Abstract
Intracellular Ca2+ release activated by inositol 1,4,5-trisphosphate (InsP3) plays a pivotal role in Ca2+ signaling in cells. A controlling mechanism for InsP3-induced Ca2+ movements is suggested by results showing that the InsP3-releasable Ca2+ pool is directly modified by a specific and sensitive GTP-regulated Ca2+-translocating process. By using saponin-permeabilized N1E-115 neuroblastoma cells or DDT1MF-2 smooth muscle-derived cells, InsP3 releases 30-50% of Ca2+ accumulated through intracellular high-affinity ATP-dependent Ca2+-pumping activity. Oxalate-promoted Ca2+ uptake is reversed by InsP3, indicating oxalate permeability of the InsP3-releasable pool, which is consistent with this compartment being the endoplasmic reticulum. GTP (10 microM) activates release of 50-70% of accumulated Ca2+ from cells. In the presence of 5-10 mM oxalate, GTP induces a biphasic Ca2+ flux response; initially (1-2 min) GTP induces rapid Ca2+ release followed thereafter by a profound increase in Ca2+ uptake. Thus, GTP-activated Ca2+ influx and efflux compete for Ca2+ access to the oxalate-permeable Ca2+ pool. The nonadditive effects of InsP3 and GTP suggest that InsP3 releases Ca2+ from a subcompartment of the GTP-releasable pool. Most significantly, InsP3 is observed to block the GTP-activated uptake phase in the presence of oxalate, indicating that GTP induces Ca2+ entry into the pool from which InsP3 activates release. Hence, the results provide direct evidence that loading of Ca2+ into the InsP3-sensitive Ca2+ pool is controlled by a GTP-regulated Ca2+-translocating