Abstract
The purpose of this study was to investigate whether genetically determined properties of muscle metabolism contribute to the exceptional physical endurance of world-class distance runners. ATP, phosphocreatine, inorganic phosphate, and pH were quantitatively determined by 31P nuclear magnetic resonance spectroscopy in the wrist flexor muscles of elite long-distance runners and sedentary control subjects. These muscles had not been exposed to any specific program of exercise training in either group of subjects. The "untrained" muscles were examined at rest, during two cycles of three grades of exercise, and in recovery. The flexor muscles of the athletes had higher concentrations of phosphocreatine and ATP than did those of the control subjects at rest and during exercise. The athletes' muscles possessed a higher capacity for generation of ATP by oxidative metabolism than did control subjects' muscles according to the following criteria: (i) high force output, 60% of maximum voluntary contraction, was more easily reached and better maintained in both exercise cycles; (ii) the ratio of inorganic phosphate to phosphocreatine rose less during exercise and recovered faster in the postexercise period; (iii) there was no loss of adenine nucleotides or total phosphate from the athletes' muscles but significant losses from the control subjects' muscles; and (iv) the pH decreased no more than 0.1 unit in the athletes' muscles during exercise, attesting to a relatively slow glycolysis and/or a rapid oxidation of lactate. In the muscles of the control subjects, on the other hand, the pH decreased nearly 0.4 unit early in the first exercise cycle, indicating a relatively fast glycolysis and/or slower oxidation of lactate. In the second exercise cycle, the pH returned to near normal in the control subjects' muscles, reflecting diminished lactate formation because of glycogen depletion and lactate washout by the high blood flow induced by exercise. By the end of the exercise program, the maximum voluntary contractile force for the control subjects had declined to less than 60% of the initial value. This decline could be explained best by exhaustion of the glycolytic contribution to muscle contraction. Therefore, the residual maximum strength provided a measure of the oxidative