Abstract
Ling, Chung-Mei (Illinois Institute of Technology, Chicago), and L. R. Hedrick. Proline oxidases in Hansenula subpelliculosa. J. Bacteriol. 87:1462–1470. 1964—Cells of Hansenula subpelliculosa can use l-proline as a carbon and a nitrogen source after a 6- to 8-hr induction period. However, they cannot use l-glutamate as both nitrogen and carbon sources unless the induction period is of several days' duration. Two l-proline oxidases were demonstrated in the mitochondrial preparation of this yeast. One forms the product Δ′-pyrroline-2-carboxylic acid (P2C), which is in equilibrium with α-keto-δ-amino-valeric acid; the other forms the product Δ′-pyrroline-5-carboxylic acid (P5C), which is in equilibrium with glutamic-γ-semialdehyde. The first-mentioned enzyme is induced when l-proline is the carbon source; the second appears to be constitutive, and is probably associated with the use of l-proline as a nitrogen source. The P2C-forming enzyme is specific for the l isomer of proline, and is inactive against l-hydroxyproline. The enzyme activity is at its peak when the mitochondria are prepared from logarithmically grown cells, and is rapidly reduced after cells reach the stationary phase of growth. Kinetic studies with varying concentrations of substrate indicate a Michaelis-Menten constant of 2.45 × 10−2m. Paper chromatographic studies, chemical tests with H2O2, sensitivity to freezing, and spectral measurements indicate that proline oxidase from H. subpelliculosa mitochondria forms a product from l-proline which is like, if not identical to, P2C formed by the action of sheep kidney d-proline oxidase upon dl-proline. The soluble