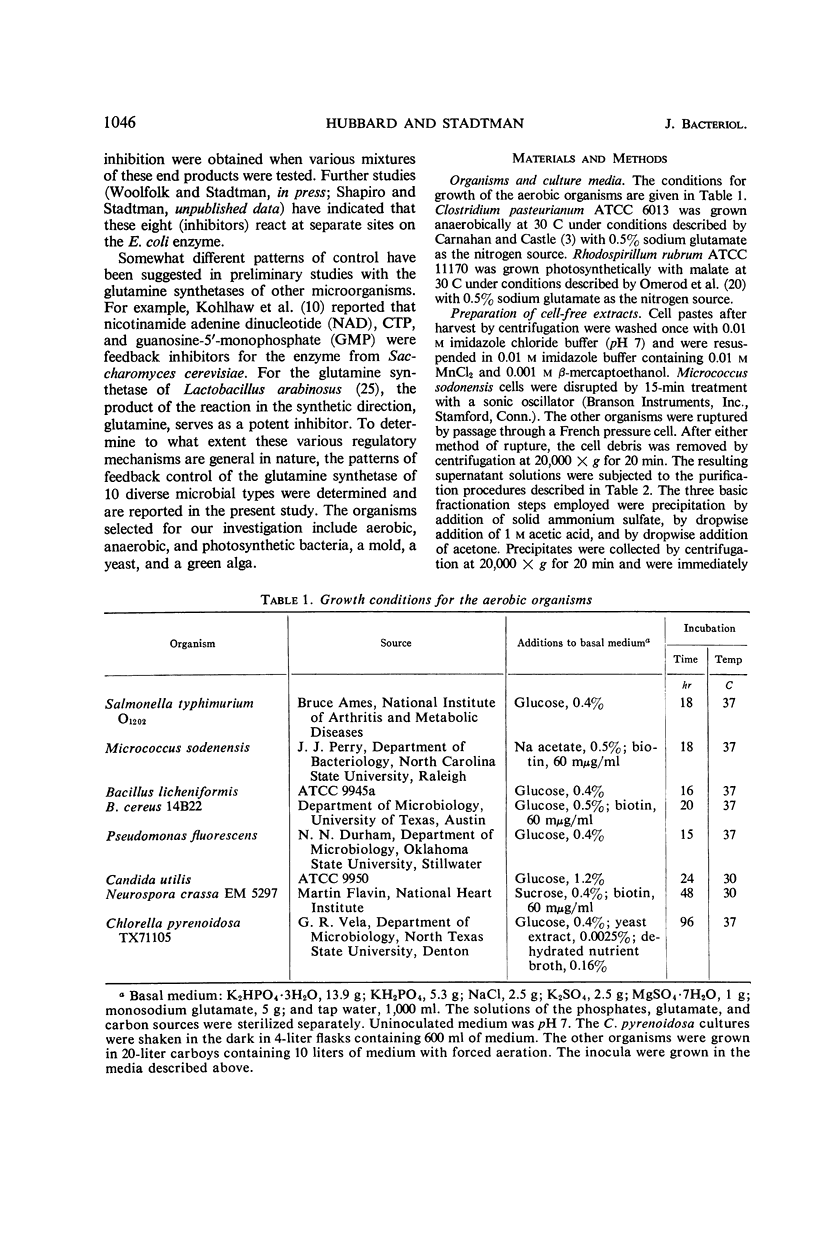
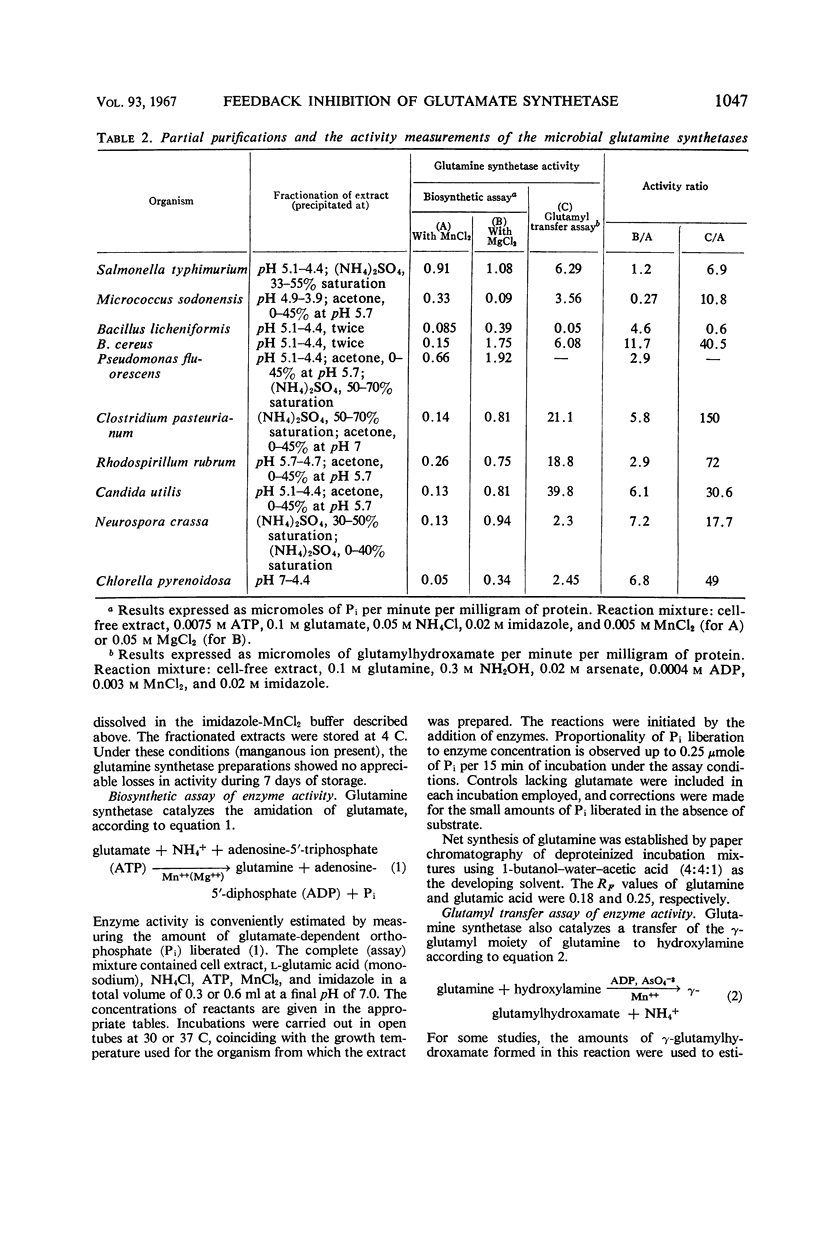
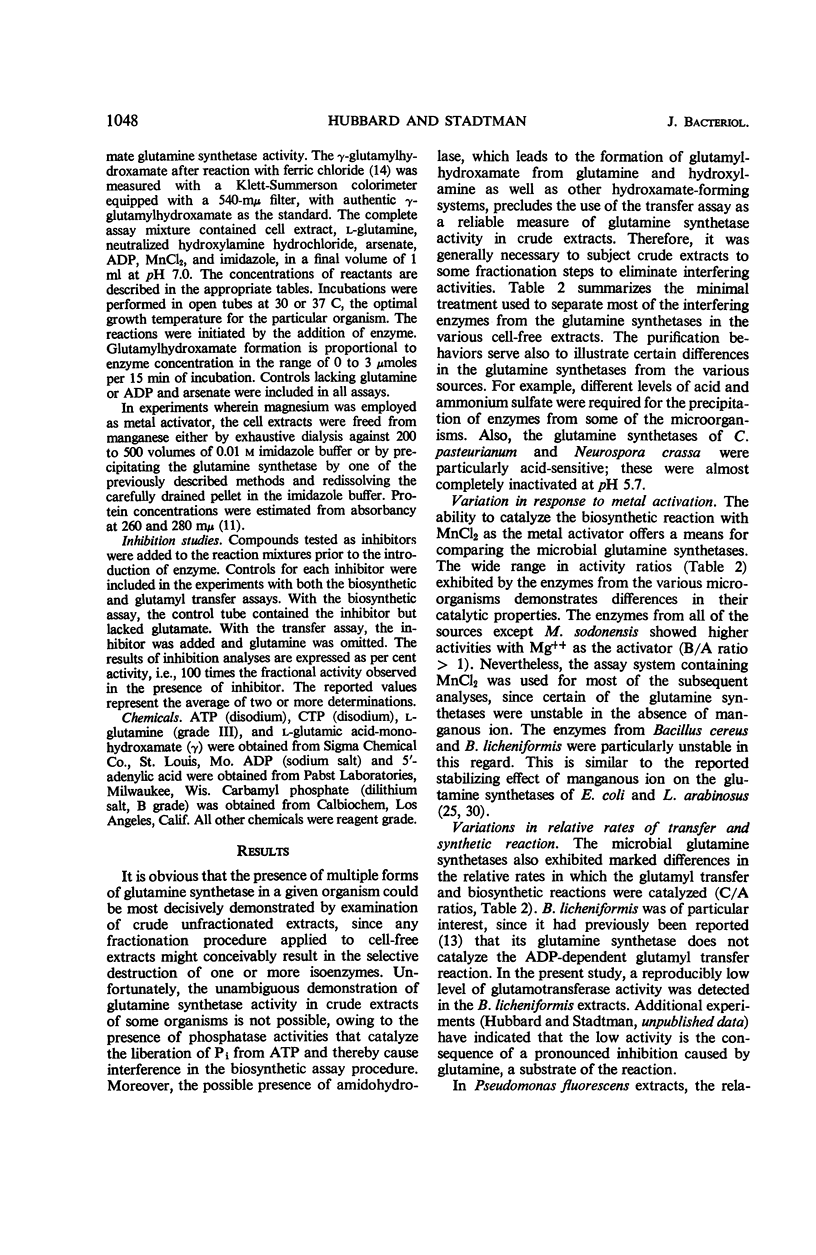
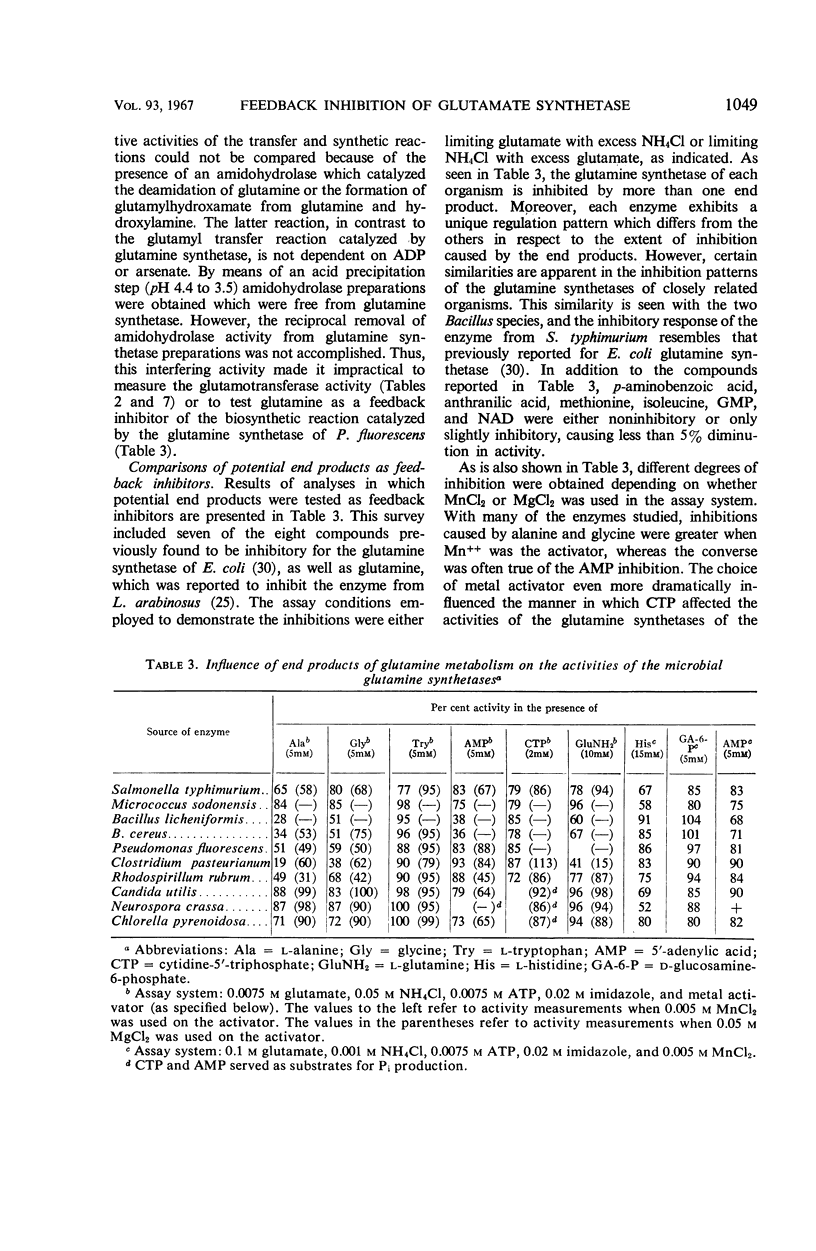
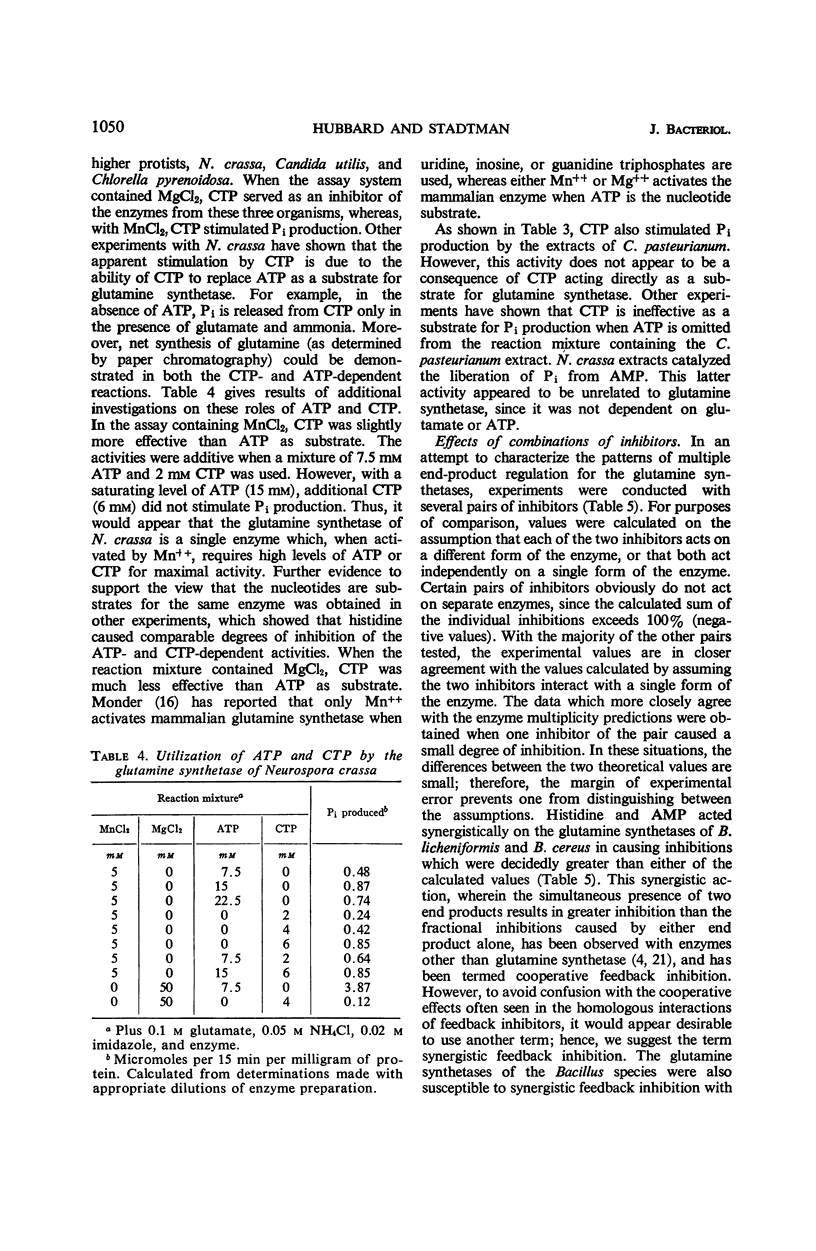
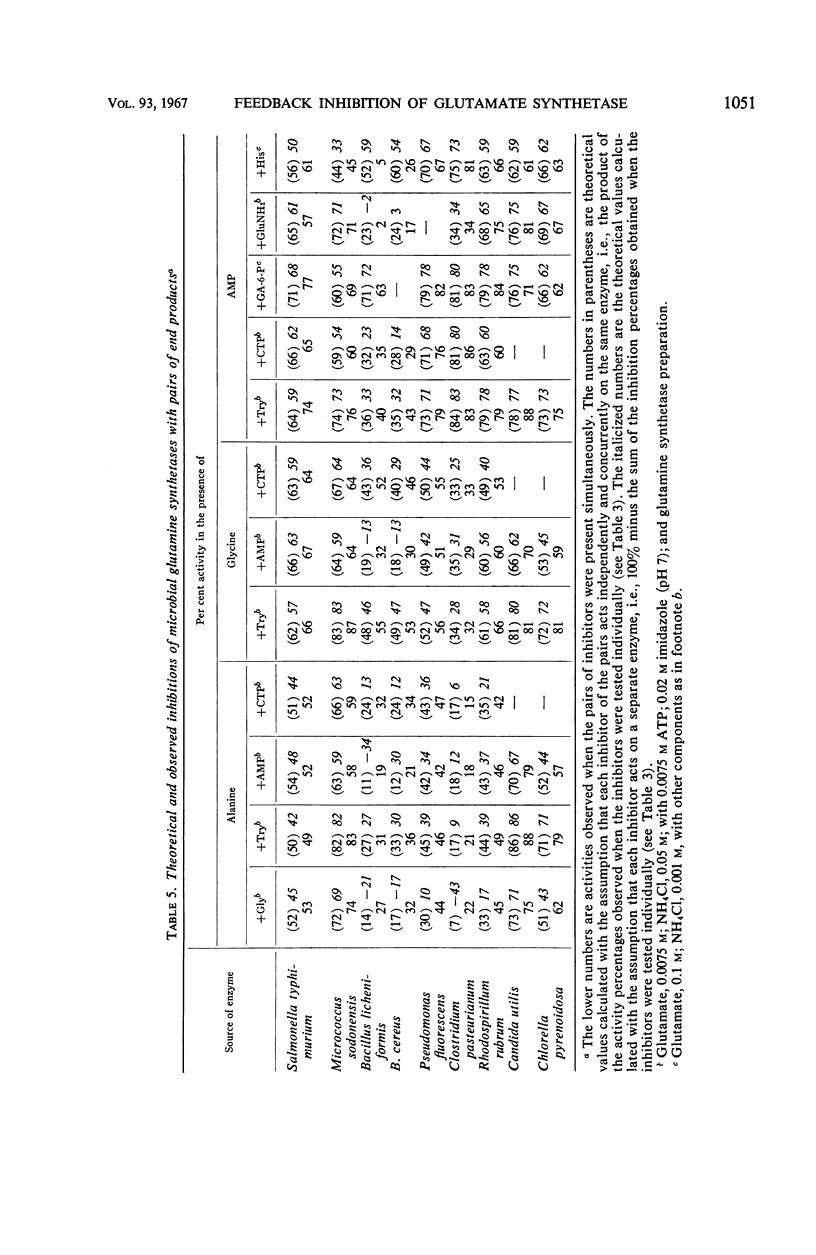
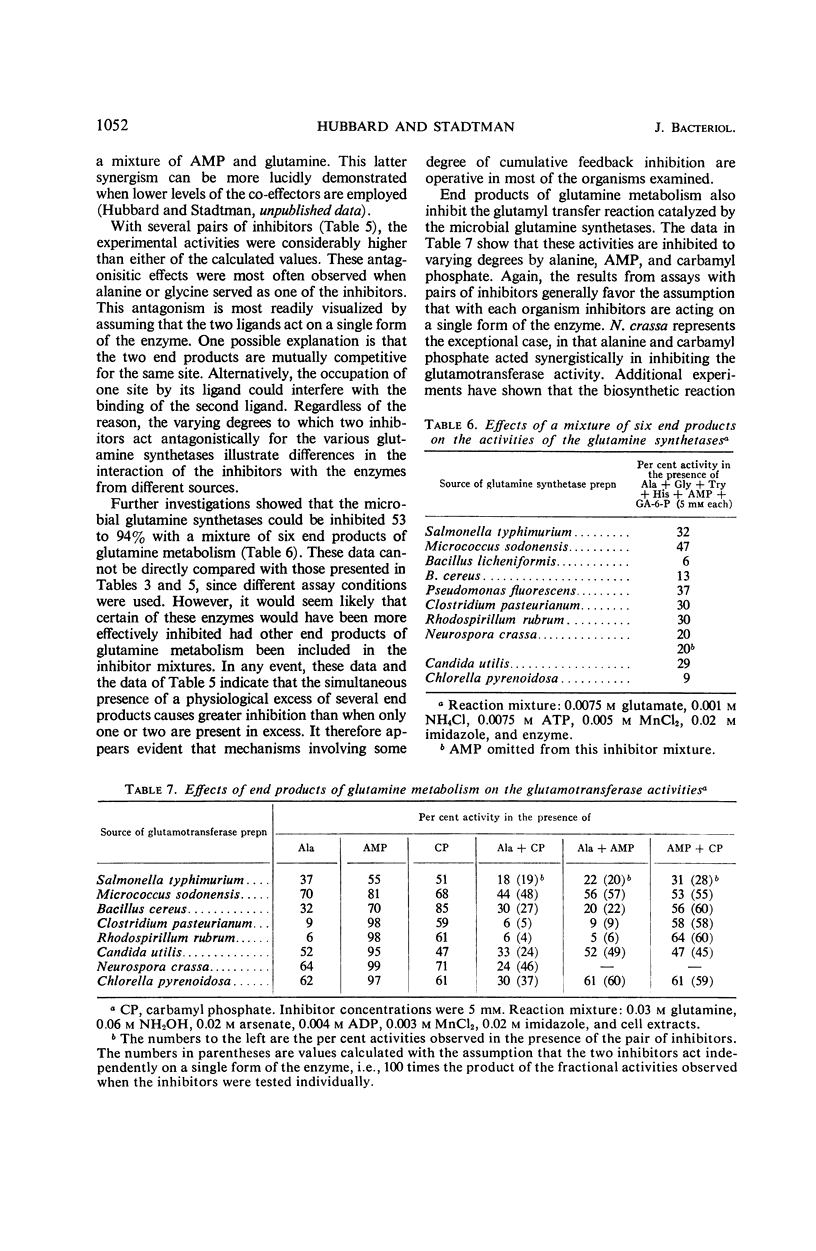
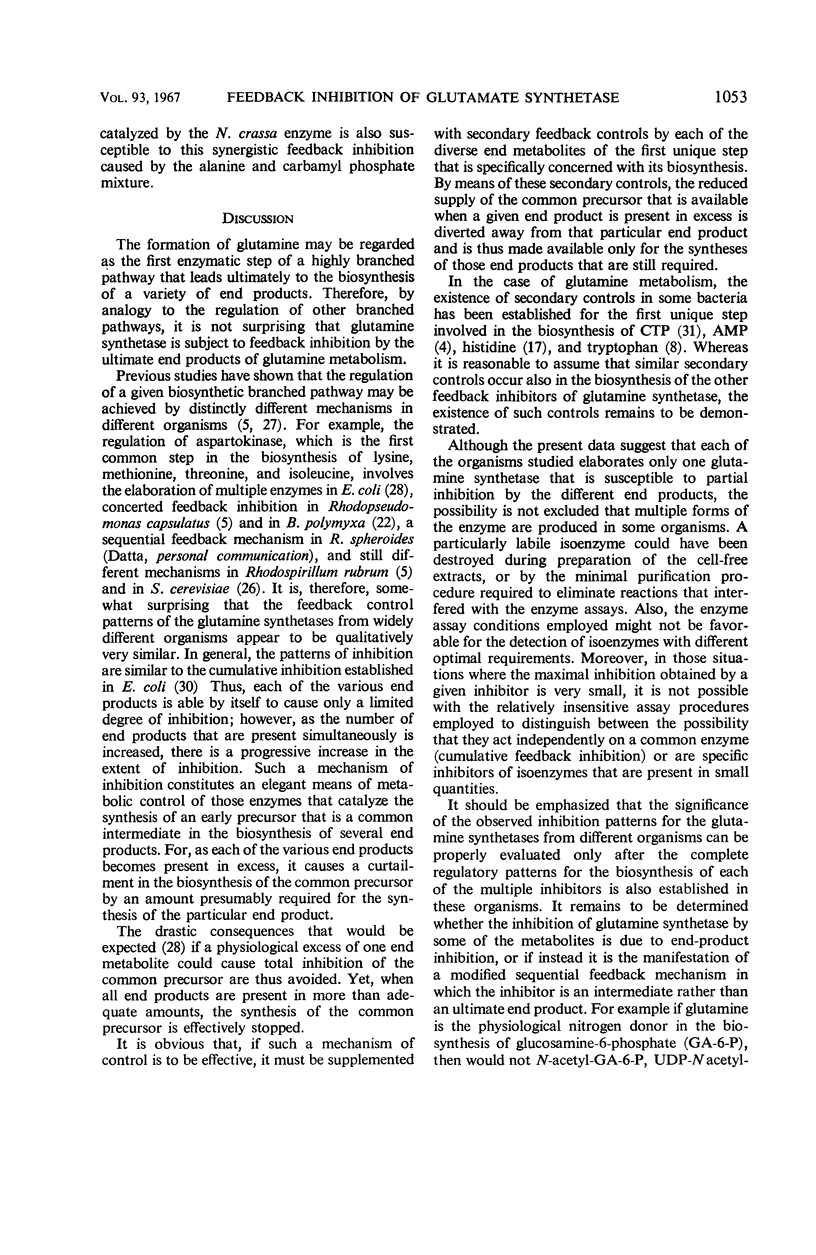
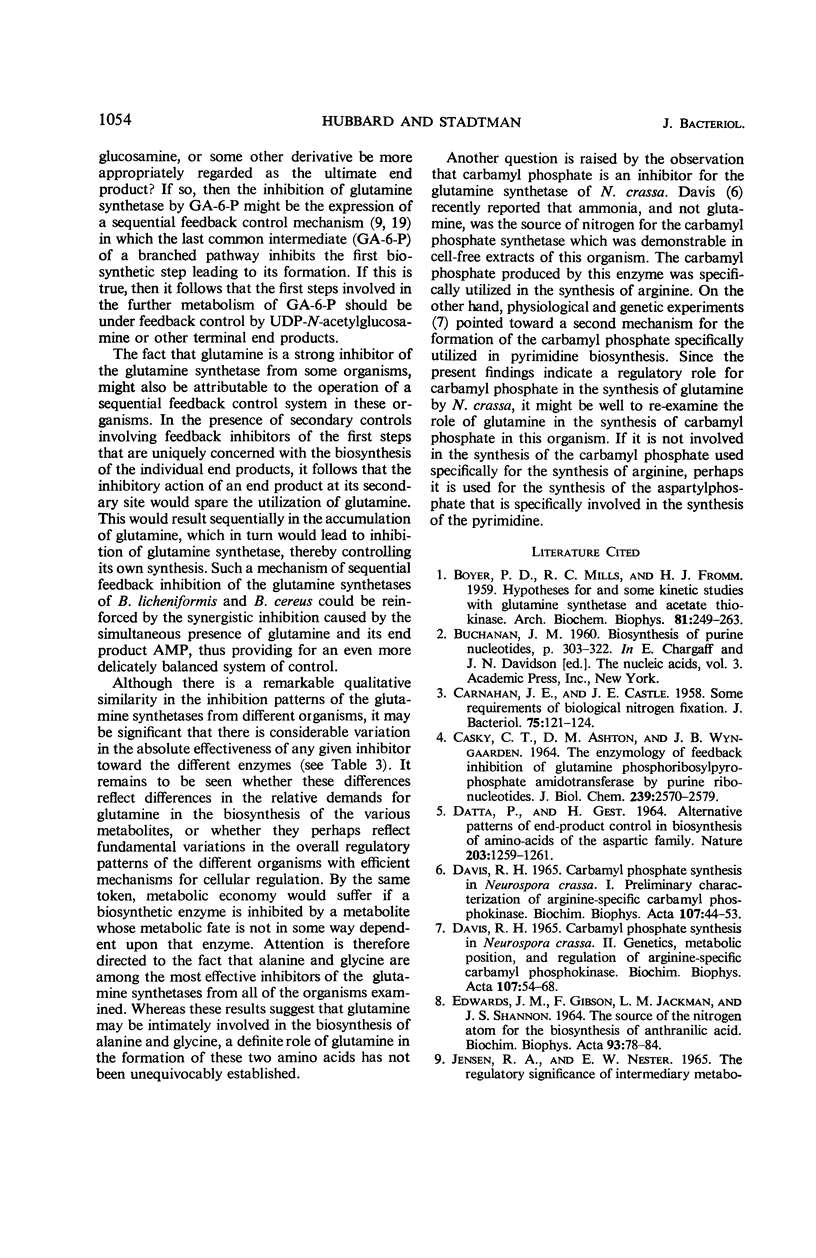
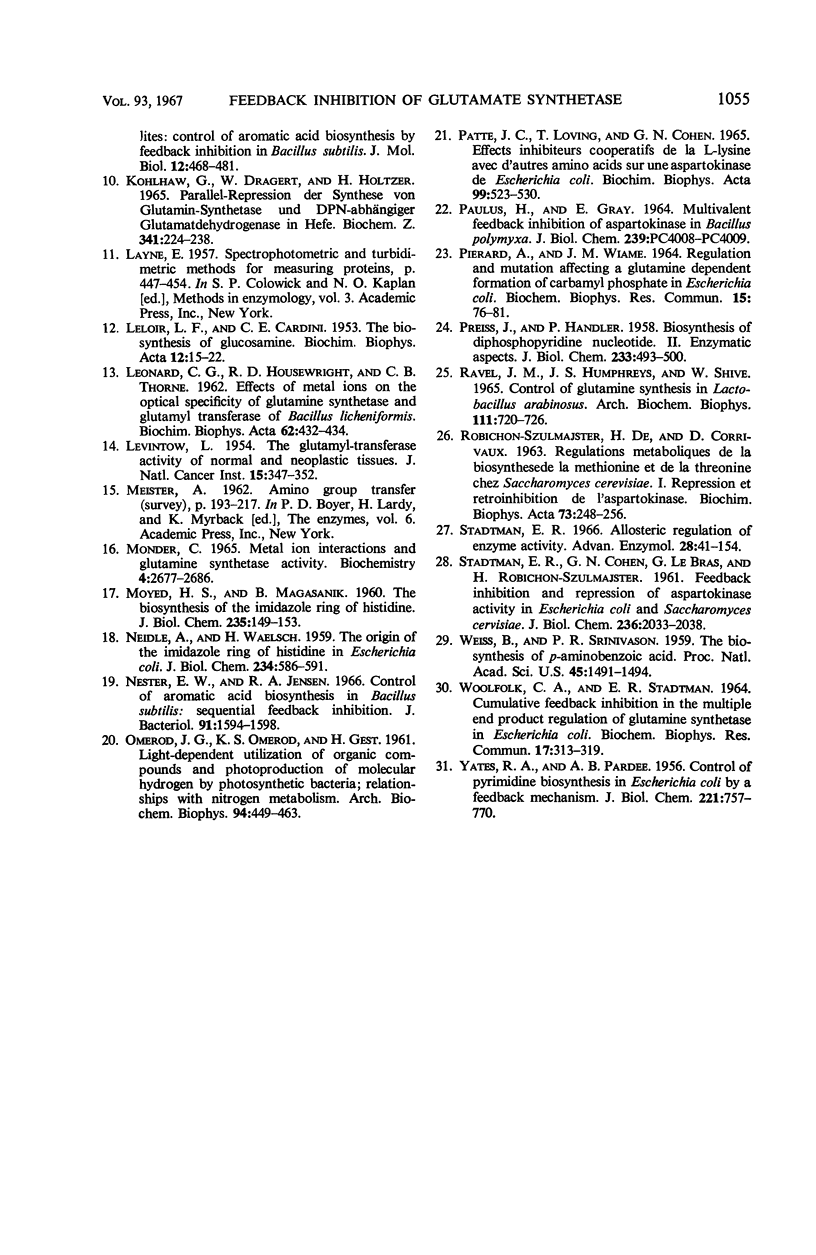
Selected References
These references are in PubMed. This may not be the complete list of references from this article.
- BOYER P. D., MILLS R. C., FROMM H. J. Hypotheses for and some kinetic studies with glutamine synthetase and acetate thiokinase. Arch Biochem Biophys. 1959 Mar;81(1):249–263. doi: 10.1016/0003-9861(59)90194-8. [DOI] [PubMed] [Google Scholar]
