Abstract
Recent studies have suggested that the canalicular bile salt transport system of rat liver corresponds to a 100-kDa membrane glycoprotein. In the present study we attempted to functionally reconstitute the 100-kDa protein into artificial proteoliposomes. Canalicular membrane proteins were solubilized with octyl glucoside in the presence of asolectin phospholipids. The extracts were treated with preimmune serum or the 100-kDa protein selectively immunoprecipitated with a polyclonal antiserum. Proteins remaining in the supernatant were then incorporated into proteoliposomes by gel-filtration chromatography. Canalicular proteoliposomes containing the 100-kDa protein exhibited transstimulatable taurocholate uptake that could be inhibited by 4,4'-diisothiocyanato-2,2'-stilbenedisulfonic acid (DIDS). In contrast, no DIDS-sensitive transstimulatable taurocholate uptake was found in 100-kDa protein-free canalicular proteoliposomes. However, when the immunoprecipitated 100-kDa protein was dissociated from the antibodies and exclusively incorporated into liposomes, reconstitution of DIDS-sensitive transstimulatable and electrogenic taurocholate anion transport was again positive. Although incorporation of solubilized basolateral membrane proteins into liposomes also resulted in a prompt reconstitution of Na+ gradient-driven taurocholate uptake, the anti-100-kDa antibodies had no effects on the reconstituted transport activity of basolateral proteins. Thus, the findings establish