Abstract
Anoxia of the heart causes failure of contraction before any irreversible injury occurs; the mechanism by which anoxia blocks cardiac excitation-contraction coupling is unknown. Studies in whole muscle are confounded by heterogeneity; however, achieving the low oxygen tensions required to study anoxia in a single myocyte during electrophysiological recording has been a barrier in experimental design. Guided by calculations of oxygen transport, we developed a system to insulate myocytes in an open dish from oxygen by a laminar counterflowing argon column, permitting free access to the cell by microelectrodes while maintaining a PO2 less than 0.02 torr (1 torr = 133 Pa). In the absence of glucose, the amplitude of stimulated contraction of anoxic ventricular myocytes fell to zero over 2 min after a lag period attributable to the consumption of endogenous glycogen. The cytosolic calcium concentration transient, measured by indo-1 fluorescence, fell to zero simultaneously with contraction. After the twitch had failed, microinjection of caffeine around the cell still caused a large calcium release and contraction, indicating that sarcoplasmic reticular calcium stores were not depleted. Twitch failure was accompanied by shortening and then failure of the action potential; under voltage clamp, large outward currents, reversing at the resting potential, developed during contractile failure. After failure of action potential-mediated contraction, voltage-clamp depolarization, with a large command voltage to compensate for the series-resistance error due to outward currents, restored normal twitch contraction. We conclude that anoxic contractile failure in the rat myocyte is due to alteration of the action potential and the distal pathways of excitation-contraction coupling remain essentially intact.
Full text
PDF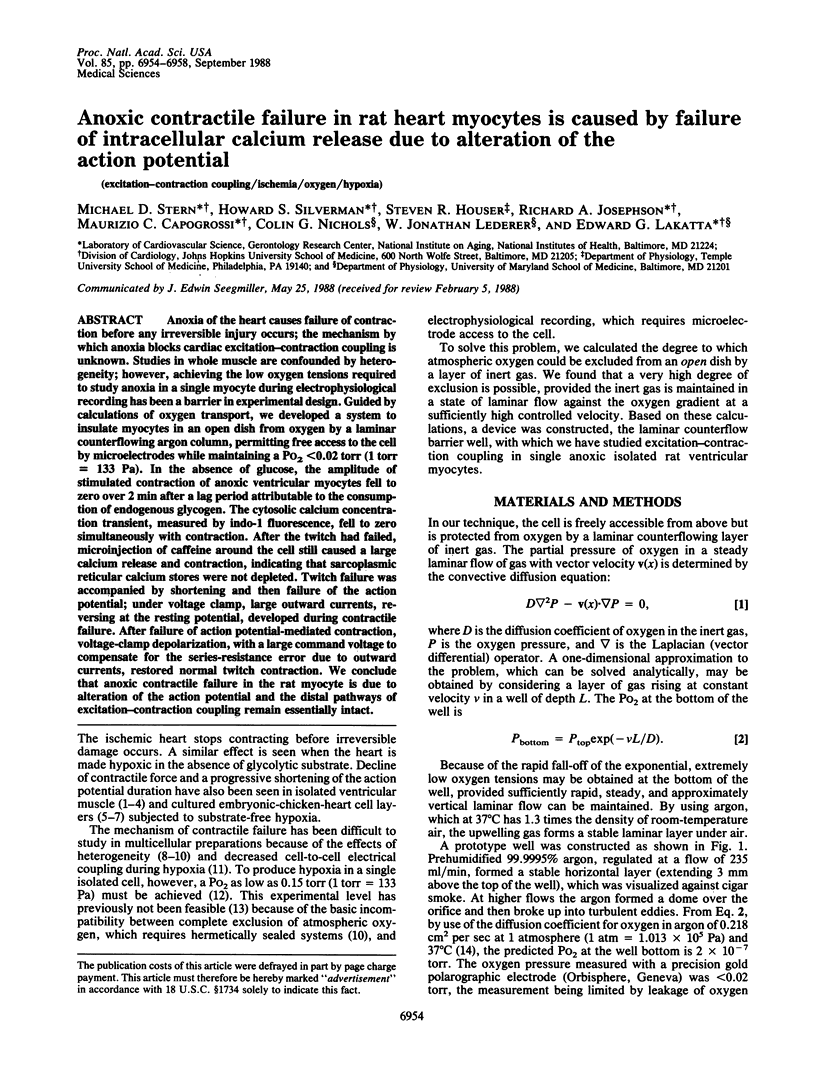
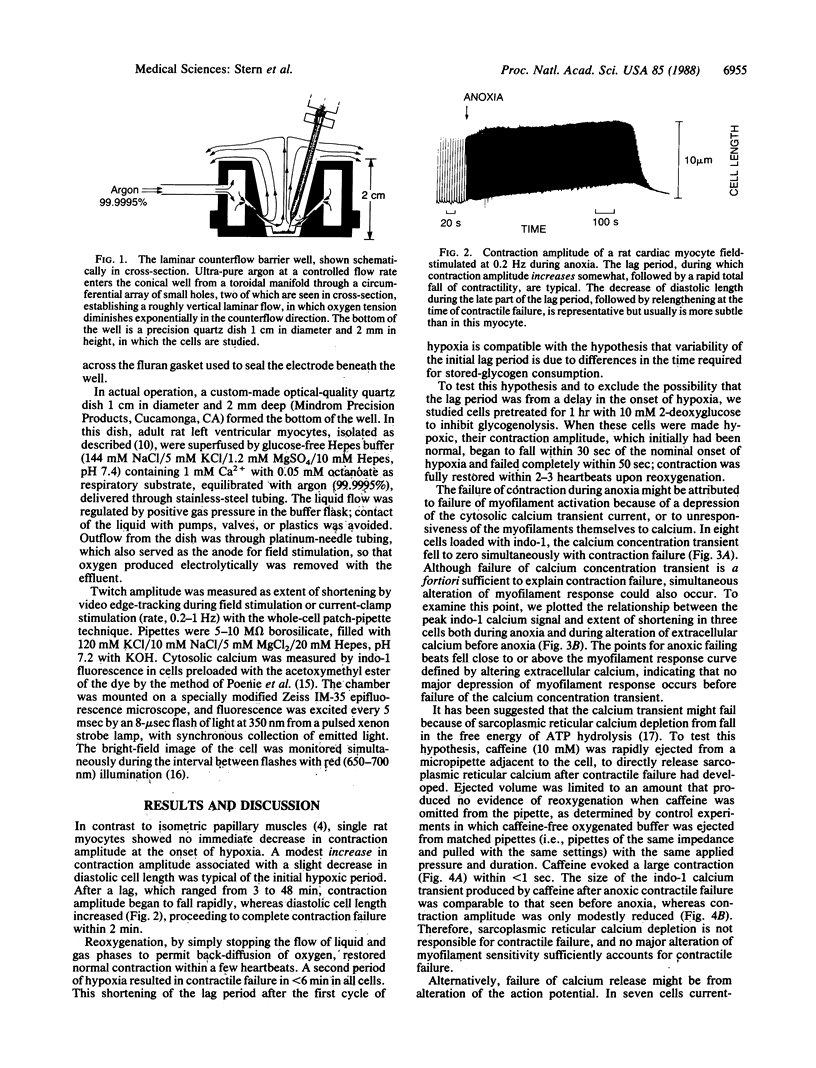
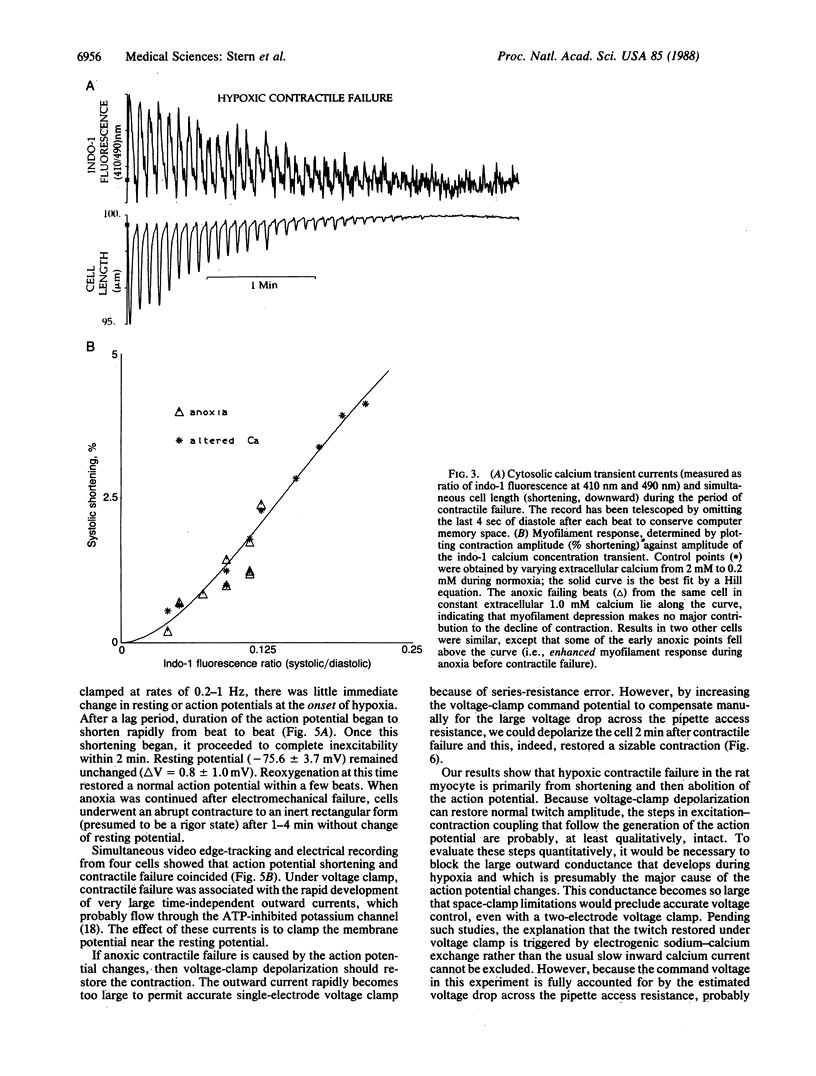
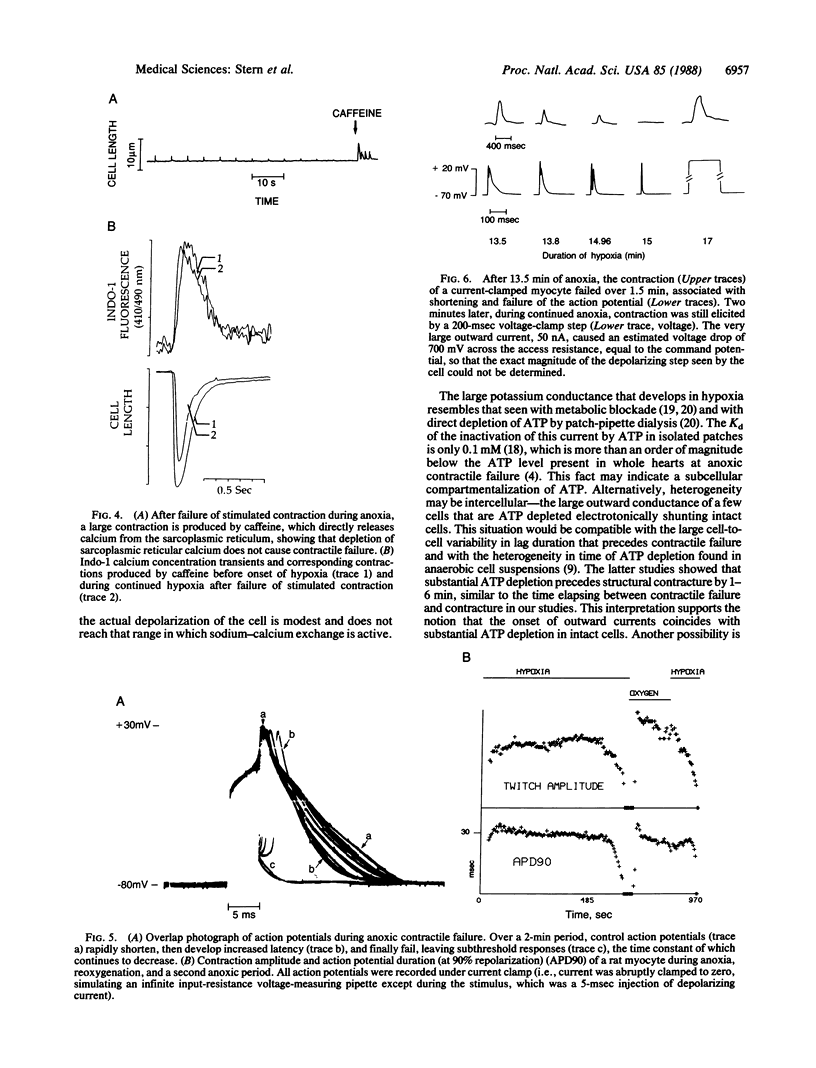
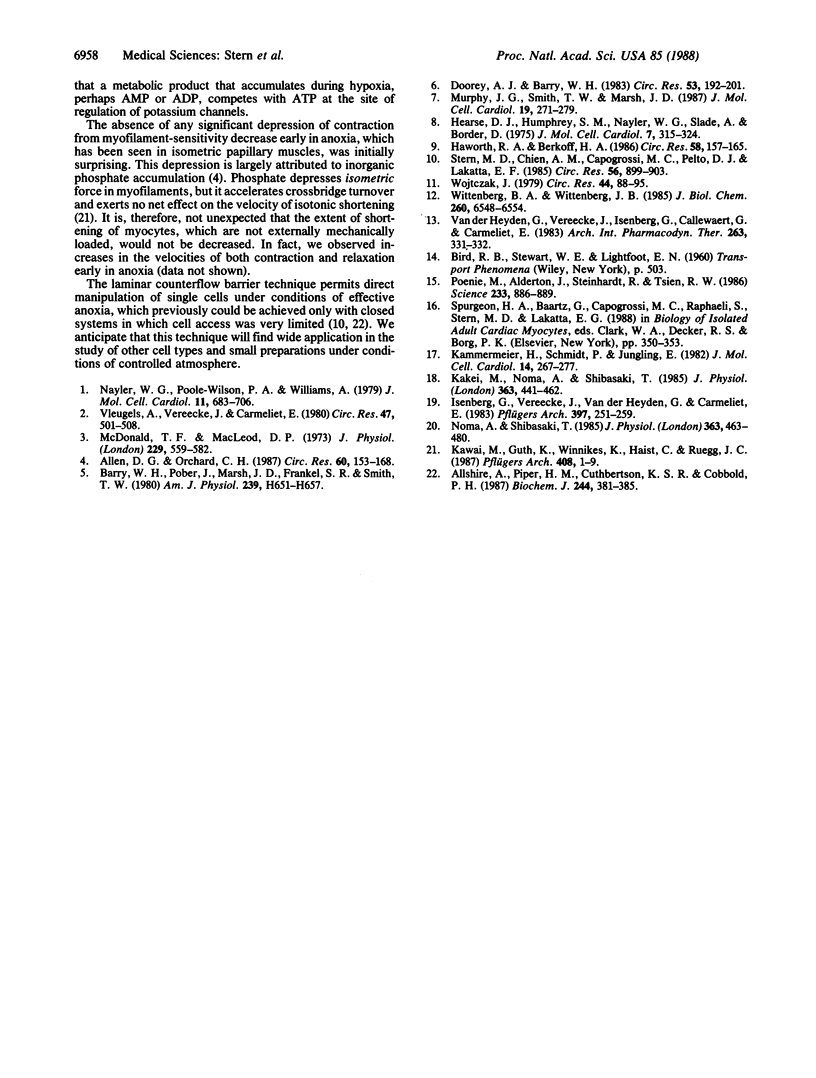
Images in this article
Selected References
These references are in PubMed. This may not be the complete list of references from this article.
- Allen D. G., Orchard C. H. Myocardial contractile function during ischemia and hypoxia. Circ Res. 1987 Feb;60(2):153–168. doi: 10.1161/01.res.60.2.153. [DOI] [PubMed] [Google Scholar]
- Allshire A., Piper H. M., Cuthbertson K. S., Cobbold P. H. Cytosolic free Ca2+ in single rat heart cells during anoxia and reoxygenation. Biochem J. 1987 Jun 1;244(2):381–385. doi: 10.1042/bj2440381. [DOI] [PMC free article] [PubMed] [Google Scholar]
- Barry W. H., Pober J., Marsh J. D., Frankel S. R., Smith T. W. Effects of graded hypoxia on contraction of cultured chick embryo ventricular cells. Am J Physiol. 1980 Nov;239(5):H651–H657. doi: 10.1152/ajpheart.1980.239.5.H651. [DOI] [PubMed] [Google Scholar]