portion of the cell extract contains NAD+ enzymes which use either P2C (α-keto-δ-amino-valeric acid) or P5C (glutamic-γ-semialdehyde) as substrates. No glutamic dehydrogenase activity could be detected when l-glutamic acid and the nicotinamide adenine dinucleotide (NAD+) cofactor were added to the supernatant solution with the yeast enzymes. The presence of a dehydrogenase NAD+ enzyme for activity with P2C (α-keto-δ-amino-valeric acid) has not been previously reported.
Full text
PDF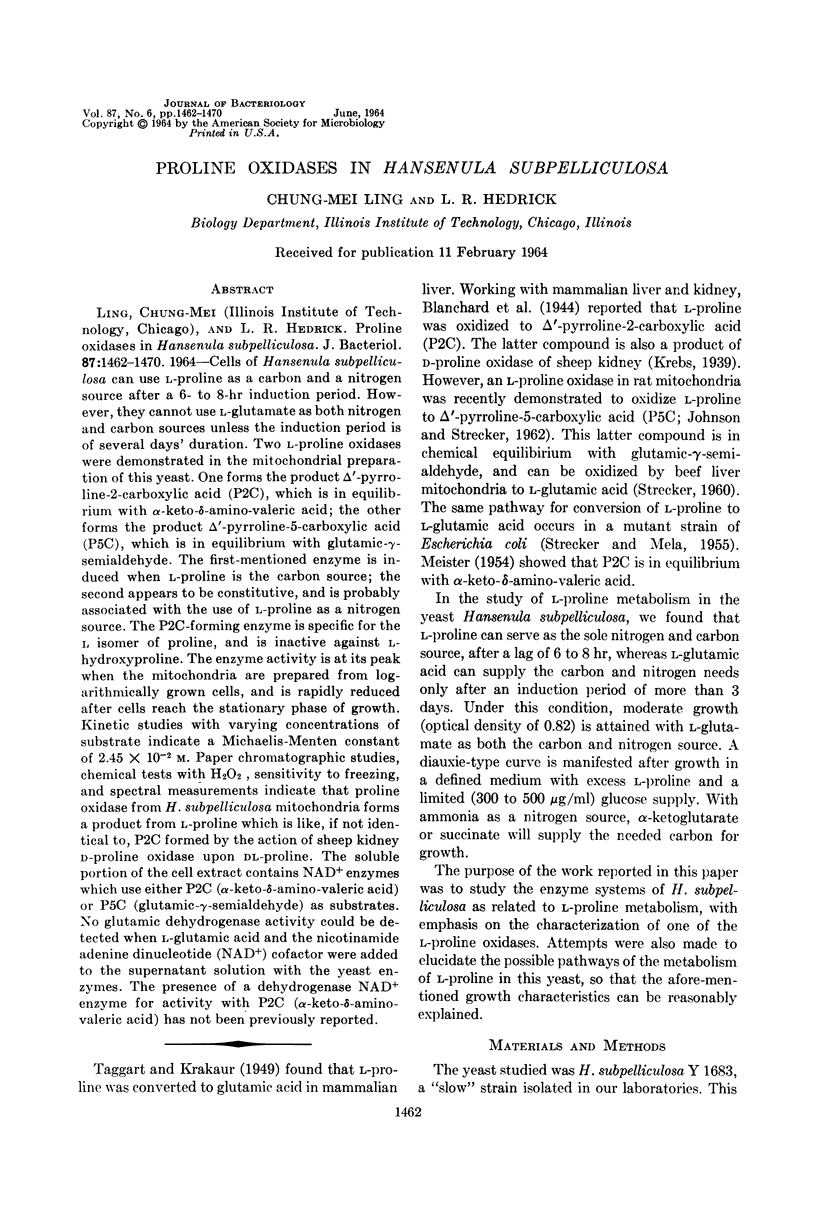
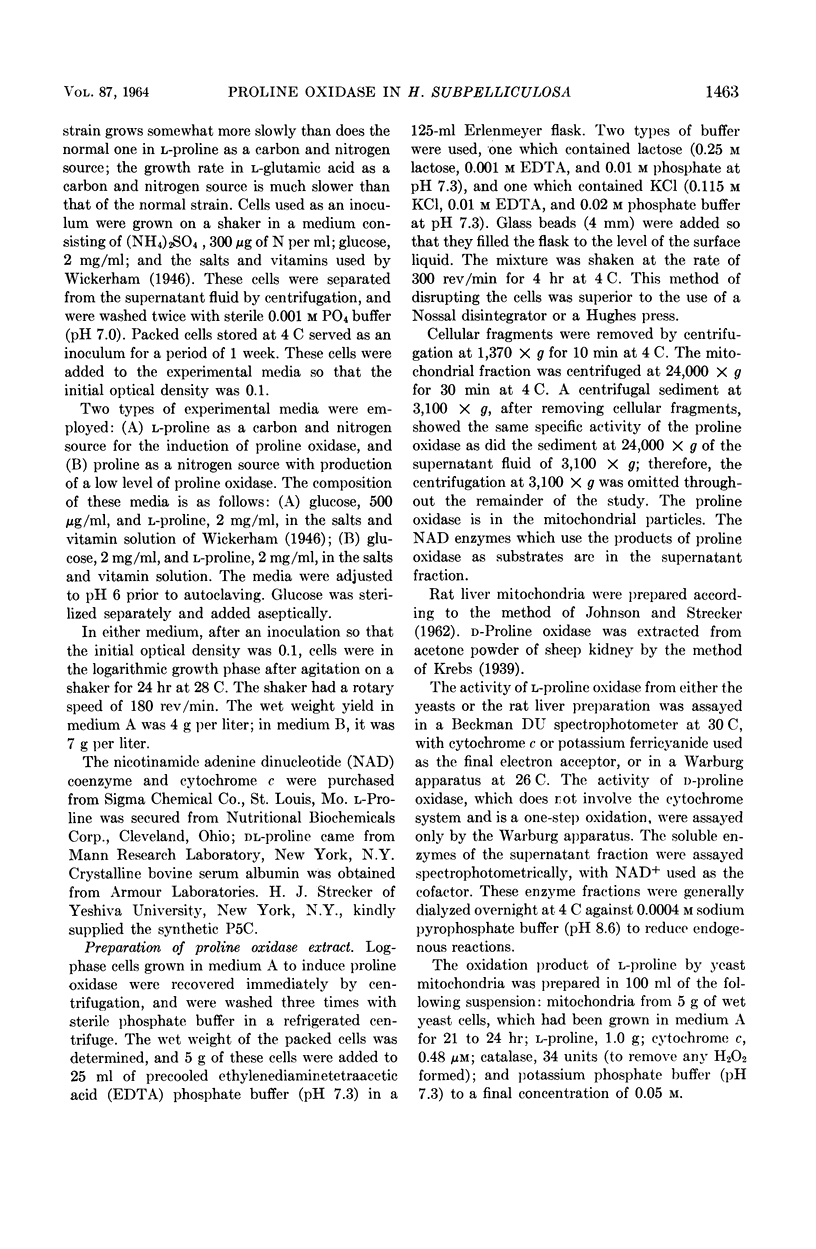