that the previously characterized canalicular-specific 100-kDa protein is directly involved in the transcanalicular secretion of bile salts.
Full text
PDF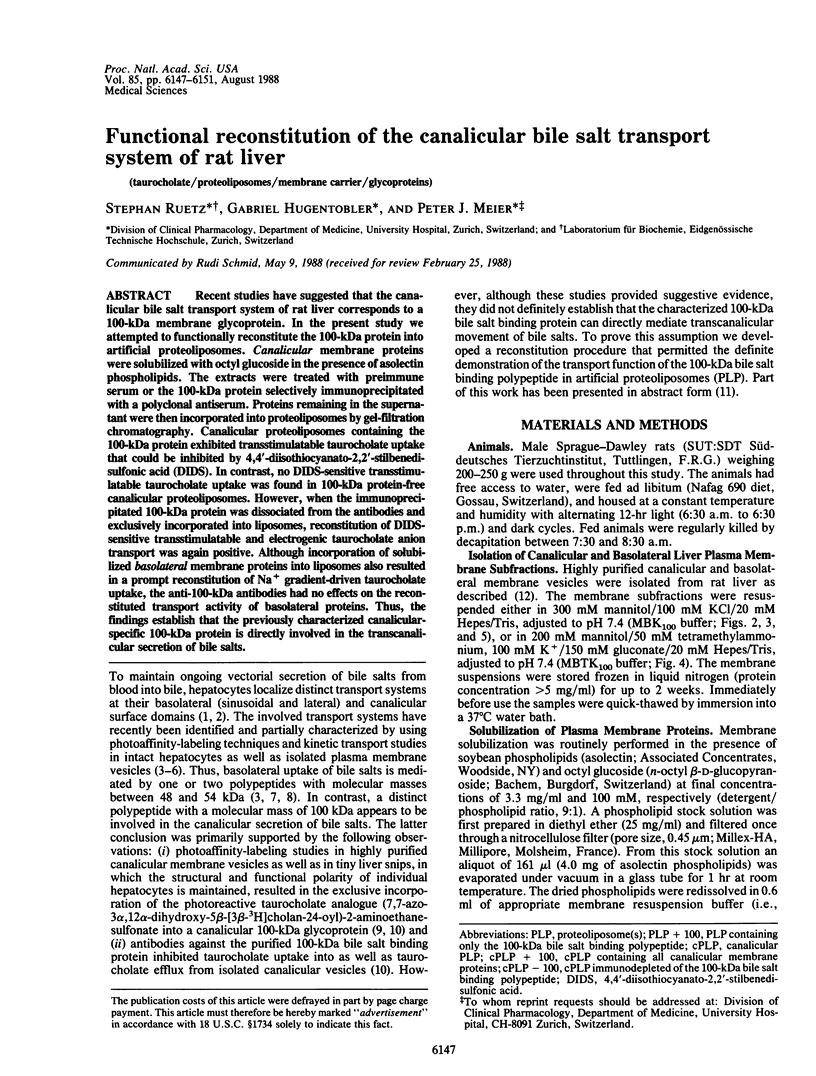
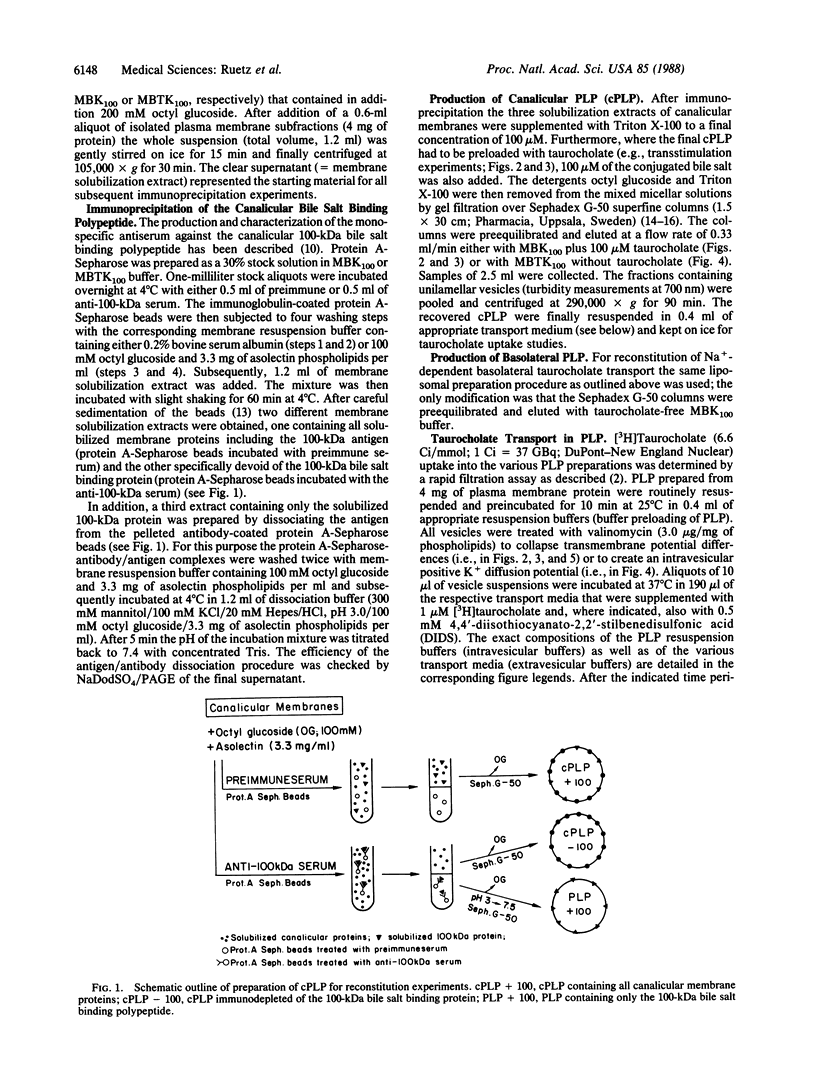
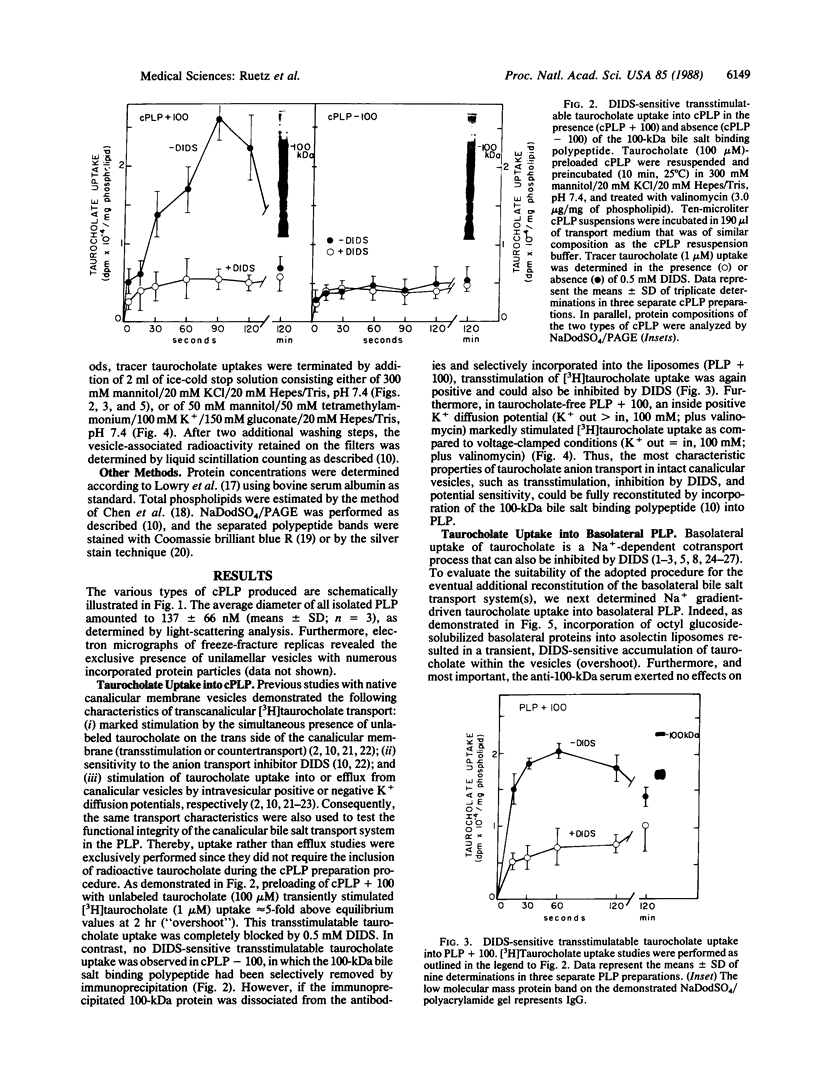
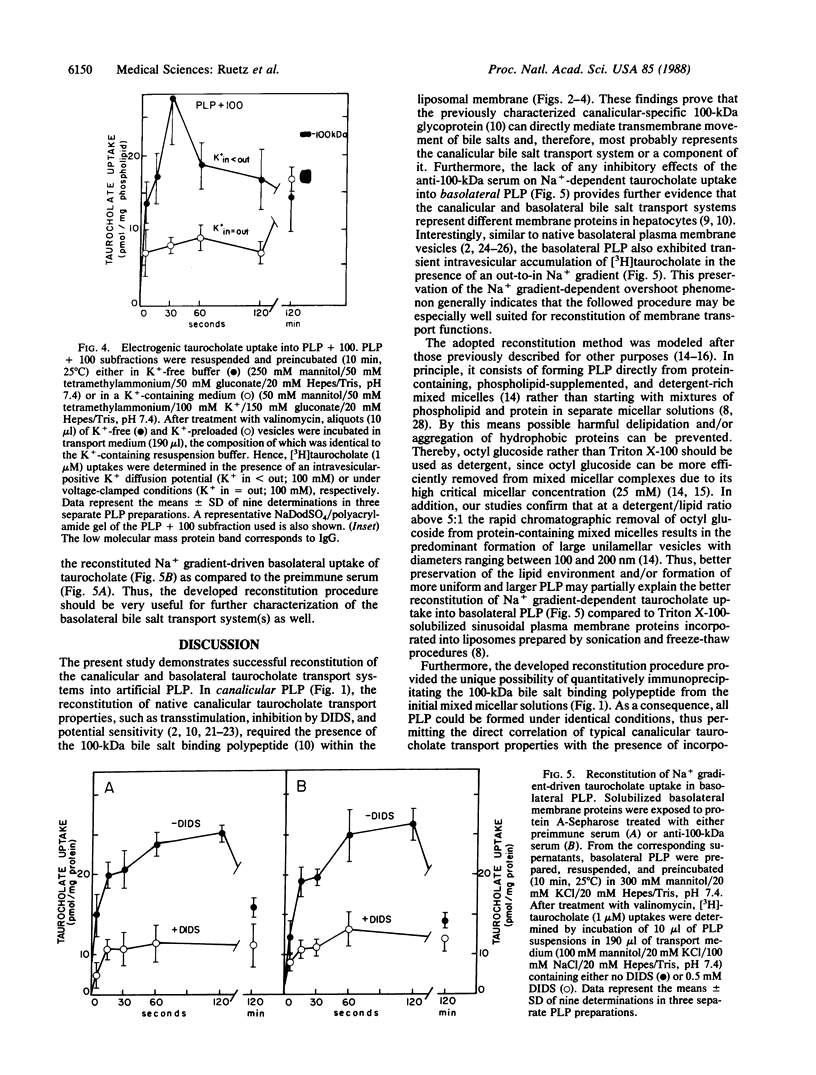
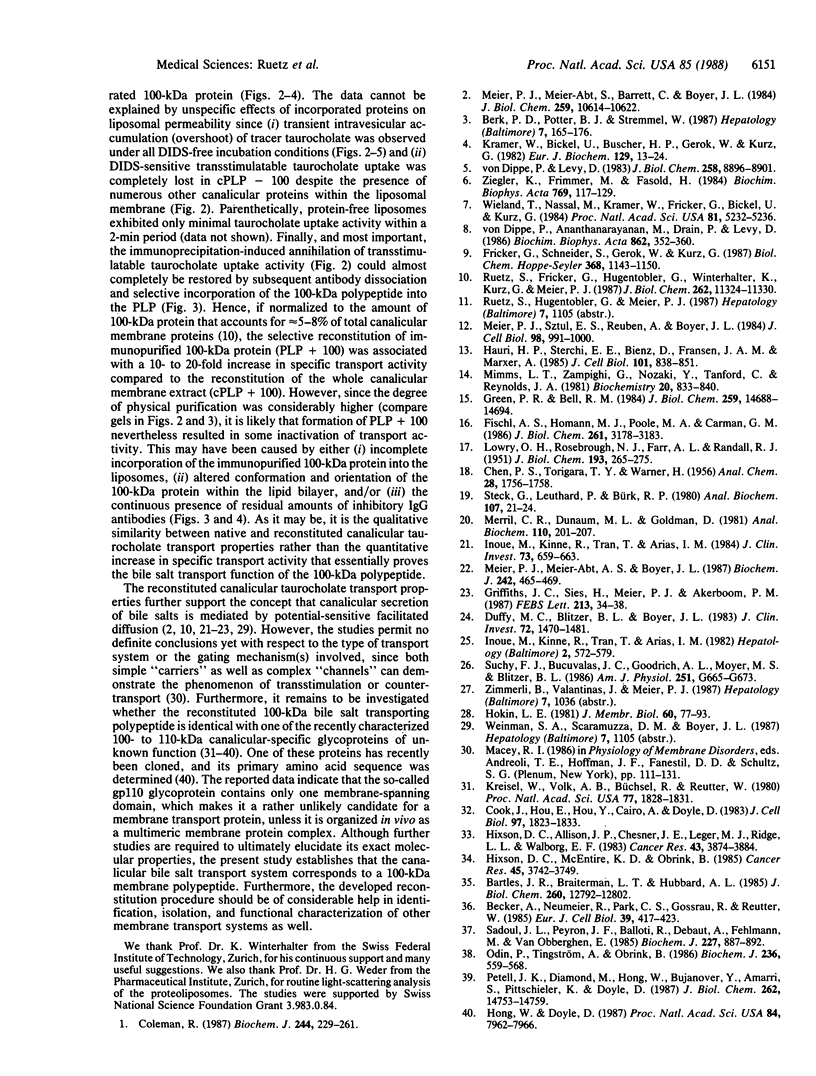
Images in this article
Selected References
These references are in PubMed. This may not be the complete list of references from this article.
