Abstract
Renal tubular absorption of proline, hydroxyproline, and glycine by the newborn of most mammals is inefficient compared to that of the adult. Cortex slices from seven-day-old rat kidney also transport proline and glycine at reduced initial rates compared to mature kidney. Nonetheless, newborn slices achieve higher intracellular concentrations during prolonged incubation; the latter reflects a reduced rate of efflux, a characteristic peculiar to the membrane of postnatal kidney. The postnatal reduction of initial uptake rates is observed clearly only at substrate concentrations in or below the physiological range; it correlates with the absence of two high-affinity systems which normally serve proline and glycine transport independently at these concentrations in mature kidney, in conjunction with a „common” low-affinity system. The low-affinity system alone performs the observed uptake in the newborn kidney. Specific activity of the high-affinity systems for proline and glycine increases asynchronously after birth, suggesting independent genetic control of the three systems for iminoglycine transport in mammalian kidney.
Full text
PDF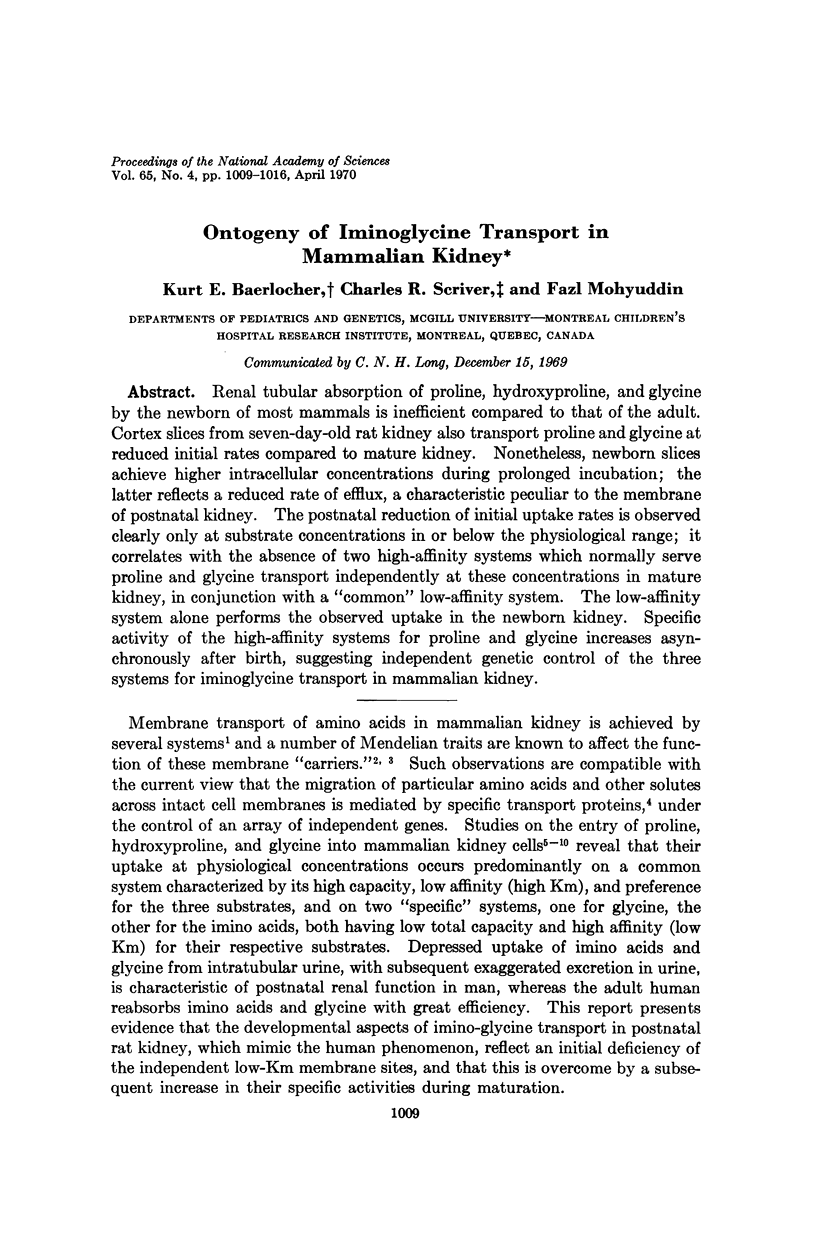
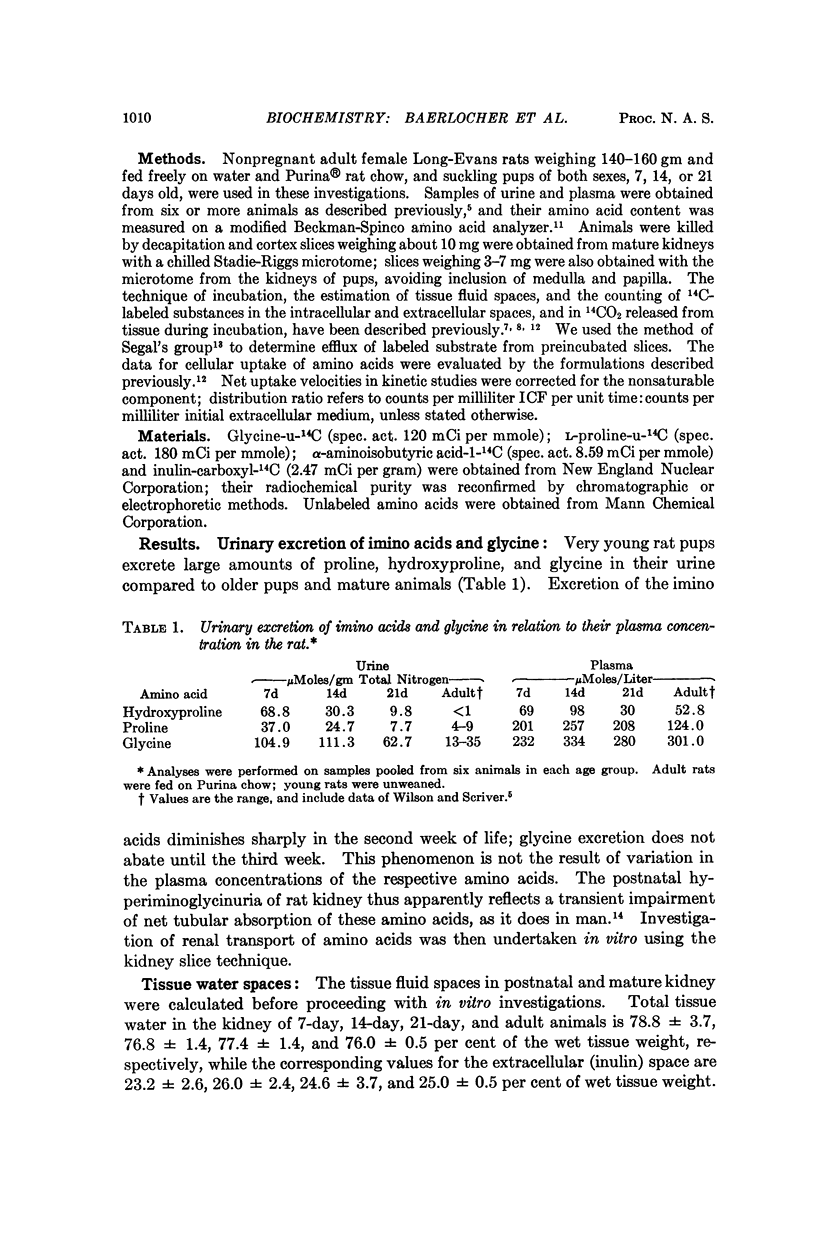
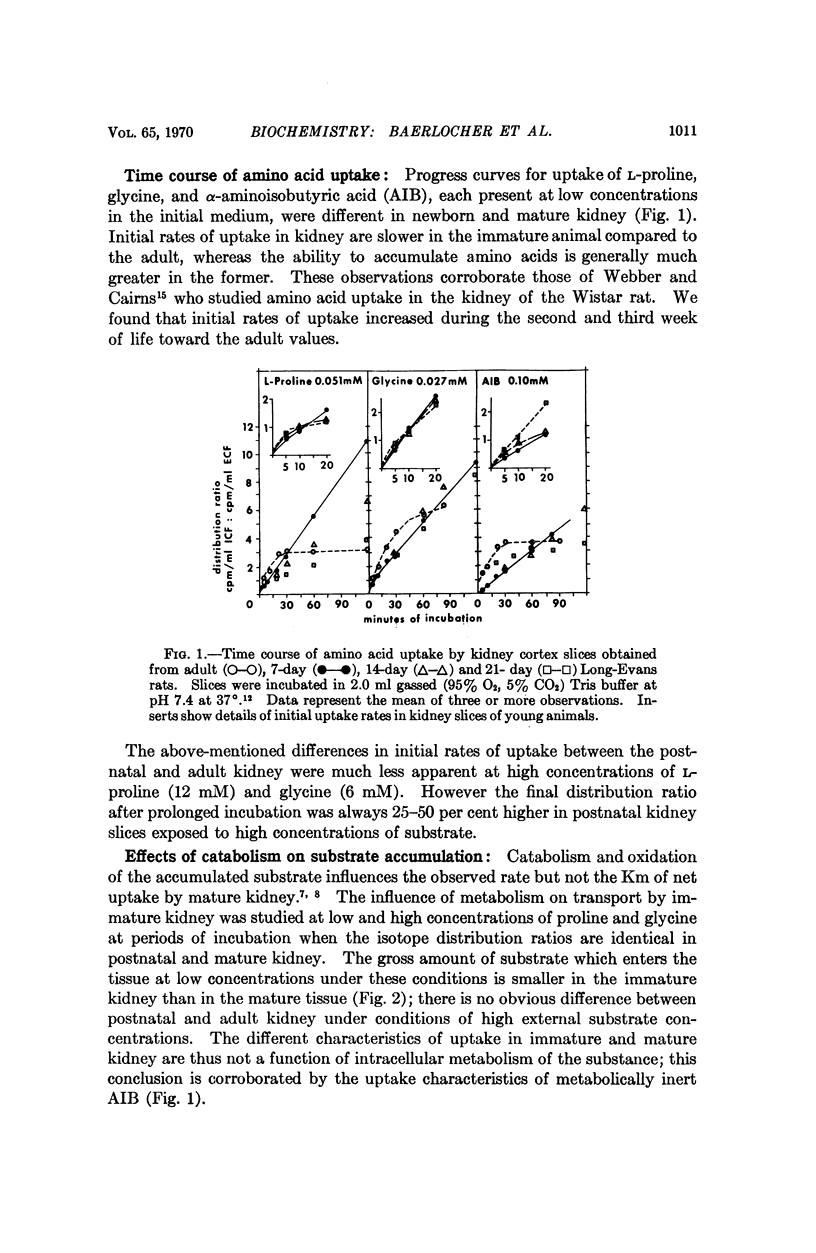
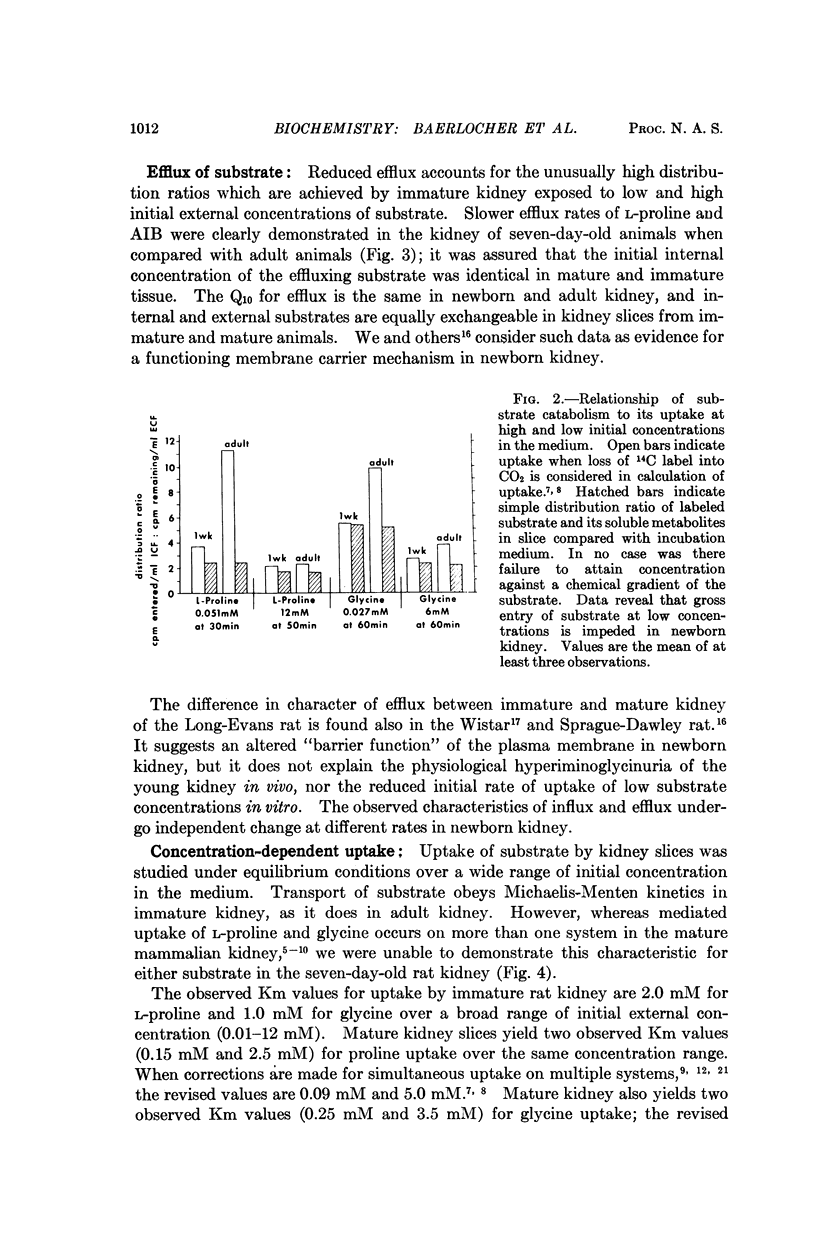
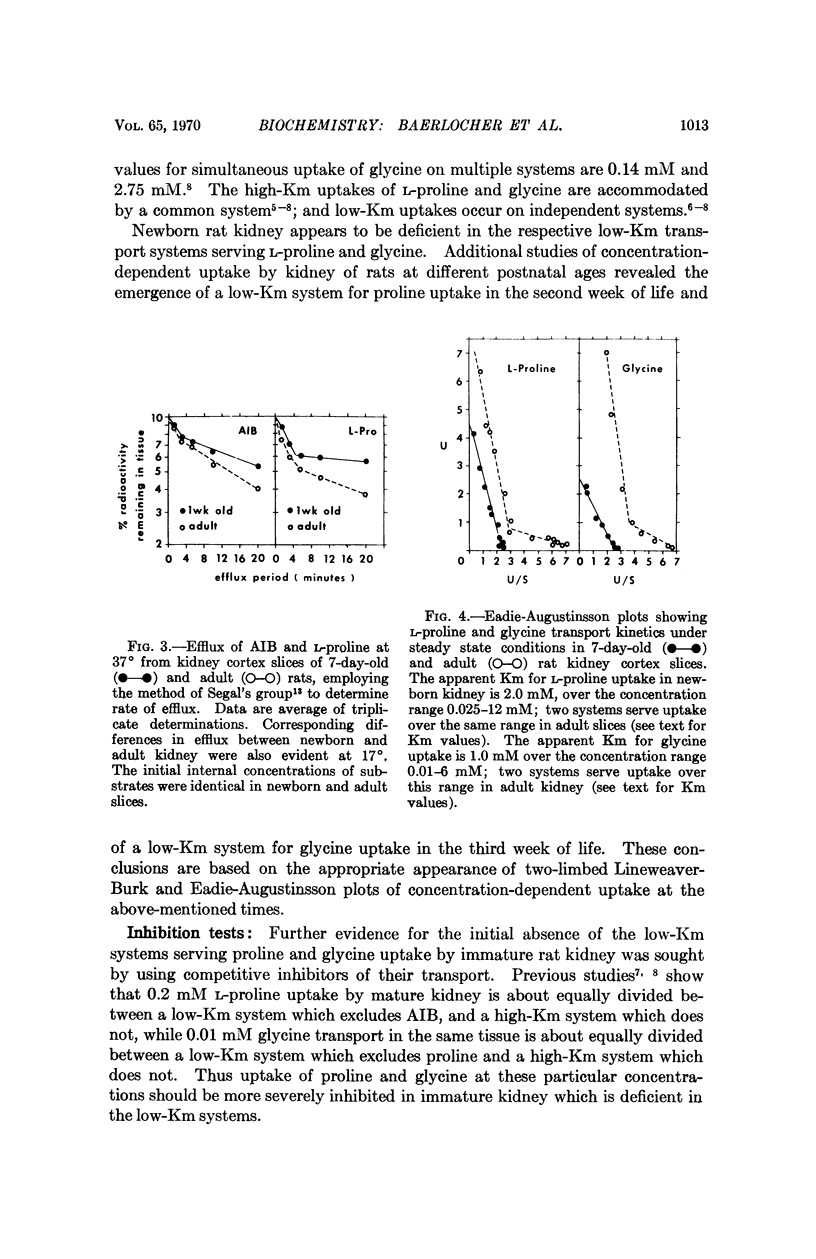