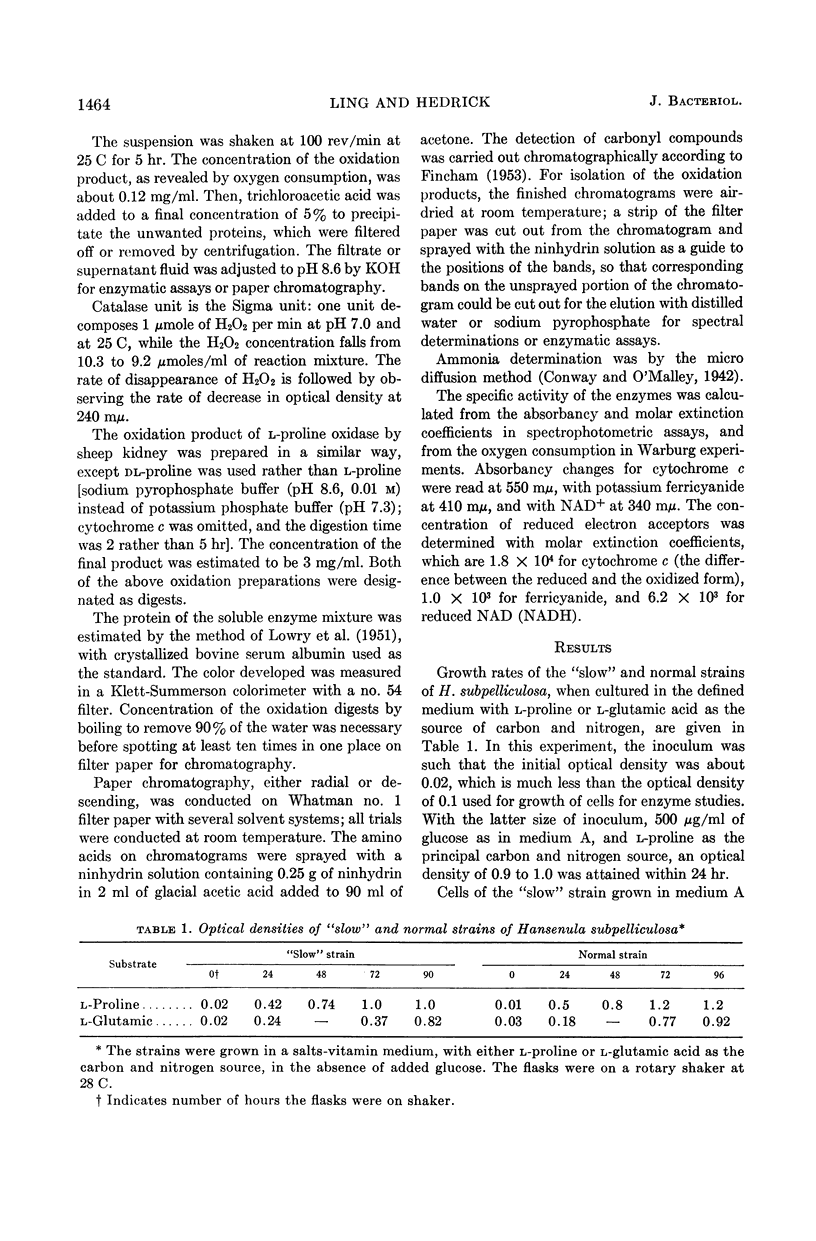
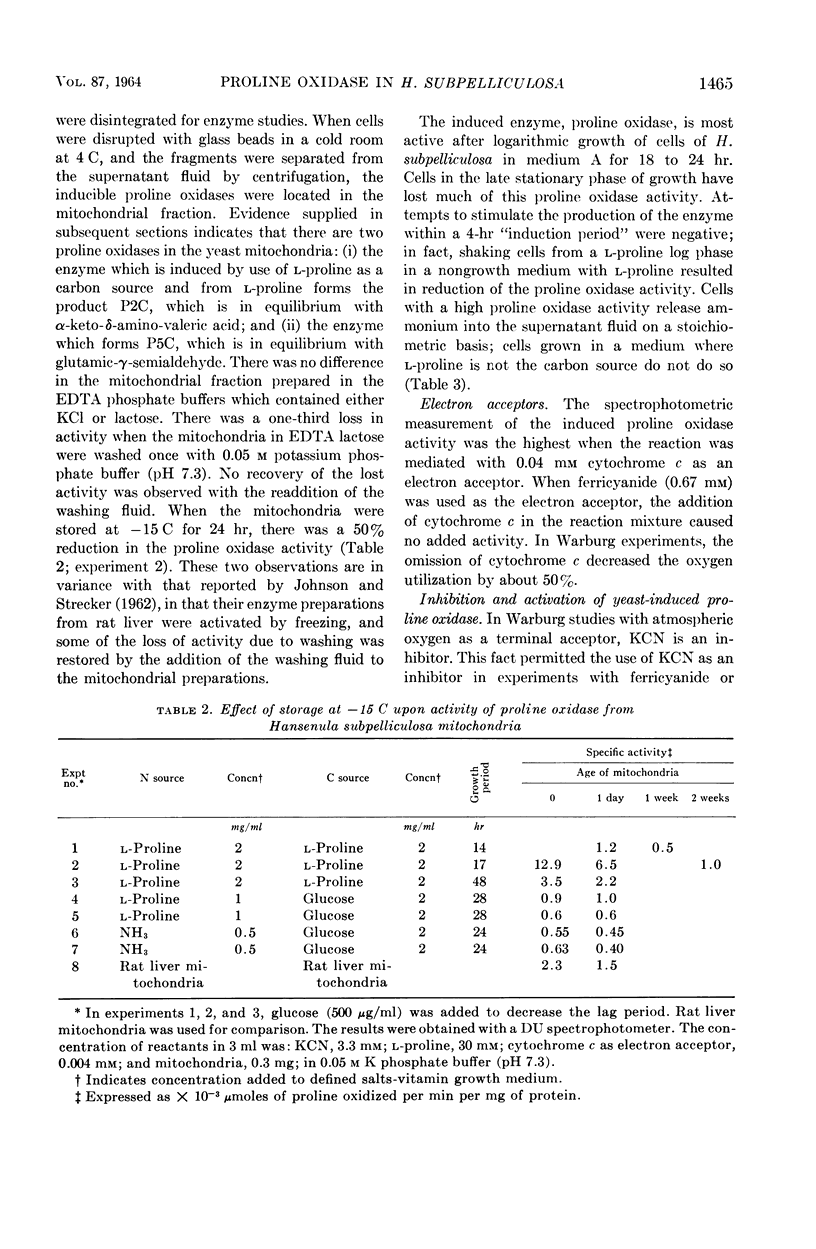
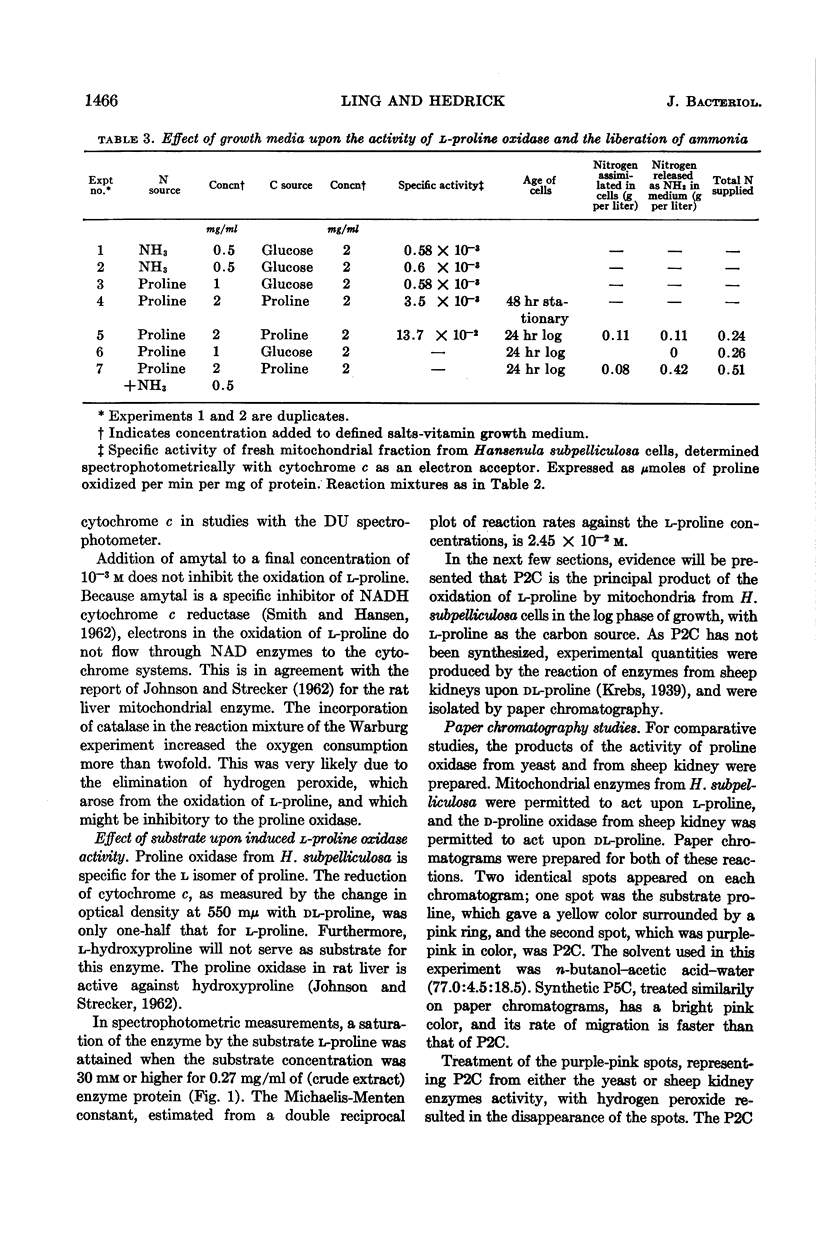
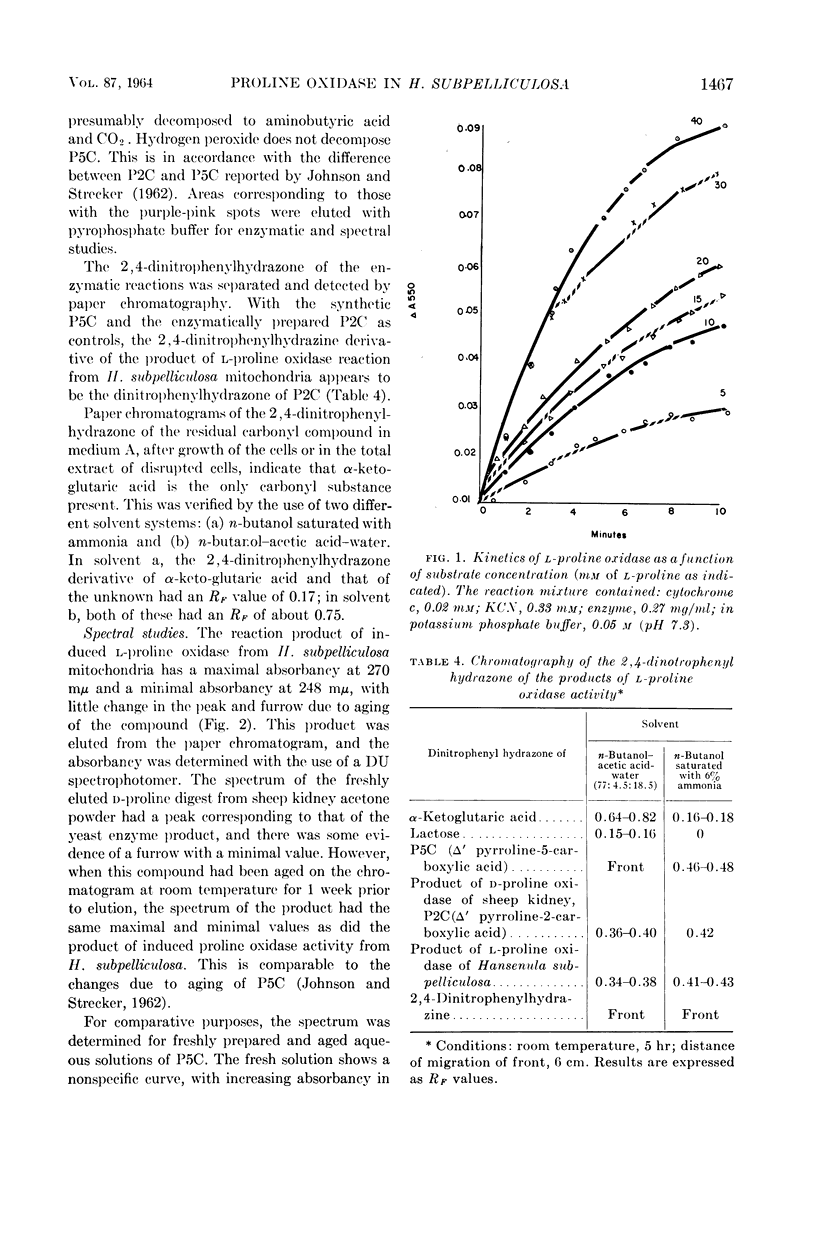
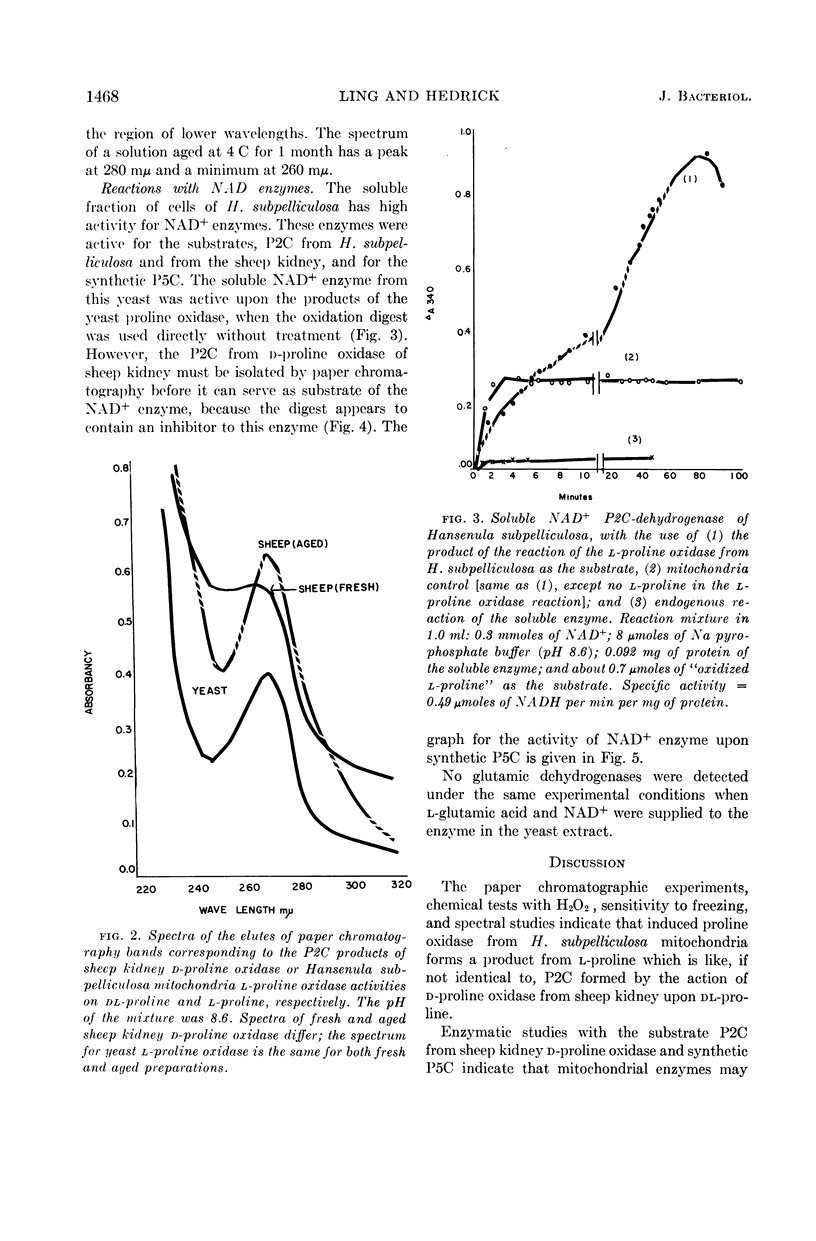
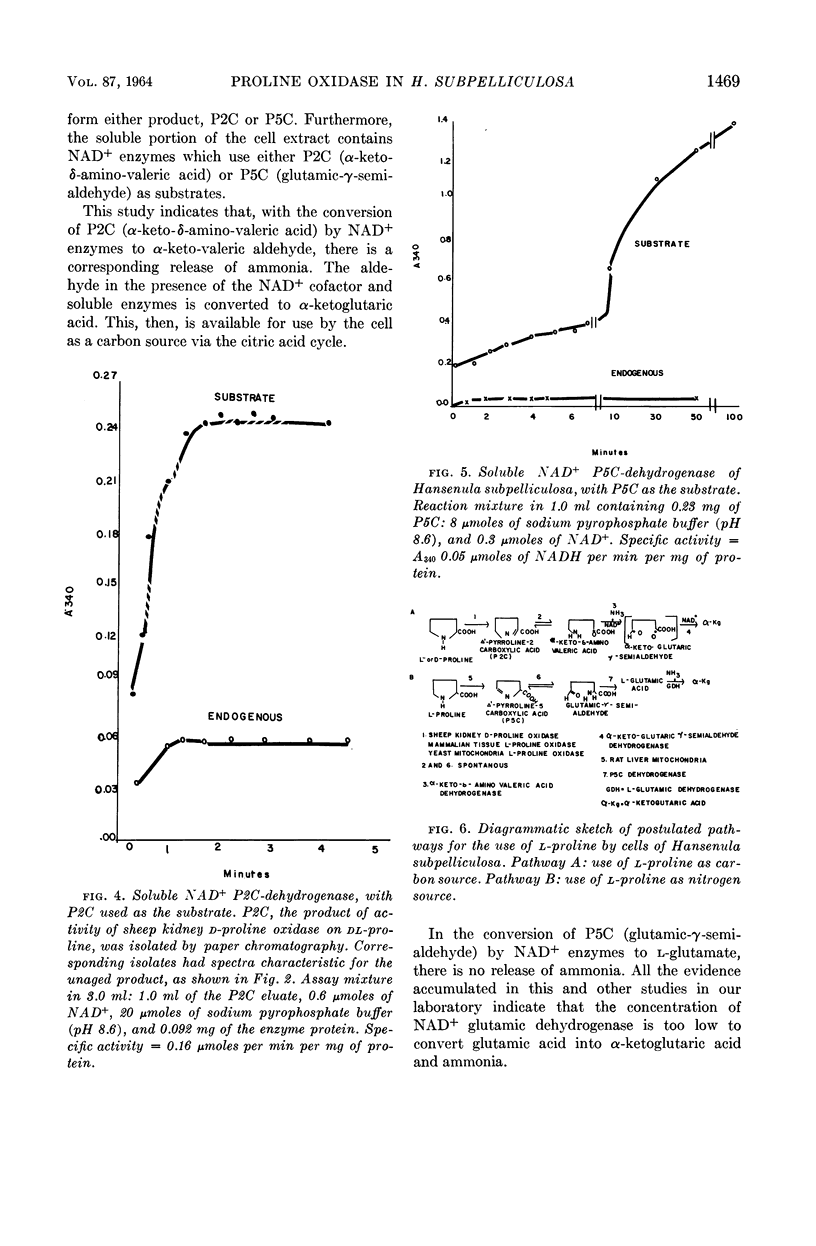
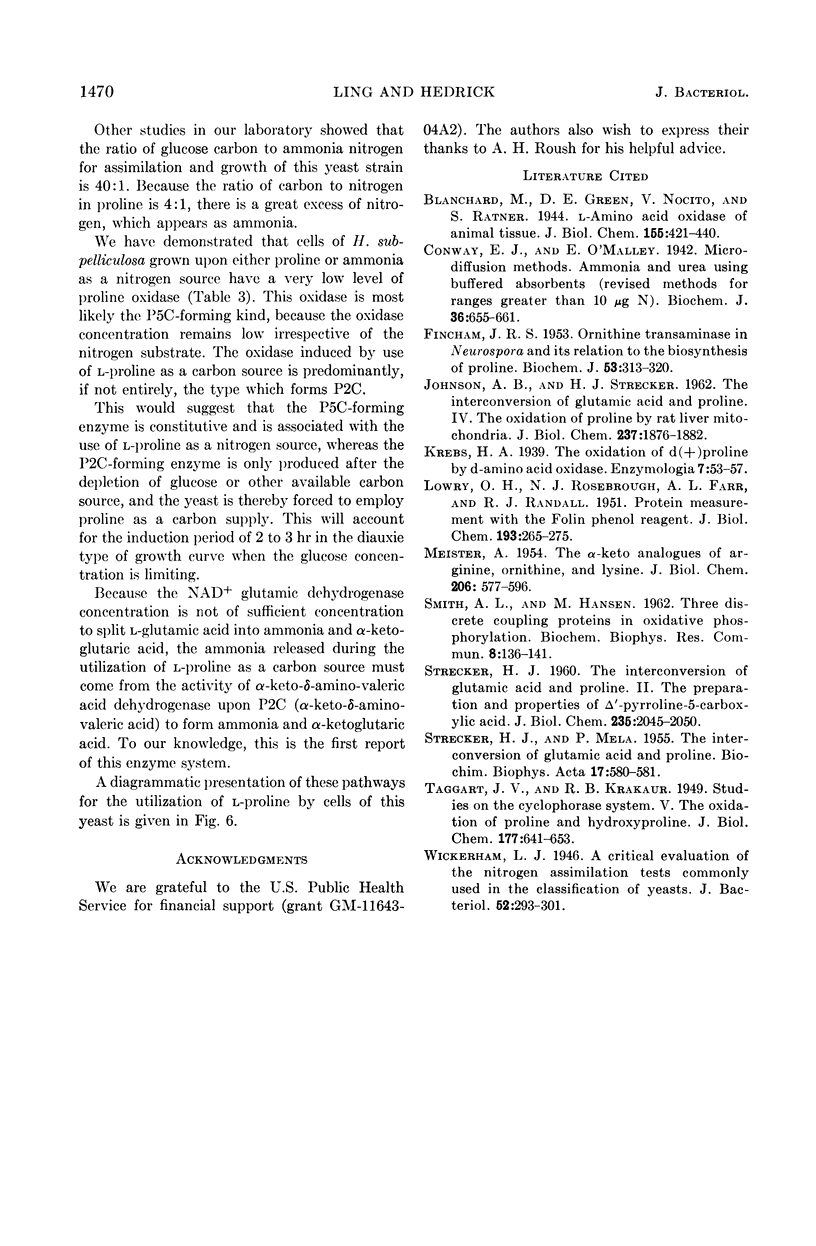
Selected References
These references are in PubMed. This may not be the complete list of references from this article.
- Conway E. J., O'malley E. Microdiffusion methods. Ammonia and urea using buffered absorbents (revised methods for ranges greater