- Bartles J. R., Braiterman L. T., Hubbard A. L. Biochemical characterization of domain-specific glycoproteins of the rat hepatocyte plasma membrane. J Biol Chem. 1985 Oct 15;260(23):12792–12802. [PubMed] [Google Scholar]
- Becker A., Neumeier R., Park C. S., Gossrau R., Reutter W. Identification of a transformation-sensitive 110-kDa plasma membrane glycoprotein of rat hepatocytes. Eur J Cell Biol. 1986 Jan;39(2):417–423. [PubMed] [Google Scholar]
- Berk P. D., Potter B. J., Stremmel W. Role of plasma membrane ligand-binding proteins in the hepatocellular uptake of albumin-bound organic anions. Hepatology. 1987 Jan-Feb;7(1):165–176. doi: 10.1002/hep.1840070131. [DOI] [PubMed] [Google Scholar]
- Coleman R. Biochemistry of bile secretion. Biochem J. 1987 Jun 1;244(2):249–261. doi: 10.1042/bj2440249. [DOI] [PMC free article] [PubMed] [Google Scholar]
- Cook J., Hou E., Hou Y., Cairo A., Doyle D. Establishment of plasma membrane domains in hepatocytes. I. Characterization and localization to the bile canaliculus of three antigens externally oriented in the plasma membrane. J Cell Biol. 1983 Dec;97(6):1823–1833. doi: 10.1083/jcb.97.6.1823. [DOI] [PMC free article] [PubMed] [Google Scholar]
- Duffy M. C., Blitzer B. L., Boyer J. L. Direct determination of the driving forces for taurocholate uptake into rat liver plasma membrane vesicles. J Clin Invest. 1983 Oct;72(4):1470–1481. doi: 10.1172/JCI111103. [DOI] [PMC free article] [PubMed] [Google Scholar]
- Fischl A. S., Homann M. J., Poole M. A., Carman G. M. Phosphatidylinositol synthase from Saccharomyces cerevisiae. Reconstitution, characterization, and regulation of activity. J Biol Chem. 1986 Mar 5;261(7):3178–3183. [PubMed] [Google Scholar]
- Fricker G., Schneider S., Gerok W., Kurz G. Identification of different transport systems for bile salts in sinusoidal and canalicular membranes of hepatocytes. Biol Chem Hoppe Seyler. 1987 Sep;368(9):1143–1150. doi: 10.1515/bchm3.1987.368.2.1143. [DOI] [PubMed] [Google Scholar]
- Green P. R., Bell R. M. Asymmetric reconstitution of homogeneous Escherichia coli sn-glycerol-3-phosphate acyltransferase into phospholipid vesicles. J Biol Chem. 1984 Dec 10;259(23):14688–14694. [PubMed] [Google Scholar]
- Griffiths J. C., Sies H., Meier P. J., Akerboom T. P. Inhibition of taurocholate efflux from rat hepatic canalicular membrane vesicles by glutathione disulfide. FEBS Lett. 1987 Mar 9;213(1):34–38. doi: 10.1016/0014-5793(87)81460-6. [DOI] [PubMed] [Google Scholar]
- Hauri H. P., Sterchi E. E., Bienz D., Fransen J. A., Marxer A. Expression and intracellular transport of microvillus membrane hydrolases in human intestinal epithelial cells. J Cell Biol. 1985 Sep;101(3):838–851. doi: 10.1083/jcb.101.3.838. [DOI] [PMC free article] [PubMed] [Google Scholar]
- Hixson D. C., Allison J. P., Chesner J. E., Leger M. J., Ridge L. L., Walborg E. F., Jr Characterization of a family of glycoproteins associated with the bile canalicular membrane of normal hepatocytes but not expressed by two transplantable rat hepatocellular carcinomas. Cancer Res. 1983 Aug;43(8):3874–3884. [PubMed] [Google Scholar]