mechanism. Such a process could be significant in regulating the extent and duration of the InsP3-induced Ca2+ signal, a crucial step in the inositol phospholipid signaling pathway.
Full text
PDF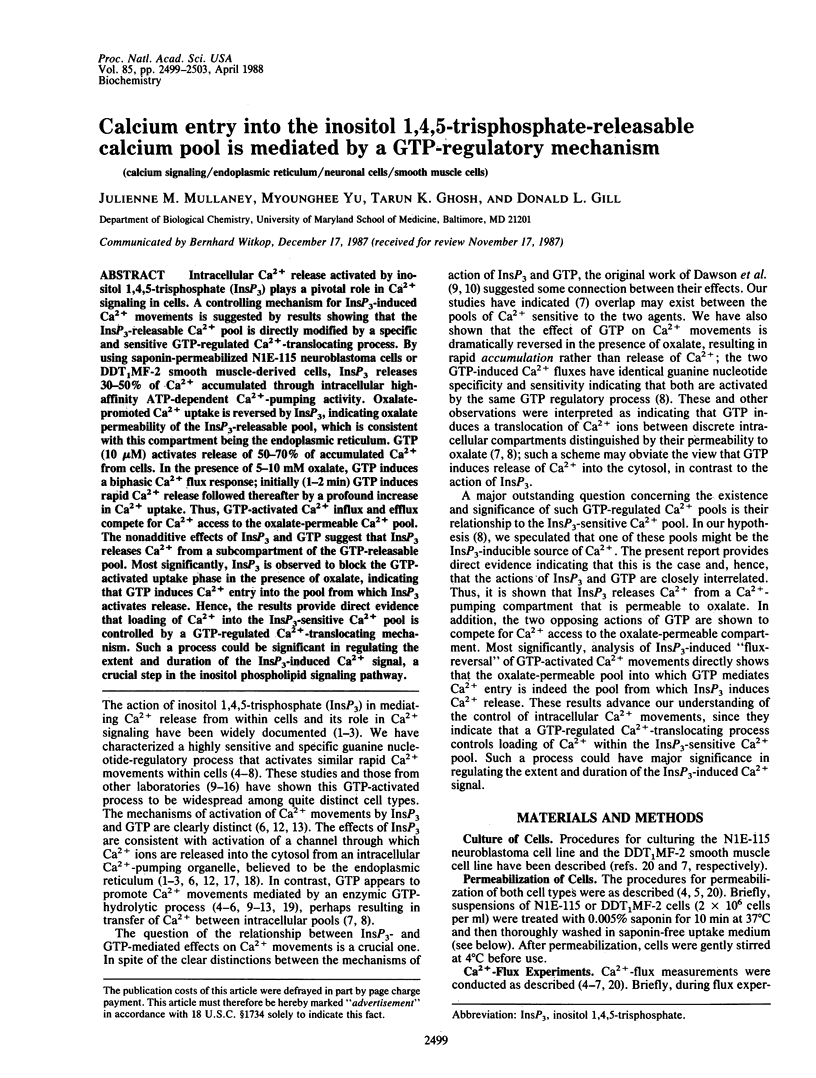
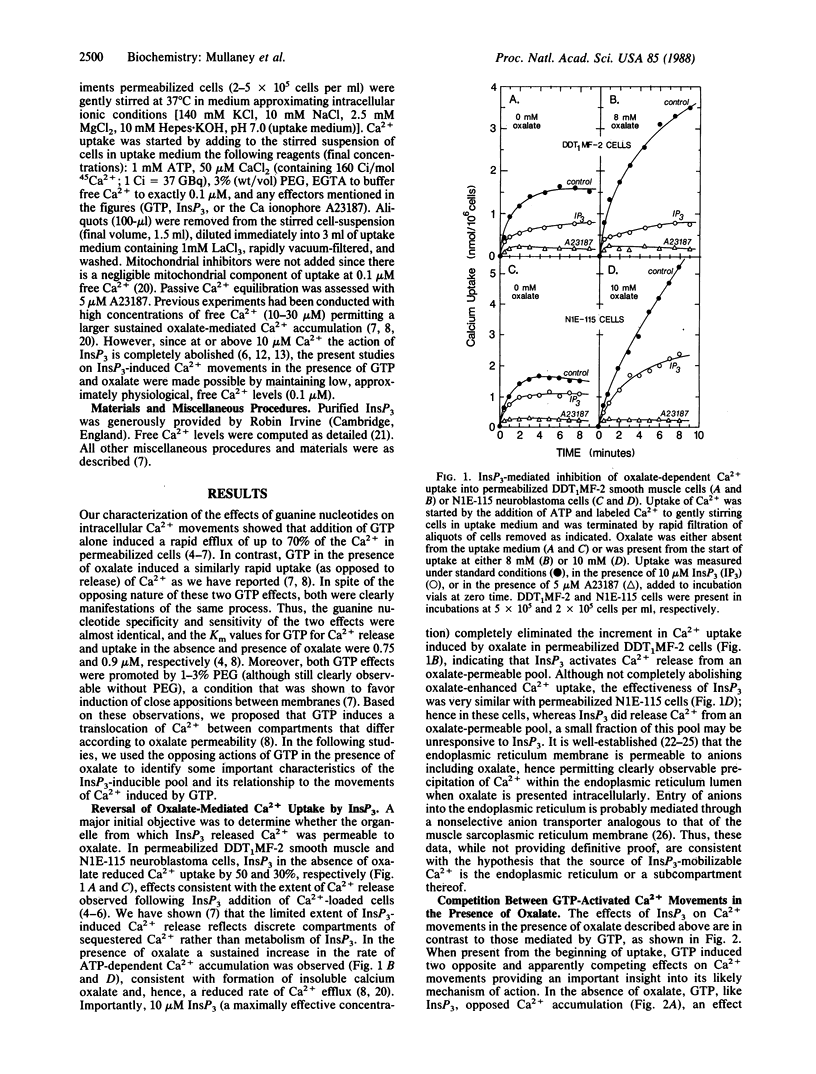
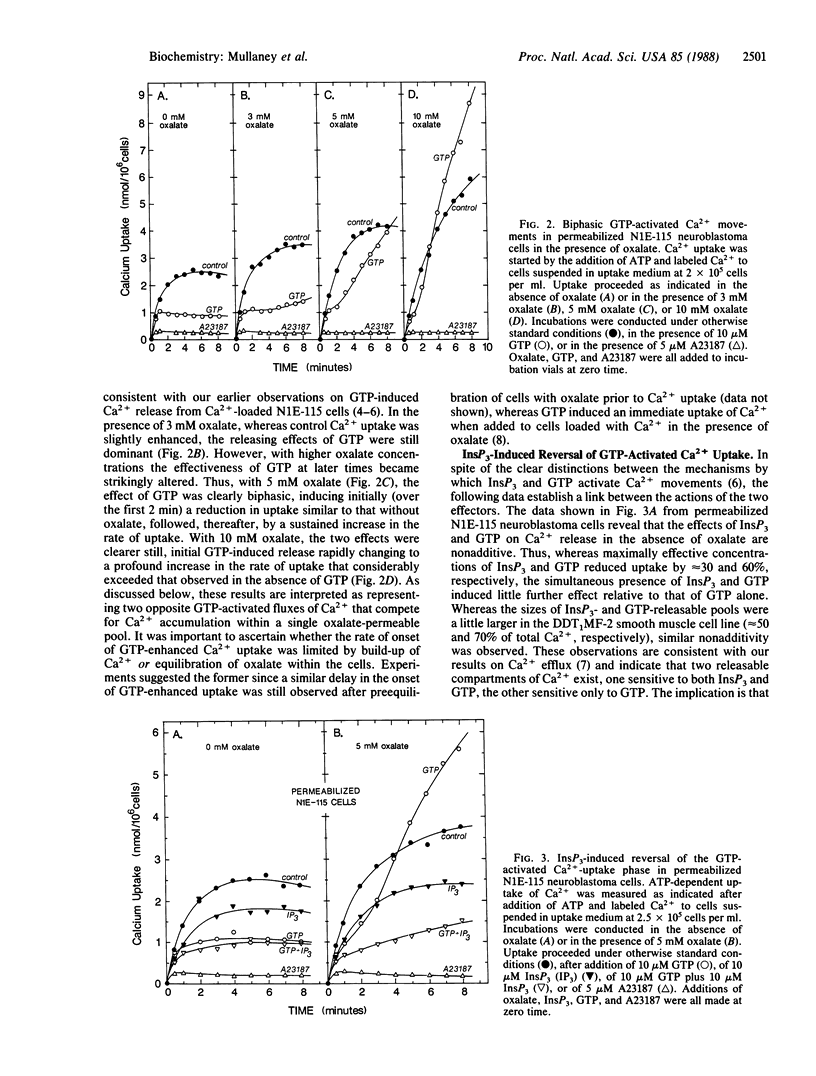
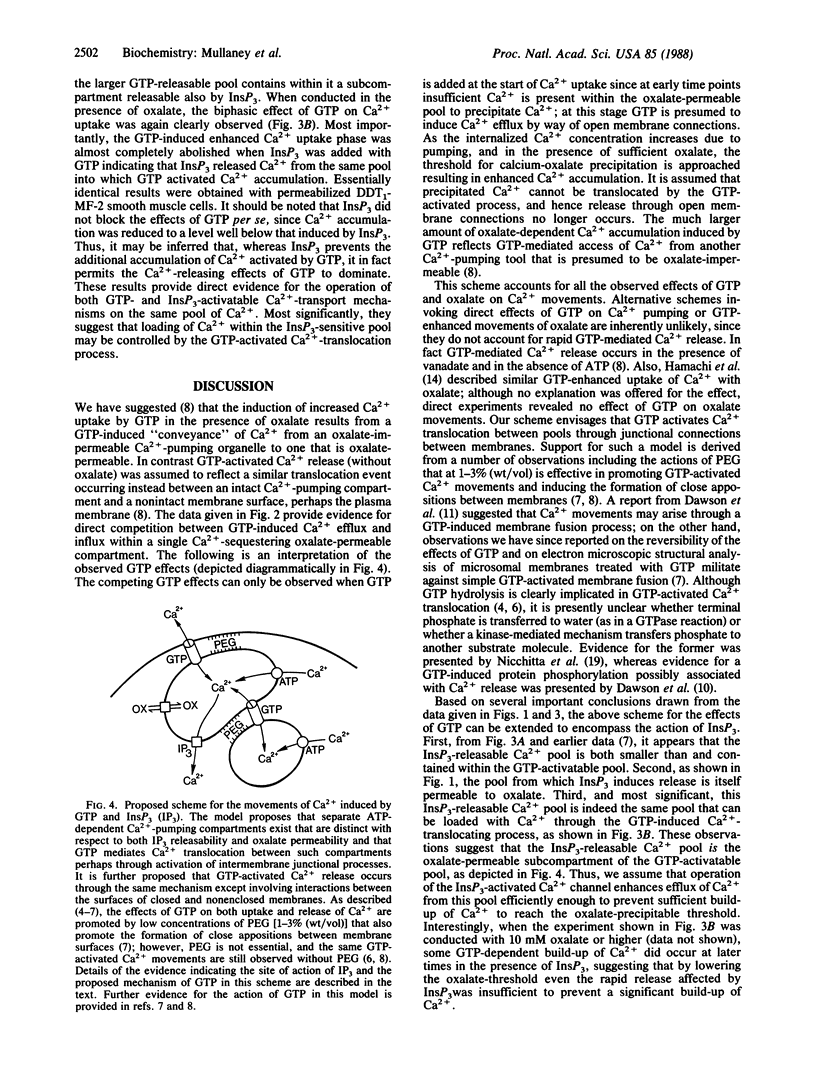
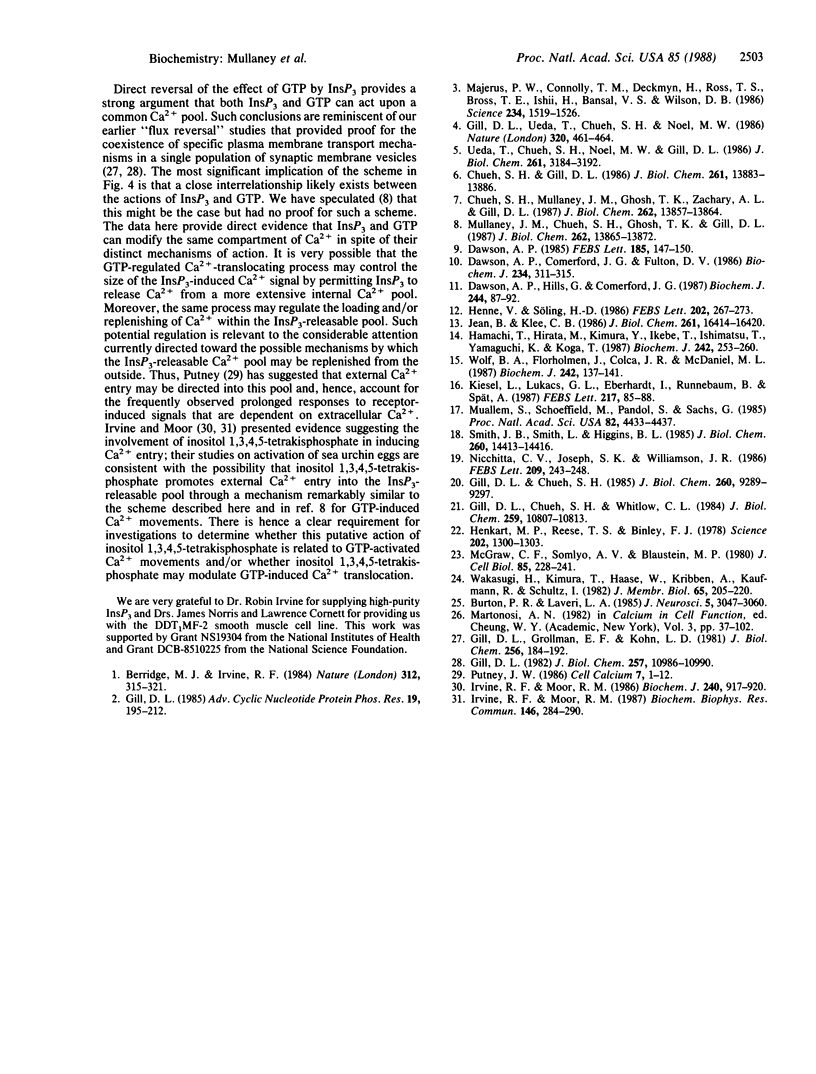
Selected References
These references are in PubMed. This may not be the complete list of references from this article.