- CARNAHAN J. E., CASTLE J. E. Some requirements of biological nitrogen fixation. J Bacteriol. 1958 Feb;75(2):121–124. doi: 10.1128/jb.75.2.121-124.1958. [DOI] [PMC free article] [PubMed] [Google Scholar]
- DATTA P., GEST H. ALTERNATIVE PATTERNS OF END-PRODUCT CONTROL IN BIOSYNTHESIS OF AMINO-ACIDS OF THE ASPARTIC FAMILY. Nature. 1964 Sep 19;203:1259–1261. doi: 10.1038/2031259a0. [DOI] [PubMed] [Google Scholar]
- Davis R. H. Carbamyl phosphate synthesis in Neurospora crassa. I. Preliminary characterization of arginine-specific carbamyl phosphokinase. Biochim Biophys Acta. 1965 Aug 24;107(1):44–53. doi: 10.1016/0304-4165(65)90387-9. [DOI] [PubMed] [Google Scholar]
- Davis R. H. Carbamyl phosphate synthesis in Neurospora crassa. II. Genetics, metabolic position, and regulation of arginine-specific carbamyl phosphokinase. Biochim Biophys Acta. 1965 Aug 24;107(1):54–68. doi: 10.1016/0304-4165(65)90388-0. [DOI] [PubMed] [Google Scholar]
- EDWARDS J. M., GIBSON F., JACKMAN L. M., SHANNON J. S. THE SOURCE OF THE NITROGEN ATOM FOR THE BIOSYNTHESIS OF ANTHRANILIC ACID. Biochim Biophys Acta. 1964 Oct 9;93:78–84. doi: 10.1016/0304-4165(64)90262-4. [DOI] [PubMed] [Google Scholar]