- Hixson D. C., McEntire K. D., Obrink B. Alterations in the expression of a hepatocyte cell adhesion molecule by transplantable rat hepatocellular carcinomas. Cancer Res. 1985 Aug;45(8):3742–3749. [PubMed] [Google Scholar]
- Hokin L. E. Reconstitution of "carriers" in artificial membranes. J Membr Biol. 1981;60(2):77–93. doi: 10.1007/BF01870412. [DOI] [PubMed] [Google Scholar]
- Hong W., Doyle D. cDNA cloning for a bile canaliculus domain-specific membrane glycoprotein of rat hepatocytes. Proc Natl Acad Sci U S A. 1987 Nov;84(22):7962–7966. doi: 10.1073/pnas.84.22.7962. [DOI] [PMC free article] [PubMed] [Google Scholar]
- Inoue M., Kinne R., Tran T., Arias I. M. Taurocholate transport by rat liver canalicular membrane vesicles. Evidence for the presence of an Na+-independent transport system. J Clin Invest. 1984 Mar;73(3):659–663. doi: 10.1172/JCI111257. [DOI] [PMC free article] [PubMed] [Google Scholar]
- Inoue M., Kinne R., Tran T., Arias I. M. Taurocholate transport by rat liver sinusoidal membrane vesicles: evidence of sodium cotransport. Hepatology. 1982 Sep-Oct;2(5):572–579. doi: 10.1002/hep.1840020510. [DOI] [PubMed] [Google Scholar]
- Kramer W., Bickel U., Buscher H. P., Gerok W., Kurz G. Bile-salt-binding polypeptides in plasma membranes of hepatocytes revealed by photoaffinity labelling. Eur J Biochem. 1982 Dec;129(1):13–24. doi: 10.1111/j.1432-1033.1982.tb07015.x. [DOI] [PubMed] [Google Scholar]
- Kreisel W., Volk B. A., Büchsel R., Reutter W. Different half-lives of the carbohydrate and protein moieties of a 110,000-dalton glycoprotein isolated from plasma membranes of rat liver. Proc Natl Acad Sci U S A. 1980 Apr;77(4):1828–1831. doi: 10.1073/pnas.77.4.1828. [DOI] [PMC free article] [PubMed] [Google Scholar]
- LOWRY O. H., ROSEBROUGH N. J., FARR A. L., RANDALL R. J. Protein measurement with the Folin phenol reagent. J Biol Chem. 1951 Nov;193(1):265–275. [PubMed] [Google Scholar]
- Meier P. J., Meier-Abt A. S., Boyer J. L. Properties of the canalicular bile acid transport system in rat liver. Biochem J. 1987 Mar 1;242(2):465–469. doi: 10.1042/bj2420465. [DOI] [PMC free article] [PubMed] [Google Scholar]
- Meier P. J., St Meier-Abt A., Barrett C., Boyer J. L. Mechanisms of taurocholate transport in canalicular and basolateral rat liver plasma membrane vesicles. Evidence for an electrogenic canalicular organic anion carrier. J Biol Chem. 1984 Aug 25;259(16):10614–10622. [PubMed] [Google Scholar]
- Meier P. J., Sztul E. S., Reuben A., Boyer J. L. Structural and functional polarity of canalicular and basolateral plasma membrane vesicles isolated in high yield from rat liver. J Cell Biol. 1984 Mar;98(3):991–1000. doi: 10.1083/jcb.98.3.991. [DOI] [PMC free article] [PubMed] [Google Scholar]
- Merril C. R., Dunau M. L., Goldman D. A rapid sensitive silver stain for polypeptides in polyacrylamide gels. Anal Biochem. 1981 Jan 1;110(1):201–207. doi: 10.1016/0003-2697(81)90136-6. [DOI] [PubMed] [Google Scholar]