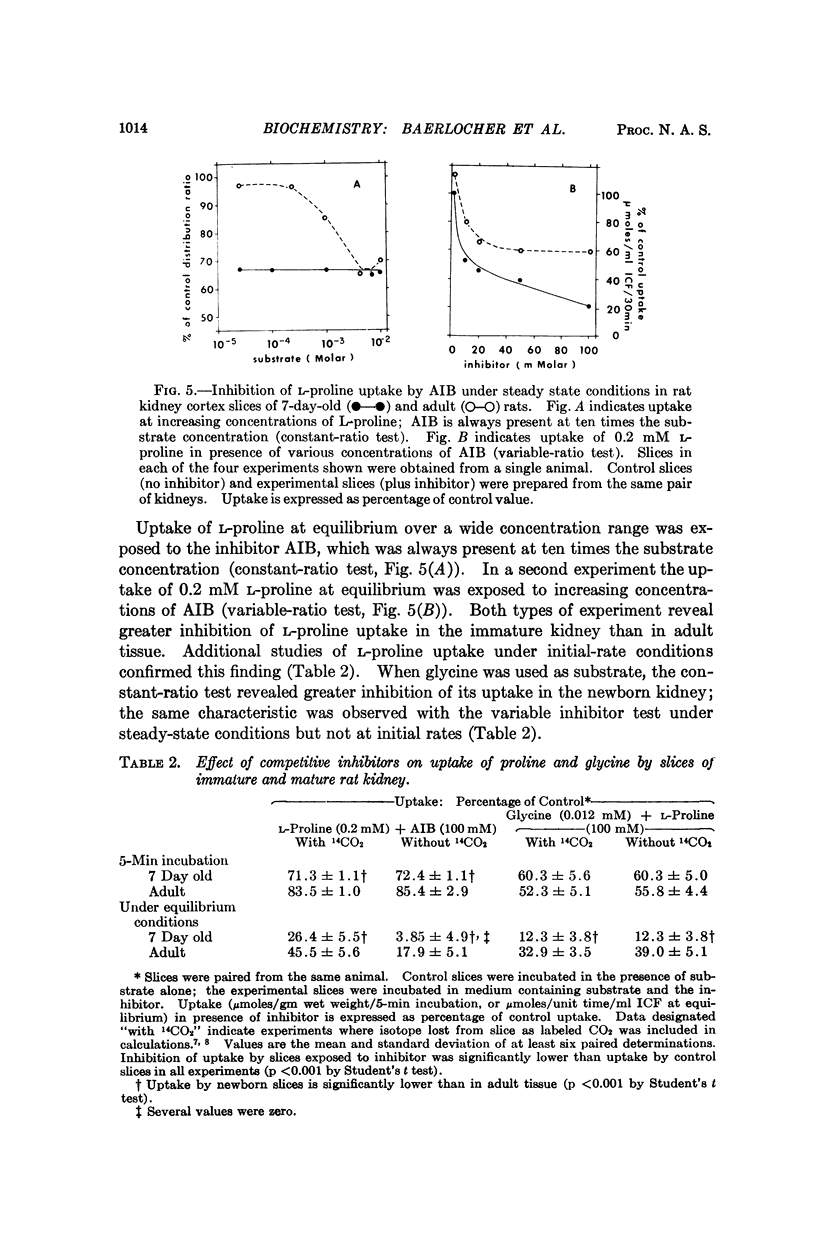
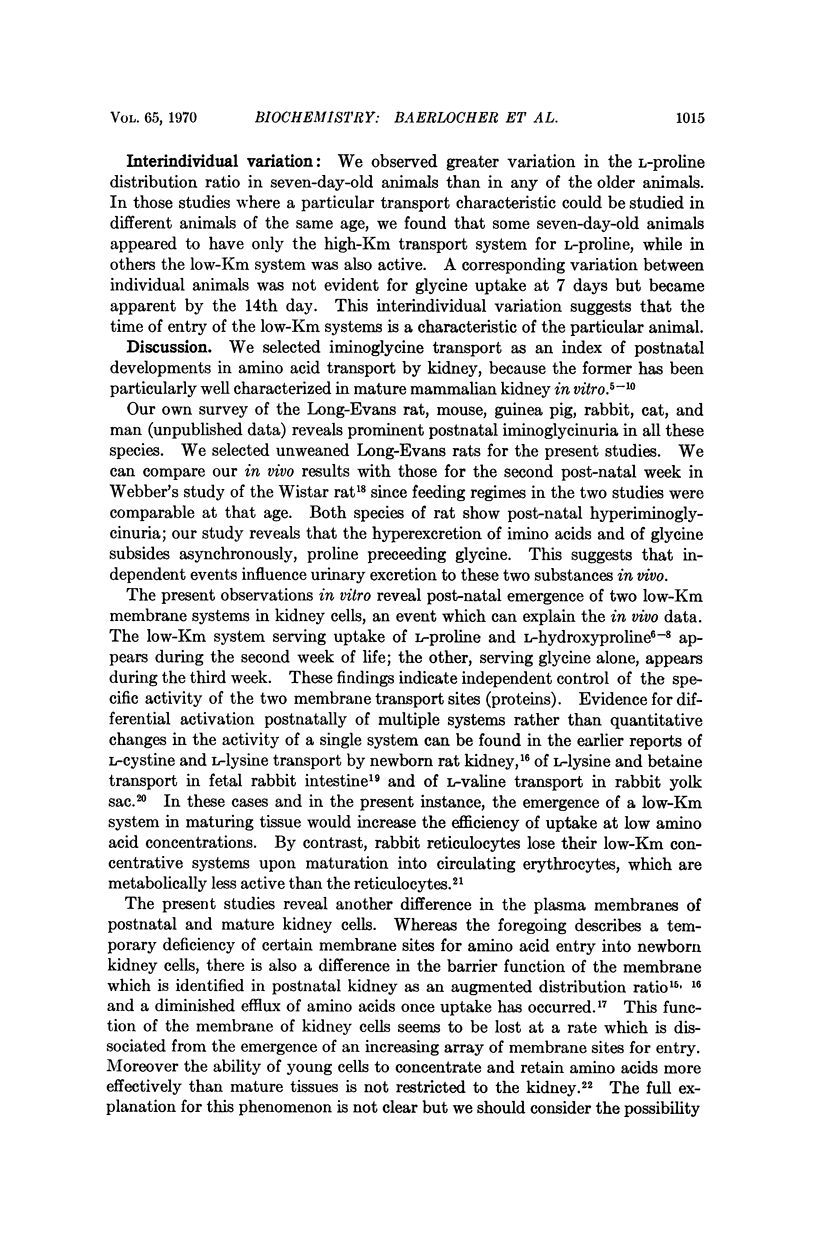
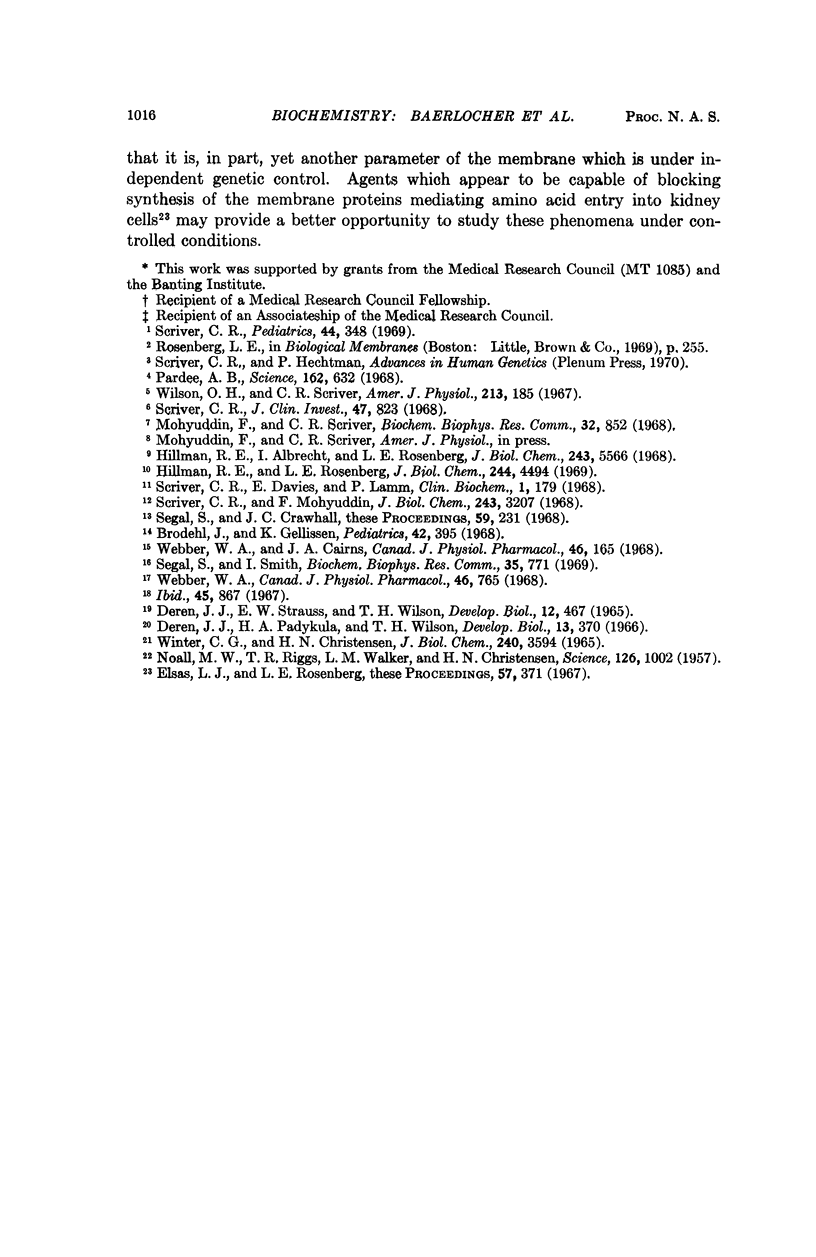
Selected References
These references are in PubMed. This may not be the complete list of references from this article.