- Doorey A. J., Barry W. H. The effects of inhibition of oxidative phosphorylation and glycolysis on contractility and high-energy phosphate content in cultured chick heart cells. Circ Res. 1983 Aug;53(2):192–201. doi: 10.1161/01.res.53.2.192. [DOI] [PubMed] [Google Scholar]
- Haworth R. A., Berkoff H. A. The control of sugar uptake by metabolic demand in isolated adult rat heart cells. Circ Res. 1986 Jan;58(1):157–165. doi: 10.1161/01.res.58.1.157. [DOI] [PubMed] [Google Scholar]
- Hearse D. J., Humphrey S. M., Nayler W. G., Slade A., Border D. Ultrastructural damage associated with reoxygenation of the anoxic myocardium. J Mol Cell Cardiol. 1975 May;7(5):315–324. doi: 10.1016/0022-2828(75)90121-2. [DOI] [PubMed] [Google Scholar]
- Isenberg G., Vereecke J., van der Heyden G., Carmeliet E. The shortening of the action potential by DNP in guinea-pig ventricular myocytes is mediated by an increase of a time-independent K conductance. Pflugers Arch. 1983 Jun 1;397(4):251–259. doi: 10.1007/BF00580257. [DOI] [PubMed] [Google Scholar]
- Kakei M., Noma A., Shibasaki T. Properties of adenosine-triphosphate-regulated potassium channels in guinea-pig ventricular cells. J Physiol. 1985 Jun;363:441–462. doi: 10.1113/jphysiol.1985.sp015721. [DOI] [PMC free article] [PubMed] [Google Scholar]