- JENSEN R. A., NESTER E. W. THE REGULATORY SIGNIFICANCE OF INTERMEDIARY METABOLITES: CONTROL OF AROMATIC ACID BIOSYNTHESIS BY FEEDBACK INHIBITION IN BACILLUS SUBTILIS. J Mol Biol. 1965 Jun;12:468–481. doi: 10.1016/s0022-2836(65)80270-4. [DOI] [PubMed] [Google Scholar]
- LELOIR L. F., CARDINI C. E. The biosynthesis of glucosamine. Biochim Biophys Acta. 1953 Sep-Oct;12(1-2):15–22. doi: 10.1016/0006-3002(53)90119-x. [DOI] [PubMed] [Google Scholar]
- LEONARD C. G., HOUSEWRIGHT R. D., THORNE C. B. Effects of metal ions on the optical specificity of glutamine synthetase and glutamyl transferase of Bacillus licheniformis. Biochim Biophys Acta. 1962 Aug 13;62:432–434. doi: 10.1016/0006-3002(62)90278-0. [DOI] [PubMed] [Google Scholar]
- LEVINTOW L. The glutamyltransferase activity of normal and neoplastic tissues. J Natl Cancer Inst. 1954 Oct;15(2):347–352. [PubMed] [Google Scholar]
- MOYED H. S., MAGASANIK B. The biosynthesis of the imidazole ring of histidine. J Biol Chem. 1960 Jan;235:149–153. [PubMed] [Google Scholar]
- Monder C. Metal ion interactions and glutamine synthetase activity. Biochemistry. 1965 Dec;4(12):2677–2686. doi: 10.1021/bi00888a017. [DOI] [PubMed] [Google Scholar]
- NEIDLE A., WAELSCH H. The origin of the imidazole ring of histidine in Escherichia coli. J Biol Chem. 1959 Mar;234(3):586–591. [PubMed] [Google Scholar]
