Abstract
The importance of hydrophobic residues to the binding of methotrexate in the active site of dihydrofolate reductase (EC 1.5.1.3) was examined by a free-energy perturbation method. The replacement of a strictly conserved residue, Phe-31, by tyrosine or valine costs 1.8 and 5.1 kcal/mol, respectively, to the binding of the drug (1 cal = 4.184 J). In the case of the Phe31----Tyr mutation, the loss of the binding energy is due to the desolvation of the phenolic group; in the case of Phe31----Val mutation, it is mainly due to the loss of the interaction with the drug. The replacement of Leu-54 by glycine decreases the binding energy by 4.0 kcal/mol. A calculation on the mutation of Phe-31 to serine shows that the alteration could reduce the binding energy of methotrexate by 9.7 kcal/mol. The calculations clearly show that the hydrophobic interactions are as important as the hydrophilic ones in the binding of methotrexate.
Full text
PDF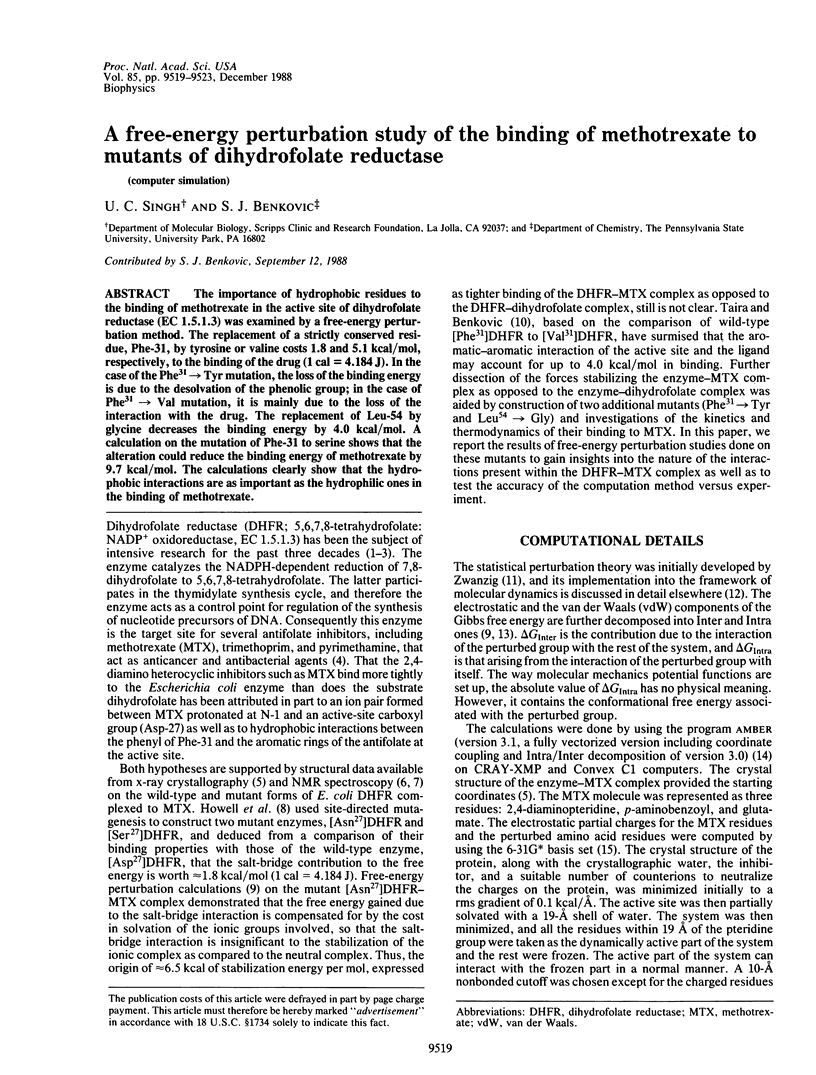
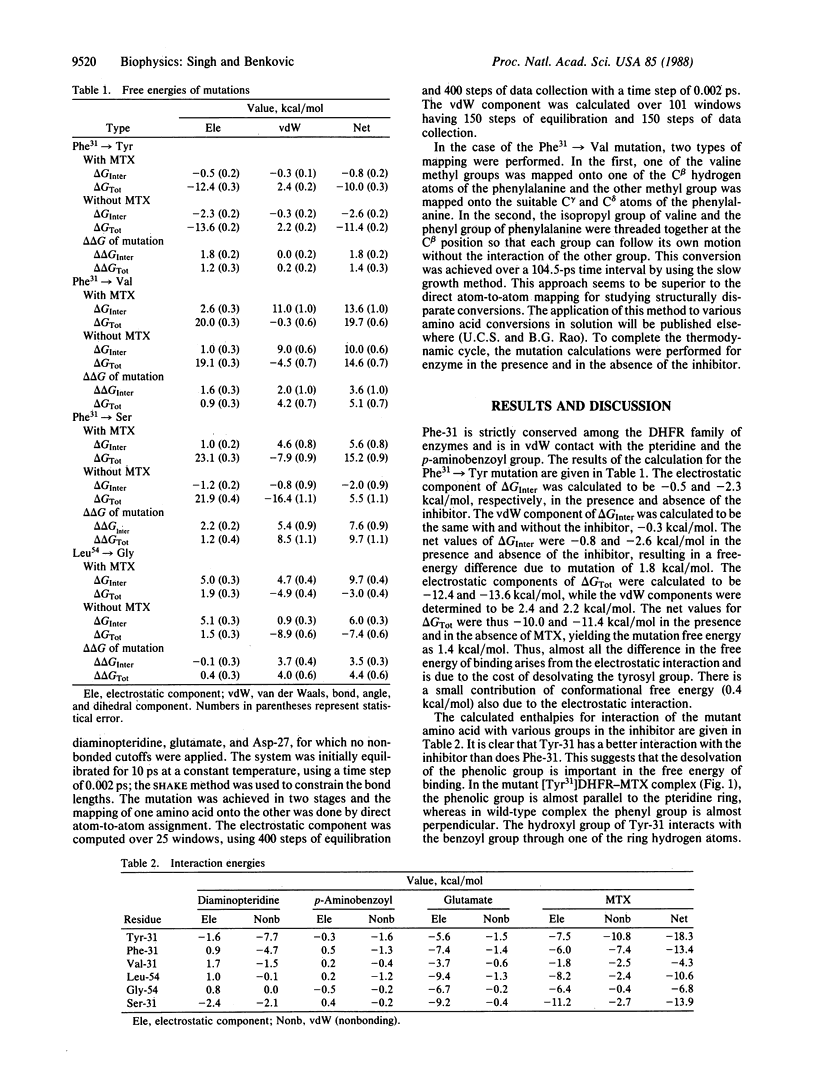
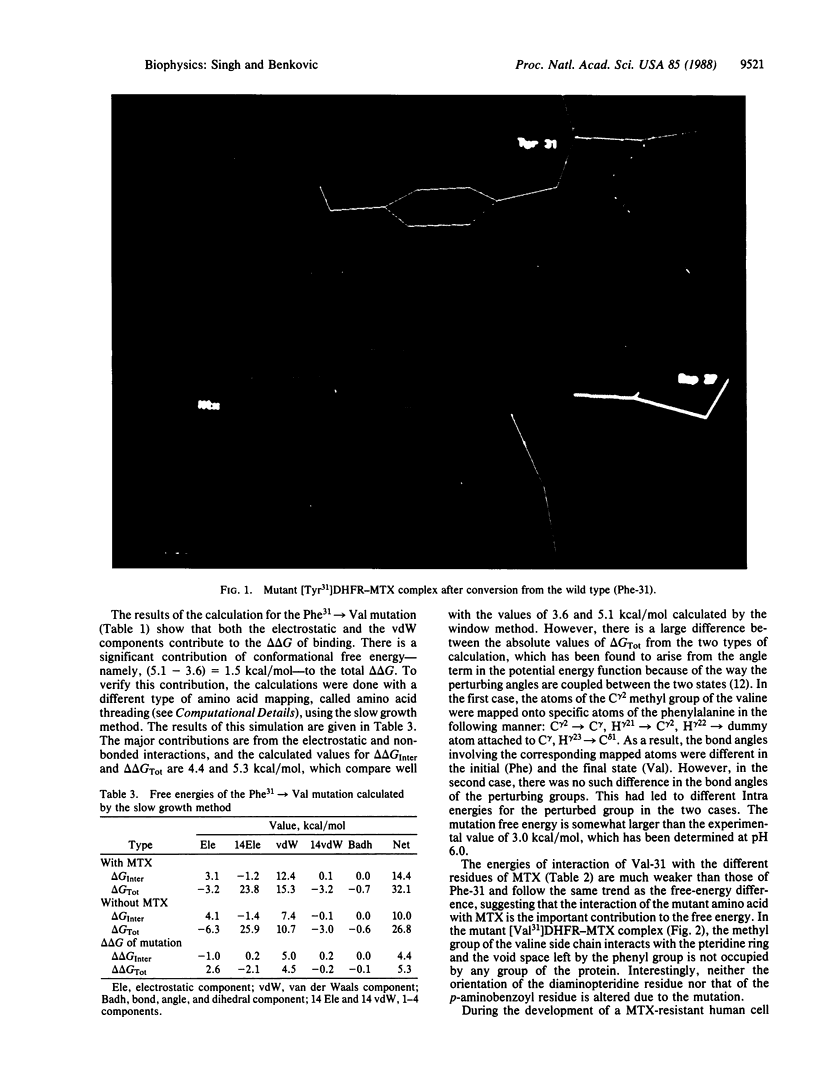
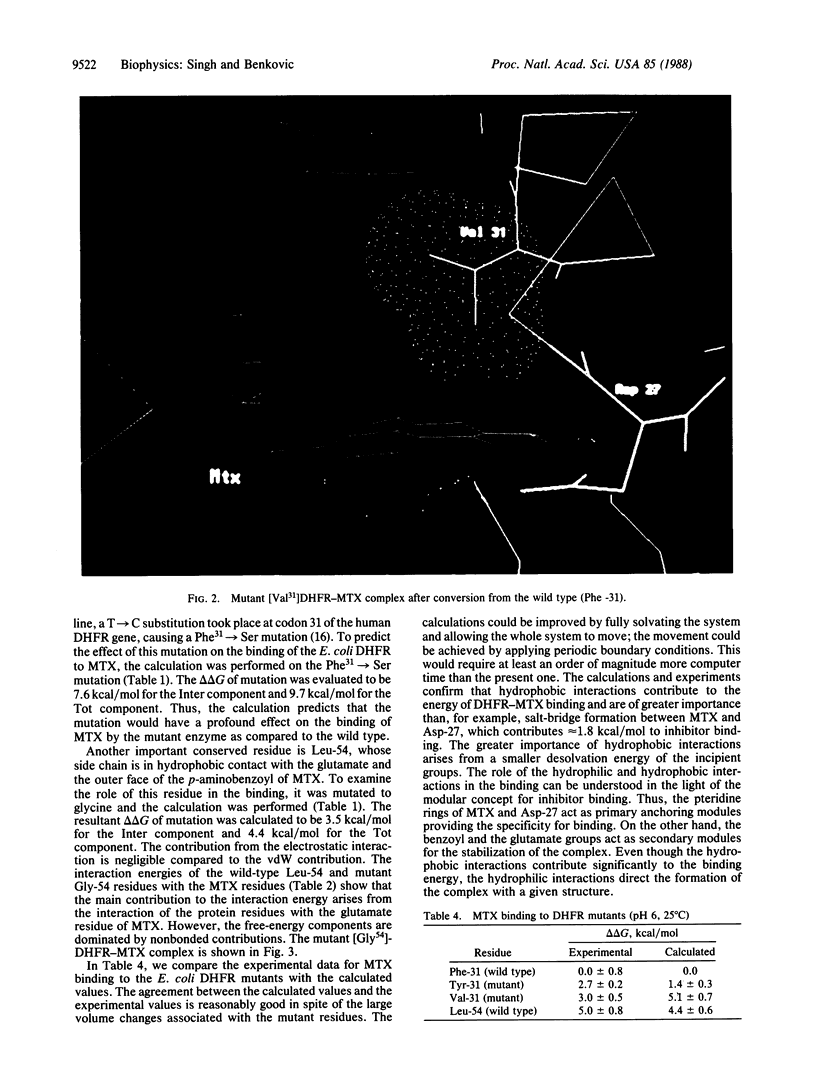
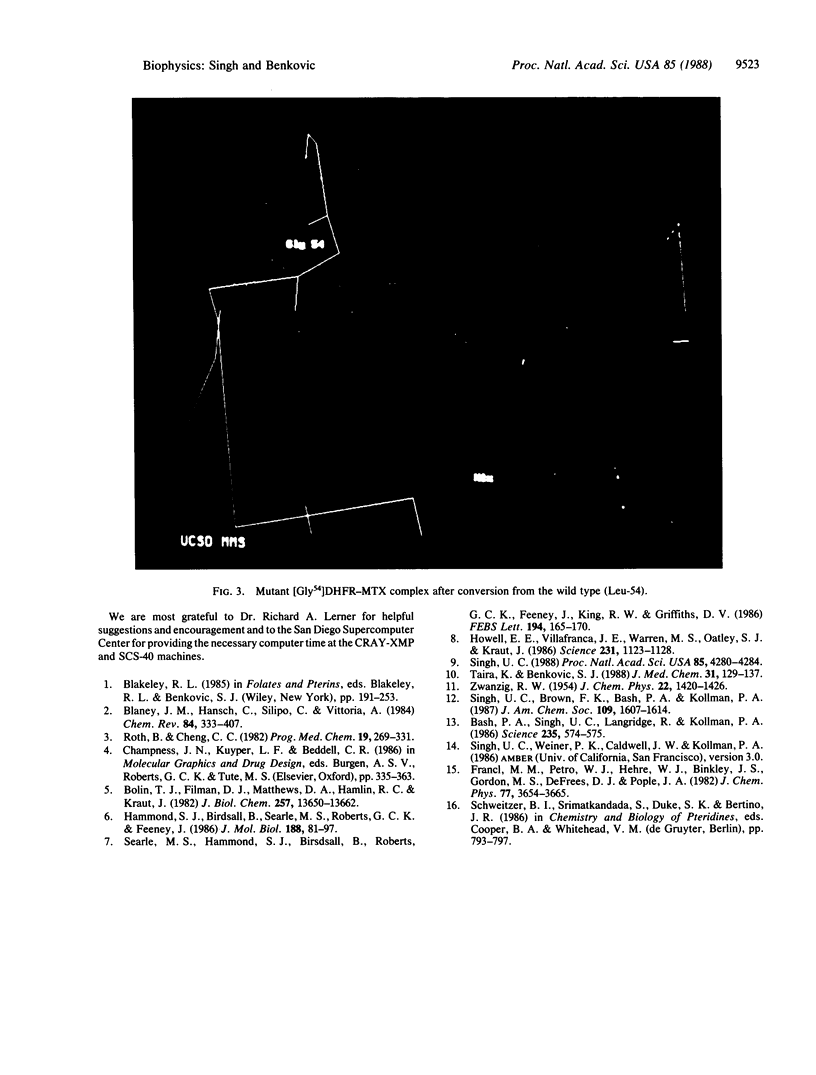
Images in this article
Selected References
These references are in PubMed. This may not be the complete list of references from this article.
- Bash P. A., Singh U. C., Brown F. K., Langridge R., Kollman P. A. Calculation of the relative change in binding free energy of a protein-inhibitor complex. Science. 1987 Jan 30;235(4788):574–576. doi: 10.1126/science.3810157. [DOI] [PubMed] [Google Scholar]
- Bolin J. T., Filman D. J., Matthews D. A., Hamlin R. C., Kraut J. Crystal structures of Escherichia coli and Lactobacillus casei dihydrofolate reductase refined at 1.7 A resolution. I. General features and binding of methotrexate. J Biol Chem. 1982 Nov 25;257(22):13650–13662. [PubMed] [Google Scholar]