capacity to support contraction, as is discussed. In conclusion, we suggest that a greater oxidative capacity relative to glycolytic capacity for support of contraction in untrained muscle of world-class runners reflects a genetic endowment for physical endurance. Additional systemic effects of training cannot be completely excluded. 31P magnetic resonance spectroscopy provides a noninvasive method for assessing this endowment.
Full text
PDF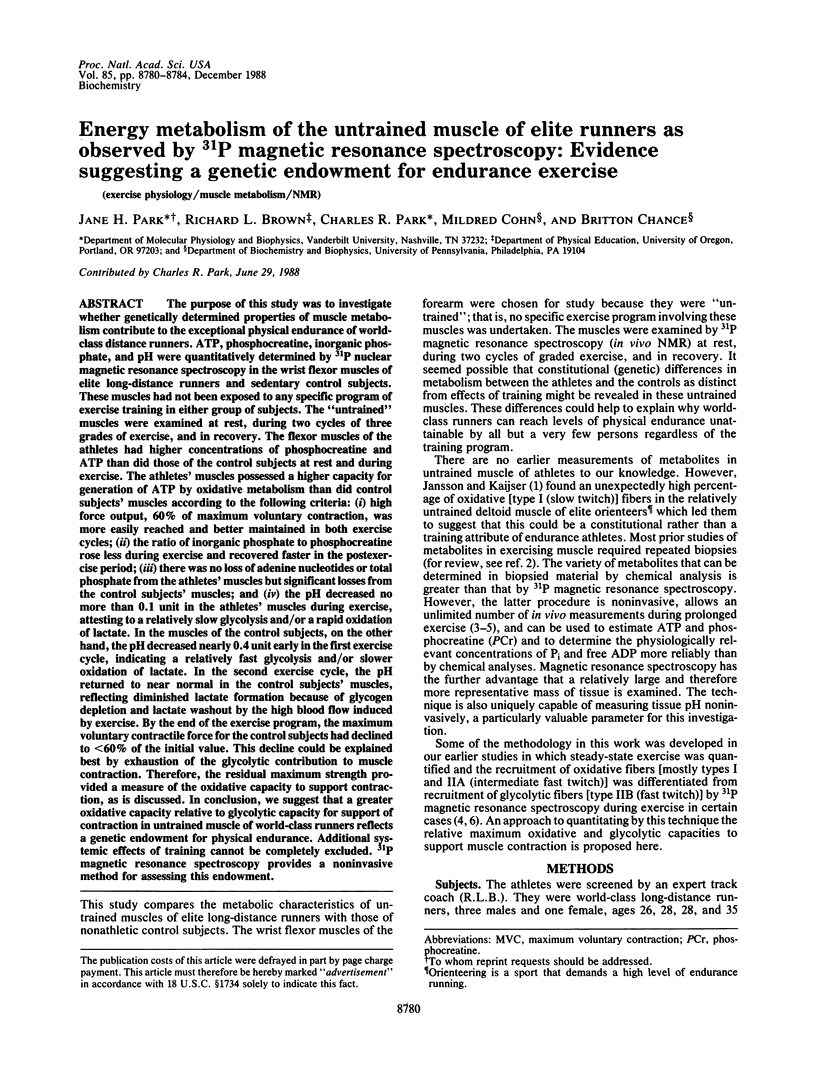
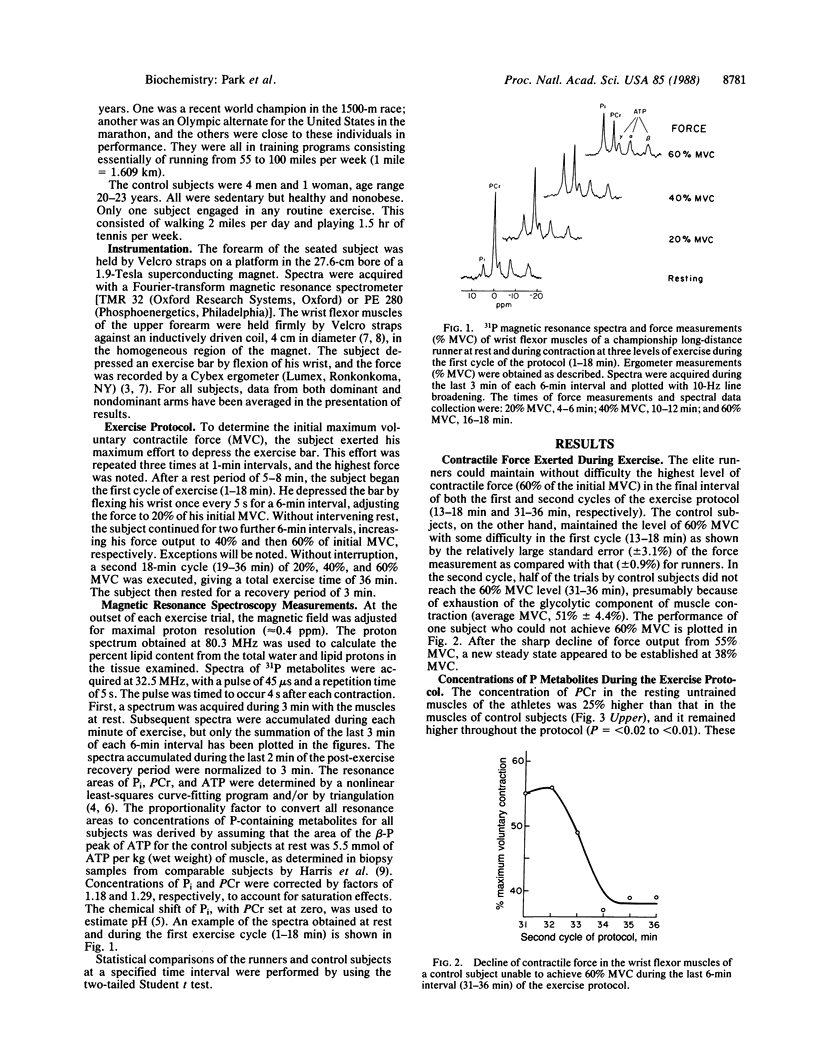
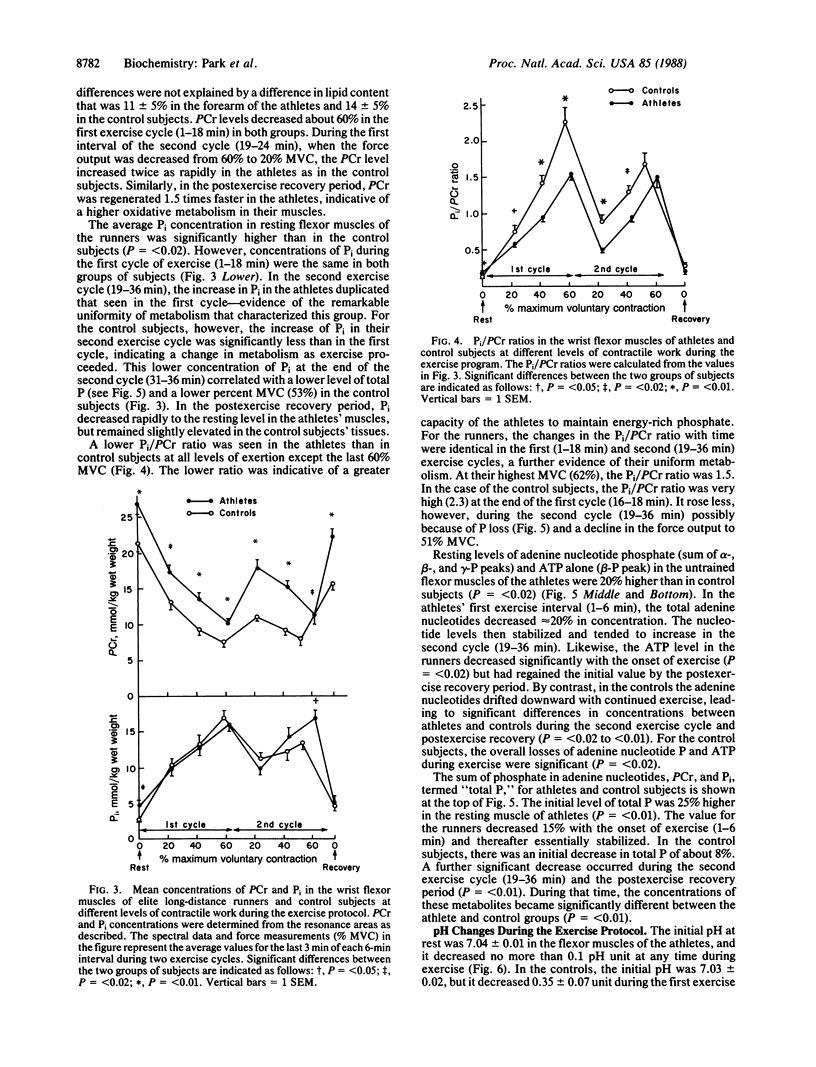
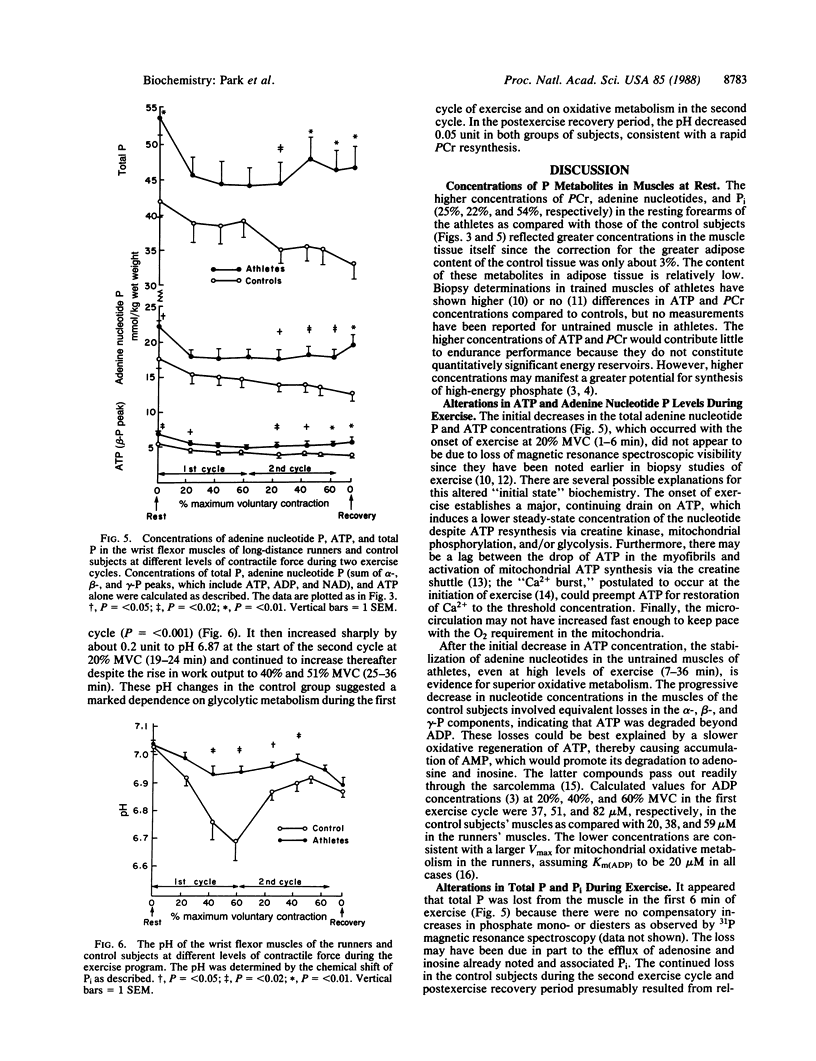
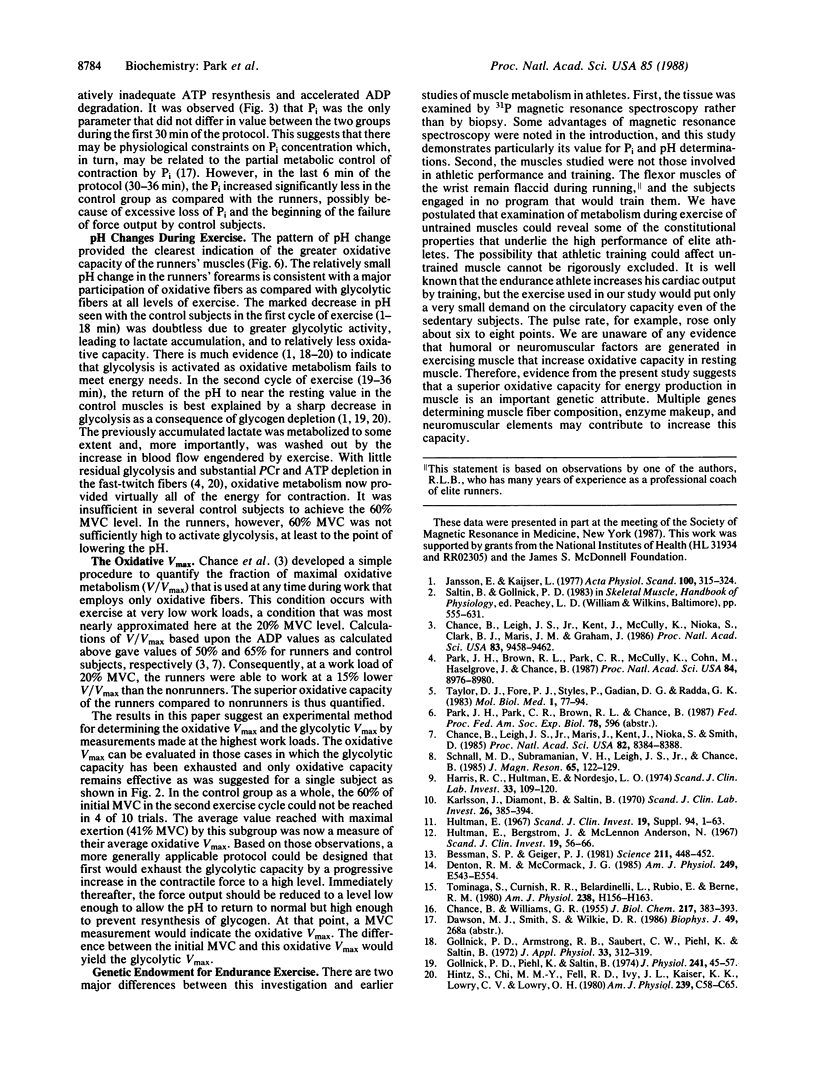
Selected References
These references are in PubMed. This may not be the complete list of references from this article.