- Nester E. W., Jensen R. A. Control of aromatic acid biosynthesis in Bacillus subtilis: sequenial feedback inhibition. J Bacteriol. 1966 Apr;91(4):1594–1598. doi: 10.1128/jb.91.4.1594-1598.1966. [DOI] [PMC free article] [PubMed] [Google Scholar]
- ORMEROD J. G., ORMEROD K. S., GEST H. Light-dependent utilization of organic compounds and photoproduction of molecular hydrogen by photosynthetic bacteria; relationships with nitrogen metabolism. Arch Biochem Biophys. 1961 Sep;94:449–463. doi: 10.1016/0003-9861(61)90073-x. [DOI] [PubMed] [Google Scholar]
- PARDEE A. B., YATES R. A. Control of pyrimidine biosynthesis in Escherichia coli by a feed-back mechanism. J Biol Chem. 1956 Aug;221(2):757–770. [PubMed] [Google Scholar]
- PAULUS H., GRAY E. MULTIVALENT FEEDBACK INHIBITION OF ASPARTOKINASE IN BACILLUS POLYMYXA. J Biol Chem. 1964 Nov;239:PC4008–PC4009. [PubMed] [Google Scholar]
- PREISS J., HANDLER P. Biosynthesis of diphosphopyridine nucleotide. II. Enzymatic aspects. J Biol Chem. 1958 Aug;233(2):493–500. [PubMed] [Google Scholar]
- Patte J. C., Loviny T., Cohen G. N. Effets inhibiteurs cooperatifs de la L-lysine avec d'autres amino acides sur une aspartokinase de Escherichia coli. Biochim Biophys Acta. 1965 Jun 22;99(3):523–530. [PubMed] [Google Scholar]
- Piérard A., Wiame J. M. Regulation and mutation affecting a glutamine dependent formation of carbamyl phosphate in Escherichia coli. Biochem Biophys Res Commun. 1964 Feb 18;15(1):76–81. doi: 10.1016/0006-291x(64)90106-8. [DOI] [PubMed] [Google Scholar]
- Ravel J. M., Humphreys J. S., Shive W. Control of glutamine synthesis in Lactobacillus arabinosus. Arch Biochem Biophys. 1965 Sep;111(3):720–726. doi: 10.1016/0003-9861(65)90255-9. [DOI] [PubMed] [Google Scholar]
- Stadtman E. R. Allosteric regulation of enzyme activity. Adv Enzymol Relat Areas Mol Biol. 1966;28:41–154. doi: 10.1002/9780470122730.ch2. [DOI] [PubMed] [Google Scholar]
- Weiss B., Srinivasan P. R. THE BIOSYNTHESIS OF p-AMINOBENZOIC ACID. Proc Natl Acad Sci U S A. 1959 Oct;45(10):1491–1494. doi: 10.1073/pnas.45.10.1491. [DOI] [PMC free article] [PubMed] [Google Scholar]