than 10mug. N). Biochem J. 1942 Sep;36(7-9):655–661. doi: 10.1042/bj0360655. [DOI] [PMC free article] [PubMed] [Google Scholar]
- FINCHAM J. R. S. Ornithine transaminase in Neurospora and its relation to the biosynthesis of proline. Biochem J. 1953 Jan;53(2):313–320. doi: 10.1042/bj0530313. [DOI] [PMC free article] [PubMed] [Google Scholar]
- JOHNSON A. B., STRECKER H. J. The interconversion of glutamic acid and proline. IV. The oxidation of proline by rat liver mitochondria. J Biol Chem. 1962 Jun;237:1876–1882. [PubMed] [Google Scholar]
- LOWRY O. H., ROSEBROUGH N. J., FARR A. L., RANDALL R. J. Protein measurement with the Folin phenol reagent. J Biol Chem. 1951 Nov;193(1):265–275. [PubMed] [Google Scholar]
- MEISTER A. The alpha-keto analogues of arginine, ornithine, and lysine. J Biol Chem. 1954 Feb;206(2):577–585. [PubMed] [Google Scholar]
- SMITH A. L., HANSEN M. Three discrete coupling proteins in oxidative phosphorylation. Biochem Biophys Res Commun. 1962 Jun 19;8:136–141. doi: 10.1016/0006-291x(62)90251-6. [DOI] [PubMed] [Google Scholar]
- STRECKER H. J., MELA P. The interconversion of glutamic acid and proline. Biochim Biophys Acta. 1955 Aug;17(4):580–581. doi: 10.1016/0006-3002(55)90422-4. [DOI] [PubMed] [Google Scholar]
- STRECKER H. J. The interconversion of glutamic acid and proline. II. The preparation and properties of delta 1-pyrroline-5-carboxylic acid. J Biol Chem. 1960 Jul;235:2045–2050. [PubMed] [Google Scholar]
- Wickerham L. J. A Critical Evaluation of the Nitrogen Assimilation Tests Commonly Used in the Classification of Yeasts. J Bacteriol. 1946 Sep;52(3):293–301. [PMC free article] [PubMed] [Google Scholar]