- Brodehl J., Gellissen K. Endogenous renal transport of free amino acids in infancy and childhood. Pediatrics. 1968 Sep;42(3):395–404. [PubMed] [Google Scholar]
- Deren J. J., Padykula H. A., Wilson T. H. Development of structure and function in the mammalian yolk sac. 3. The development of amino acid transport by rabbit yolk sac. Dev Biol. 1966 Jun;13(3):370–384. doi: 10.1016/0012-1606(66)90055-8. [DOI] [PubMed] [Google Scholar]
- Deren J. J., Strauss E. W., Wilson T. H. The development of structure and transport systems of the fetal rabbit intestine. Dev Biol. 1965 Dec;12(3):467–486. doi: 10.1016/0012-1606(65)90010-2. [DOI] [PubMed] [Google Scholar]
- Elsas L. J., Rosenberg L. E. Inhibition of amino Acid transport in rat kidney cortex by puromycin. Proc Natl Acad Sci U S A. 1967 Feb;57(2):371–378. doi: 10.1073/pnas.57.2.371. [DOI] [PMC free article] [PubMed] [Google Scholar]
- Hillman R. E., Albrecht I., Rosenberg L. E. Identification and analysis of multiple glycine transport systems in isolated mammalian renal tubules. J Biol Chem. 1968 Nov 10;243(21):5566–5571. [PubMed] [Google Scholar]
- Hillman R. E., Rosenberg L. E. Amino acid transport by isolated mammalian renal tubules. II. Transport systems for L-proline. J Biol Chem. 1969 Aug 25;244(16):4494–4498. [PubMed] [Google Scholar]
- NOALL M. W., RIGGS T. R., WALKER L. M., CHRISTENSEN H. N. Endocrine control of amino acid transfer; distribution of an unmetabolizable amino acid. Science. 1957 Nov 15;126(3281):1002–1005. doi: 10.1126/science.126.3281.1002. [DOI] [PubMed] [Google Scholar]
- Pardee A. B. Membrane transport proteins. Proteins that appear to be parts of membrane transport systems are being isolated and characterized. Science. 1968 Nov 8;162(3854):632–637. doi: 10.1126/science.162.3854.632. [DOI] [PubMed] [Google Scholar]
- Scriver C. R., Mohyuddin F. Amino acid transport in kidney. Heterogeneity of alpha-aminoisobutyric uptake. J Biol Chem. 1968 Jun 25;243(12):3207–3213. [PubMed] [Google Scholar]
- Scriver C. R. Renal tubular transport of proline, hydroxyproline, and glycine. 3. Genetic basis for more than one mode of transport in human kidney. J Clin Invest. 1968 Apr;47(4):823–835. doi: 10.1172/JCI105776. [DOI] [PMC free article] [PubMed] [Google Scholar]
- Scriver C. R. The human biochemical genetics of amino acid transport. Pediatrics. 1969 Sep;44(3):348–357. [PubMed] [Google Scholar]
- Segal S., Crawhall J. C. Characteristics of cystine and cysteine transport in rat kidney cortex slices. Proc Natl Acad Sci U S A. 1968 Jan;59(1):231–237. doi: 10.1073/pnas.59.1.231. [DOI] [PMC free article] [PubMed] [Google Scholar]
- Webber W. A. A comparison of the effux rates of AIB from kidney cortex slices of mature and newborn rats. Can J Physiol Pharmacol. 1968 Sep;46(5):765–769. doi: 10.1139/y68-119. [DOI] [PubMed] [Google Scholar]
- Webber W. A. Amino acid excretion patterns in developing rats. Can J Physiol Pharmacol. 1967 Sep;45(5):867–872. doi: 10.1139/y67-101. [DOI] [PubMed] [Google Scholar]
- Webber W. A., Cairns J. A. A comparison of the amino acid concentrating ability of the kidney cortex of newborn and mature rats. Can J Physiol Pharmacol. 1968 Mar;46(2):165–169. doi: 10.1139/y68-027. [DOI] [PubMed] [Google Scholar]
- Wilson O. H., Scriver C. R. Specificity of transport of neutral and basic amino acids in rat kidney. Am J Physiol. 1967 Jul;213(1):185–190. doi: 10.1152/ajplegacy.1967.213.1.185. [DOI] [PubMed] [Google Scholar]
- Winter C. G., Christensen H. N. Contrasts in neutral amino acid transport by rabbit erythrocytes and reticulocytes. J Biol Chem. 1965 Sep;240(9):3594–3600. [PubMed] [Google Scholar]