- Kammermeier H., Schmidt P., Jüngling E. Free energy change of ATP-hydrolysis: a causal factor of early hypoxic failure of the myocardium? J Mol Cell Cardiol. 1982 May;14(5):267–277. doi: 10.1016/0022-2828(82)90205-x. [DOI] [PubMed] [Google Scholar]
- Kawai M., Güth K., Winnikes K., Haist C., Rüegg J. C. The effect of inorganic phosphate on the ATP hydrolysis rate and the tension transients in chemically skinned rabbit psoas fibers. Pflugers Arch. 1987 Jan;408(1):1–9. doi: 10.1007/BF00581833. [DOI] [PubMed] [Google Scholar]
- McDonald T. F., MacLeod D. P. Metabolism and the electrical activity of anoxic ventricular muscle. J Physiol. 1973 Mar;229(3):559–582. doi: 10.1113/jphysiol.1973.sp010154. [DOI] [PMC free article] [PubMed] [Google Scholar]
- Murphy J. G., Smith T. W., Marsh J. D. Calcium flux measurements during hypoxia in cultured heart cells. J Mol Cell Cardiol. 1987 Mar;19(3):271–279. doi: 10.1016/s0022-2828(87)80594-1. [DOI] [PubMed] [Google Scholar]
- Nayler W. G., Poole-Wilson P. A., Williams A. Hypoxia and calcium. J Mol Cell Cardiol. 1979 Jul;11(7):683–706. doi: 10.1016/0022-2828(79)90381-x. [DOI] [PubMed] [Google Scholar]
- Noma A., Shibasaki T. Membrane current through adenosine-triphosphate-regulated potassium channels in guinea-pig ventricular cells. J Physiol. 1985 Jun;363:463–480. doi: 10.1113/jphysiol.1985.sp015722. [DOI] [PMC free article] [PubMed] [Google Scholar]