- Hammond S. J., Birdsall B., Searle M. S., Roberts G. C., Feeney J. Dihydrofolate reductase. 1H resonance assignments and coenzyme-induced conformational changes. J Mol Biol. 1986 Mar 5;188(1):81–97. doi: 10.1016/0022-2836(86)90483-3. [DOI] [PubMed] [Google Scholar]
- Howell E. E., Villafranca J. E., Warren M. S., Oatley S. J., Kraut J. Functional role of aspartic acid-27 in dihydrofolate reductase revealed by mutagenesis. Science. 1986 Mar 7;231(4742):1123–1128. doi: 10.1126/science.3511529. [DOI] [PubMed] [Google Scholar]
- Roth B., Cheng C. C. Recent progress in the medicinal chemistry of 2,4-diaminopyrimidines. Prog Med Chem. 1982;19:269–331. doi: 10.1016/s0079-6468(08)70332-1. [DOI] [PubMed] [Google Scholar]
- Searle M. S., Hammond S. J., Birdsall B., Roberts G. C., Feeney J., King R. W., Griffiths D. V. Identification of the 1H resonances of valine and leucine residues in dihydrofolate reductase by using a combination of selective deuteration and two-dimensional correlation spectroscopy. FEBS Lett. 1986 Jan 1;194(1):165–170. doi: 10.1016/0014-5793(86)80070-9. [DOI] [PubMed] [Google Scholar]
- Singh U. C. Probing the salt bridge in the dihydrofolate reductase-methotrexate complex by using the coordinate-coupled free-energy perturbation method. Proc Natl Acad Sci U S A. 1988 Jun;85(12):4280–4284. doi: 10.1073/pnas.85.12.4280. [DOI] [PMC free article] [PubMed] [Google Scholar]
- Taira K., Benkovic S. J. Evaluation of the importance of hydrophobic interactions in drug binding to dihydrofolate reductase. J Med Chem. 1988 Jan;31(1):129–137. doi: 10.1021/jm00396a019. [DOI] [PubMed] [Google Scholar]