- Mimms L. T., Zampighi G., Nozaki Y., Tanford C., Reynolds J. A. Phospholipid vesicle formation and transmembrane protein incorporation using octyl glucoside. Biochemistry. 1981 Feb 17;20(4):833–840. doi: 10.1021/bi00507a028. [DOI] [PubMed] [Google Scholar]
- Odin P., Tingström A., Obrink B. Chemical characterization of cell-CAM 105, a cell-adhesion molecule isolated from rat liver membranes. Biochem J. 1986 Jun 1;236(2):559–568. doi: 10.1042/bj2360559. [DOI] [PMC free article] [PubMed] [Google Scholar]
- Petell J. K., Diamond M., Hong W. J., Bujanover Y., Amarri S., Pittschieler K., Doyle D. Isolation and characterization of a Mr = 110,000 glycoprotein localized to the hepatocyte bile canaliculus. J Biol Chem. 1987 Oct 25;262(30):14753–14759. [PubMed] [Google Scholar]
- Ruetz S., Fricker G., Hugentobler G., Winterhalter K., Kurz G., Meier P. J. Isolation and characterization of the putative canalicular bile salt transport system of rat liver. J Biol Chem. 1987 Aug 15;262(23):11324–11330. [PubMed] [Google Scholar]
- Sadoul J. L., Peyron J. F., Ballotti R., Debant A., Fehlmann M., Van Obberghen E. Identification of a cellular 110 000-Da protein substrate for the insulin-receptor kinase. Biochem J. 1985 May 1;227(3):887–892. doi: 10.1042/bj2270887. [DOI] [PMC free article] [PubMed] [Google Scholar]
- Steck G., Leuthard P., Bürk R. R. Detection of basic proteins and low molecular weight peptides in polyacrylamide gels by formaldehyde fixation. Anal Biochem. 1980 Sep 1;107(1):21–24. doi: 10.1016/0003-2697(80)90486-8. [DOI] [PubMed] [Google Scholar]
- Suchy F. J., Bucuvalas J. C., Goodrich A. L., Moyer M. S., Blitzer B. L. Taurocholate transport and Na+-K+-ATPase activity in fetal and neonatal rat liver plasma membrane vesicles. Am J Physiol. 1986 Nov;251(5 Pt 1):G665–G673. doi: 10.1152/ajpgi.1986.251.5.G665. [DOI] [PubMed] [Google Scholar]
- Wieland T., Nassal M., Kramer W., Fricker G., Bickel U., Kurz G. Identity of hepatic membrane transport systems for bile salts, phalloidin, and antamanide by photoaffinity labeling. Proc Natl Acad Sci U S A. 1984 Aug;81(16):5232–5236. doi: 10.1073/pnas.81.16.5232. [DOI] [PMC free article] [PubMed] [Google Scholar]
- Ziegler K., Frimmer M., Fasold H. Further characterization of membrane proteins involved in the transport of organic anions in hepatocytes. Comparison of two different affinity labels: 4,4'-diisothiocyano-1,2-diphenylethane-2,2'-disulfonic acid and brominated taurodehydrocholic acid. Biochim Biophys Acta. 1984 Jan 11;769(1):117–129. doi: 10.1016/0005-2736(84)90015-4. [DOI] [PubMed] [Google Scholar]
- von Dippe P., Ananthanarayanan M., Drain P., Levy D. Purification and reconstitution of the bile acid transport system from hepatocyte sinusoidal plasma membranes. Biochim Biophys Acta. 1986 Nov 17;862(2):352–360. doi: 10.1016/0005-2736(86)90238-5. [DOI] [PubMed] [Google Scholar]
- von Dippe P., Levy D. Characterization of the bile acid transport system in normal and transformed hepatocytes. Photoaffinity labeling of the taurocholate carrier protein. J Biol Chem. 1983 Jul 25;258(14):8896–8901. [PubMed] [Google Scholar]