- Bessman S. P., Geiger P. J. Transport of energy in muscle: the phosphorylcreatine shuttle. Science. 1981 Jan 30;211(4481):448–452. doi: 10.1126/science.6450446. [DOI] [PubMed] [Google Scholar]
- CHANCE B., WILLIAMS G. R. Respiratory enzymes in oxidative phosphorylation. I. Kinetics of oxygen utilization. J Biol Chem. 1955 Nov;217(1):383–393. [PubMed] [Google Scholar]
- Chance B., Leigh J. S., Jr, Clark B. J., Maris J., Kent J., Nioka S., Smith D. Control of oxidative metabolism and oxygen delivery in human skeletal muscle: a steady-state analysis of the work/energy cost transfer function. Proc Natl Acad Sci U S A. 1985 Dec;82(24):8384–8388. doi: 10.1073/pnas.82.24.8384. [DOI] [PMC free article] [PubMed] [Google Scholar]
- Chance B., Leigh J. S., Jr, Kent J., McCully K., Nioka S., Clark B. J., Maris J. M., Graham T. Multiple controls of oxidative metabolism in living tissues as studied by phosphorus magnetic resonance. Proc Natl Acad Sci U S A. 1986 Dec;83(24):9458–9462. doi: 10.1073/pnas.83.24.9458. [DOI] [PMC free article] [PubMed] [Google Scholar]
- Denton R. M., McCormack J. G. Ca2+ transport by mammalian mitochondria and its role in hormone action. Am J Physiol. 1985 Dec;249(6 Pt 1):E543–E554. doi: 10.1152/ajpendo.1985.249.6.E543. [DOI] [PubMed] [Google Scholar]
- Gollnick P. D., Armstrong R. B., Saubert C. W., 4th, Piehl K., Saltin B. Enzyme activity and fiber composition in skeletal muscle of untrained and trained men. J Appl Physiol. 1972 Sep;33(3):312–319. doi: 10.1152/jappl.1972.33.3.312. [DOI] [PubMed] [Google Scholar]
- Gollnick P. D., Piehl K., Saltin B. Selective glycogen depletion pattern in human muscle fibres after exercise of varying intensity and at varying pedalling rates. J Physiol. 1974 Aug;241(1):45–57. doi: 10.1113/jphysiol.1974.sp010639. [DOI] [PMC free article] [PubMed] [Google Scholar]
- Harris R. C., Hultman E., Nordesjö L. O. Glycogen, glycolytic intermediates and high-energy phosphates determined in biopsy samples of musculus quadriceps femoris of man at rest. Methods and variance of values. Scand J Clin Lab Invest. 1974 Apr;33(2):109–120. [PubMed] [Google Scholar]
- Hintz C. S., Lowry C. V., Kaiser K. K., McKee D., Lowry O. H. Enzyme levels in individual rat muscle fibers. Am J Physiol. 1980 Sep;239(3):C58–C65. doi: 10.1152/ajpcell.1980.239.3.C58. [DOI] [PubMed] [Google Scholar]
- Hultman E., Bergström J., Anderson N. M. Breakdown and resynthesis of phosphorylcreatine and adenosine triphosphate in connection with muscular work in man. Scand J Clin Lab Invest. 1967;19(1):56–66. doi: 10.3109/00365516709093481. [DOI] [PubMed] [Google Scholar]
- Hultman E. Studies on muscle metabolism of glycogen and active phosphate in man with special reference to exercise and diet. Scand J Clin Lab Invest Suppl. 1967;94:1–63. [PubMed] [Google Scholar]
- Jansson E., Kaijser L. Muscle adaptation to extreme endurance training in man. Acta Physiol Scand. 1977 Jul;100(3):315–324. doi: 10.1111/j.1748-1716.1977.tb05956.x. [DOI] [PubMed] [Google Scholar]
- Karlsson J., Diamant B., Saltin B. Muscle metabolites during submaximal and maximal exercise in man. Scand J Clin Lab Invest. 1970 Dec;26(4):385–394. doi: 10.3109/00365517009046250. [DOI] [PubMed] [Google Scholar]
- Park J. H., Brown R. L., Park C. R., McCully K., Cohn M., Haselgrove J., Chance B. Functional pools of oxidative and glycolytic fibers in human muscle observed by 31P magnetic resonance spectroscopy during exercise. Proc Natl Acad Sci U S A. 1987 Dec;84(24):8976–8980. doi: 10.1073/pnas.84.24.8976. [DOI] [PMC free article] [PubMed] [Google Scholar]
- Taylor D. J., Bore P. J., Styles P., Gadian D. G., Radda G. K. Bioenergetics of intact human muscle. A 31P nuclear magnetic resonance study. Mol Biol Med. 1983 Jul;1(1):77–94. [PubMed] [Google Scholar]
- Tominaga S., Curnish R. R., Belardinelli L., Rubio R., Berne R. M. Adenosine release during early and sustained exercise of canine skeletal muscle. Am J Physiol. 1980 Feb;238(2):H156–H163. doi: 10.1152/ajpheart.1980.238.2.H156. [DOI] [PubMed] [Google Scholar]