- Poenie M., Alderton J., Steinhardt R., Tsien R. Calcium rises abruptly and briefly throughout the cell at the onset of anaphase. Science. 1986 Aug 22;233(4766):886–889. doi: 10.1126/science.3755550. [DOI] [PubMed] [Google Scholar]
- Stern M. D., Chien A. M., Capogrossi M. C., Pelto D. J., Lakatta E. G. Direct observation of the "oxygen paradox" in single rat ventricular myocytes. Circ Res. 1985 Jun;56(6):899–903. doi: 10.1161/01.res.56.6.899. [DOI] [PubMed] [Google Scholar]
- Van der Heyden G., Vereecke J., Isenberg G., Callewaert G., Carmeliet E. Metabolic inhibition and the cardiac ventricular action potential: voltage clamp analysis using single cells. Arch Int Pharmacodyn Ther. 1983 Jun;263(2):331–332. [PubMed] [Google Scholar]
- Vleugels A., Vereecke J., Carmeliet E. Ionic currents during hypoxia in voltage-clamped cat ventricular muscle. Circ Res. 1980 Oct;47(4):501–508. doi: 10.1161/01.res.47.4.501. [DOI] [PubMed] [Google Scholar]
- Wittenberg B. A., Wittenberg J. B. Oxygen pressure gradients in isolated cardiac myocytes. J Biol Chem. 1985 Jun 10;260(11):6548–6554. [PubMed] [Google Scholar]
- Wojtczak J. Contractures and increase in internal longitudianl resistance of cow ventricular muscle induced by hypoxia. Circ Res. 1979 Jan;44(1):88–95. doi: 10.1161/01.res.44.1.88. [DOI] [PubMed] [Google Scholar]