- Berridge M. J., Irvine R. F. Inositol trisphosphate, a novel second messenger in cellular signal transduction. Nature. 1984 Nov 22;312(5992):315–321. doi: 10.1038/312315a0. [DOI] [PubMed] [Google Scholar]
- Burton P. R., Laveri L. A. The distribution, relationships to other organelles, and calcium-sequestering ability of smooth endoplasmic reticulum in frog olfactory axons. J Neurosci. 1985 Nov;5(11):3047–3060. doi: 10.1523/JNEUROSCI.05-11-03047.1985. [DOI] [PMC free article] [PubMed] [Google Scholar]
- Chueh S. H., Gill D. L. Inositol 1,4,5-trisphosphate and guanine nucleotides activate calcium release from endoplasmic reticulum via distinct mechanisms. J Biol Chem. 1986 Oct 25;261(30):13883–13886. [PubMed] [Google Scholar]
- Chueh S. H., Mullaney J. M., Ghosh T. K., Zachary A. L., Gill D. L. GTP- and inositol 1,4,5-trisphosphate-activated intracellular calcium movements in neuronal and smooth muscle cell lines. J Biol Chem. 1987 Oct 5;262(28):13857–13864. [PubMed] [Google Scholar]
- Dawson A. P., Comerford J. G., Fulton D. V. The effect of GTP on inositol 1,4,5-trisphosphate-stimulated Ca2+ efflux from a rat liver microsomal fraction. Is a GTP-dependent protein phosphorylation involved? Biochem J. 1986 Mar 1;234(2):311–315. doi: 10.1042/bj2340311. [DOI] [PMC free article] [PubMed] [Google Scholar]
- Dawson A. P. GTP enhances inositol trisphosphate-stimulated Ca2+ release from rat liver microsomes. FEBS Lett. 1985 Jun 3;185(1):147–150. doi: 10.1016/0014-5793(85)80759-6. [DOI] [PubMed] [Google Scholar]
- Dawson A. P., Hills G., Comerford J. G. The mechanism of action of GTP on Ca2+ efflux from rat liver microsomal vesicles. Biochem J. 1987 May 15;244(1):87–92. doi: 10.1042/bj2440087. [DOI] [PMC free article] [PubMed] [Google Scholar]
- Gill D. L., Chueh S. H. An intracellular (ATP + Mg2+)-dependent calcium pump within the N1E-115 neuronal cell line. J Biol Chem. 1985 Aug 5;260(16):9289–9297. [PubMed] [Google Scholar]
- Gill D. L., Chueh S. H., Whitlow C. L. Functional importance of the synaptic plasma membrane calcium pump and sodium-calcium exchanger. J Biol Chem. 1984 Sep 10;259(17):10807–10813. [PubMed] [Google Scholar]
- Gill D. L., Grollman E. F., Kohn L. D. Calcium transport mechanisms in membrane vesicles from guinea pig brain synaptosomes. J Biol Chem. 1981 Jan 10;256(1):184–192. [PubMed] [Google Scholar]
- Gill D. L. Sodium channel, sodium pump, and sodium-calcium exchange activities in synaptosomal plasma membrane vesicles. J Biol Chem. 1982 Sep 25;257(18):10986–10990. [PubMed] [Google Scholar]
- Gill D. L., Ueda T., Chueh S. H., Noel M. W. Ca2+ release from endoplasmic reticulum is mediated by a guanine nucleotide regulatory mechanism. Nature. 1986 Apr 3;320(6061):461–464. doi: 10.1038/320461a0. [DOI] [PubMed] [Google Scholar]
- Hamachi T., Hirata M., Kimura Y., Ikebe T., Ishimatsu T., Yamaguchi K., Koga T. Effect of guanosine triphosphate on the release and uptake of Ca2+ in saponin-permeabilized macrophages and the skeletal-muscle sarcoplasmic reticulum. Biochem J. 1987 Feb 15;242(1):253–260. doi: 10.1042/bj2420253. [DOI] [PMC free article] [PubMed] [Google Scholar]
- Henkart M. P., Reese T. S., Brinley F. J., Jr Endoplasmic reticulum sequesters calcium in the squid giant axon. Science. 1978 Dec 22;202(4374):1300–1303. doi: 10.1126/science.725607. [DOI] [PubMed] [Google Scholar]
- Henne V., Söling H. D. Guanosine 5'-triphosphate releases calcium from rat liver and guinea pig parotid gland endoplasmic reticulum independently of inositol 1,4,5-trisphosphate. FEBS Lett. 1986 Jul 7;202(2):267–273. doi: 10.1016/0014-5793(86)80699-8. [DOI] [PubMed] [Google Scholar]
- Irvine R. F., Moor R. M. Inositol(1,3,4,5)tetrakisphosphate-induced activation of sea urchin eggs requires the presence of inositol trisphosphate. Biochem Biophys Res Commun. 1987 Jul 15;146(1):284–290. doi: 10.1016/0006-291x(87)90723-6. [DOI] [PubMed] [Google Scholar]
- Irvine R. F., Moor R. M. Micro-injection of inositol 1,3,4,5-tetrakisphosphate activates sea urchin eggs by a mechanism dependent on external Ca2+. Biochem J. 1986 Dec 15;240(3):917–920. doi: 10.1042/bj2400917. [DOI] [PMC free article] [PubMed] [Google Scholar]
- Jean T., Klee C. B. Calcium modulation of inositol 1,4,5-trisphosphate-induced calcium release from neuroblastoma x glioma hybrid (NG108-15) microsomes. J Biol Chem. 1986 Dec 15;261(35):16414–16420. [PubMed] [Google Scholar]
- Kiesel L., Lukács G. L., Eberhardt I., Runnebaum B., Spät A. Effect of inositol 1,4,5-trisphosphate and GTP on calcium release from pituitary microsomes. FEBS Lett. 1987 Jun 8;217(1):85–88. doi: 10.1016/0014-5793(87)81248-6. [DOI] [PubMed] [Google Scholar]
- Majerus P. W., Connolly T. M., Deckmyn H., Ross T. S., Bross T. E., Ishii H., Bansal V. S., Wilson D. B. The metabolism of phosphoinositide-derived messenger molecules. Science. 1986 Dec 19;234(4783):1519–1526. doi: 10.1126/science.3024320. [DOI] [PubMed] [Google Scholar]
- McGraw C. F., Somlyo A. V., Blaustein M. P. Localization of calcium in presynaptic nerve terminals. An ultrastructural and electron microprobe analysis. J Cell Biol. 1980 May;85(2):228–241. doi: 10.1083/jcb.85.2.228. [DOI] [PMC free article] [PubMed] [Google Scholar]
- Muallem S., Schoeffield M., Pandol S., Sachs G. Inositol trisphosphate modification of ion transport in rough endoplasmic reticulum. Proc Natl Acad Sci U S A. 1985 Jul;82(13):4433–4437. doi: 10.1073/pnas.82.13.4433. [DOI] [PMC free article] [PubMed] [Google Scholar]
- Mullaney J. M., Chueh S. H., Ghosh T. K., Gill D. L. Intracellular calcium uptake activated by GTP. Evidence for a possible guanine nucleotide-induced transmembrane conveyance of intracellular calcium. J Biol Chem. 1987 Oct 5;262(28):13865–13872. [PubMed] [Google Scholar]
- Nicchitta C. V., Joseph S. K., Williamson J. R. Polyethylene glycol-stimulated microsomal GTP hydrolysis. Relationship to GTP-mediated Ca2+ release. FEBS Lett. 1986 Dec 15;209(2):243–248. doi: 10.1016/0014-5793(86)81120-6. [DOI] [PubMed] [Google Scholar]
- Putney J. W., Jr A model for receptor-regulated calcium entry. Cell Calcium. 1986 Feb;7(1):1–12. doi: 10.1016/0143-4160(86)90026-6. [DOI] [PubMed] [Google Scholar]
- Smith J. B., Smith L., Higgins B. L. Temperature and nucleotide dependence of calcium release by myo-inositol 1,4,5-trisphosphate in cultured vascular smooth muscle cells. J Biol Chem. 1985 Nov 25;260(27):14413–14416. [PubMed] [Google Scholar]
- Ueda T., Chueh S. H., Noel M. W., Gill D. L. Influence of inositol 1,4,5-trisphosphate and guanine nucleotides on intracellular calcium release within the N1E-115 neuronal cell line. J Biol Chem. 1986 Mar 5;261(7):3184–3192. [PubMed] [Google Scholar]
- Wakasugi H., Kimura T., Haase W., Kribben A., Kaufmann R., Schulz I. Calcium uptake into acini from rat pancreas: evidence for intracellular ATP-dependent calcium sequestration. J Membr Biol. 1982;65(3):205–220. doi: 10.1007/BF01869964. [DOI] [PubMed] [Google Scholar]
- Wolf B. A., Florholmen J., Colca J. R., McDaniel M. L. GTP mobilization of Ca2+ from the endoplasmic reticulum of islets. Comparison with myo-inositol 1,4,5-trisphosphate. Biochem J. 1987 Feb 15;242(1):137–141. doi: 10.1042/bj2420137. [DOI] [PMC free article] [PubMed] [Google Scholar]