Abstract
The pink yeast Rhodotorula rubra of marine origin was found to be capable of extended growth at very low phosphate concentrations (K0.5 = 10.8 nm). Average intracellular phosphate concentrations, based on isotope exchange techniques, were 15 to 200 nm, giving concentration gradients across the cell envelope of about 106. Sensitivity to metabolic inhibitors occurred at micromolar concentrations. Inability of the phosphate transport system, Ks = 0.5 to 2.8 μm, Vmax = 55 μmoles per g of cells per min, to discriminate against arsenate transport led to arsenate toxicity at 1 to 10 nm, whereas environmental arsenate levels are reportedly much higher. Phosphate competitively prevented arsenate toxicity. The Ki for phosphate inhibition of arsenate uptake was 0.7 to 1.2 μm. Phosphate uptake experiments showed that maximal growth rates could be achieved with approximately 4% of the total phosphate-arsenate transport system. Organisms adapted to a range both of concentration of NaCl and of pH. Maximal affinity for phosphate occurred at pH 4 and at low concentrations of NaCl; however, Vmax for phosphate transport was little affected. Maximal specific growth rates on minimal medium were consistent in batch culture but gradually increased to the much higher rates found with yeast extract media when the population was subjected to long-term continuous culture with gradually increasing dilution rates. Phosphate initial uptake rates that were in agreement with the steady-state flux in continuous culture were obtained by using organisms and medium directly from continuous culture. This procedure resulted in rates about 500 times greater than one in which harvested batch-grown cells were used. Discrepancies between values found and those reported in the literature for other organisms were even larger. Growth could not be sustained below a threshold phosphate concentration of 3.4 nm. Such thresholds are explained in terms of a system where growth rate is set by intracellular nutrient concentrations. Threshold concentrations occur in response to nutrient sinks not related to growth, such as efflux and endogenous metabolism. Equations are presented for evaluation of growth rate-limiting substrate concentrations in the presence of background substrate and for evaluating low inhibitor concentration inhibition mechanisms by substrate prevention of inhibitor flux.
Full text
PDF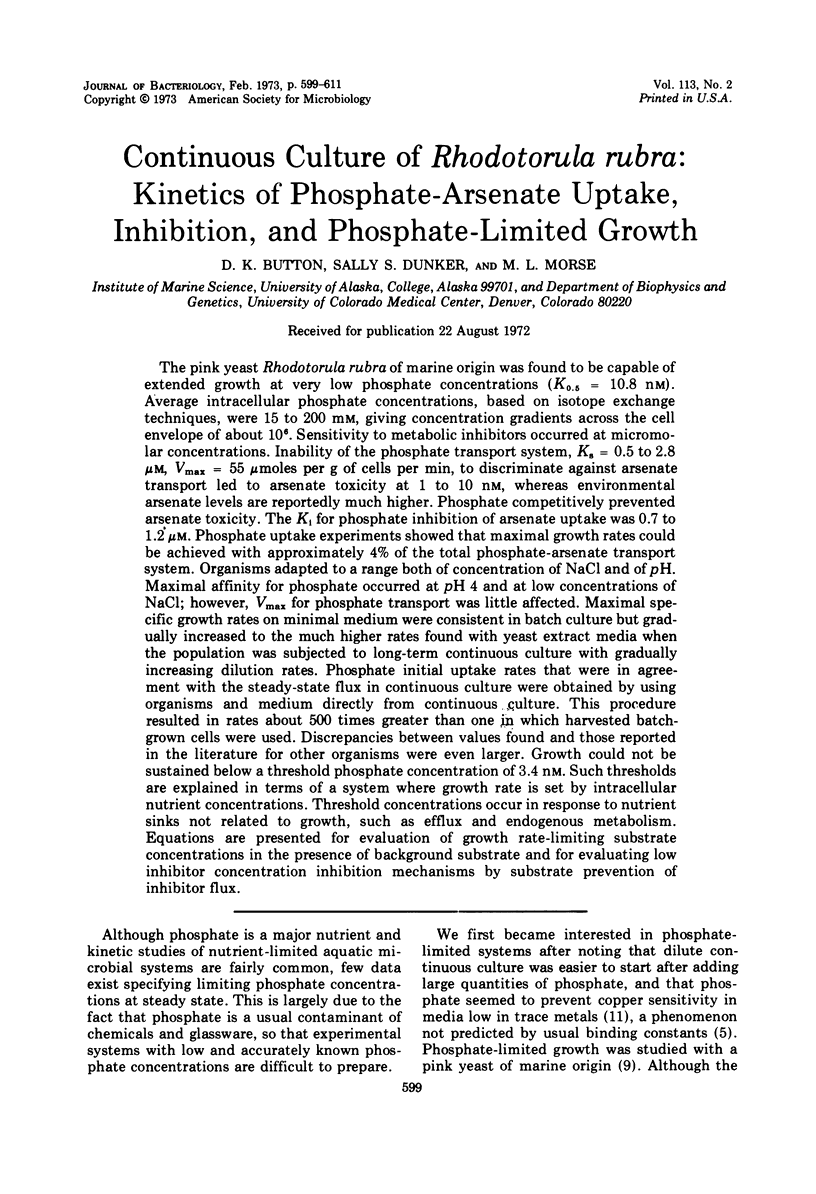
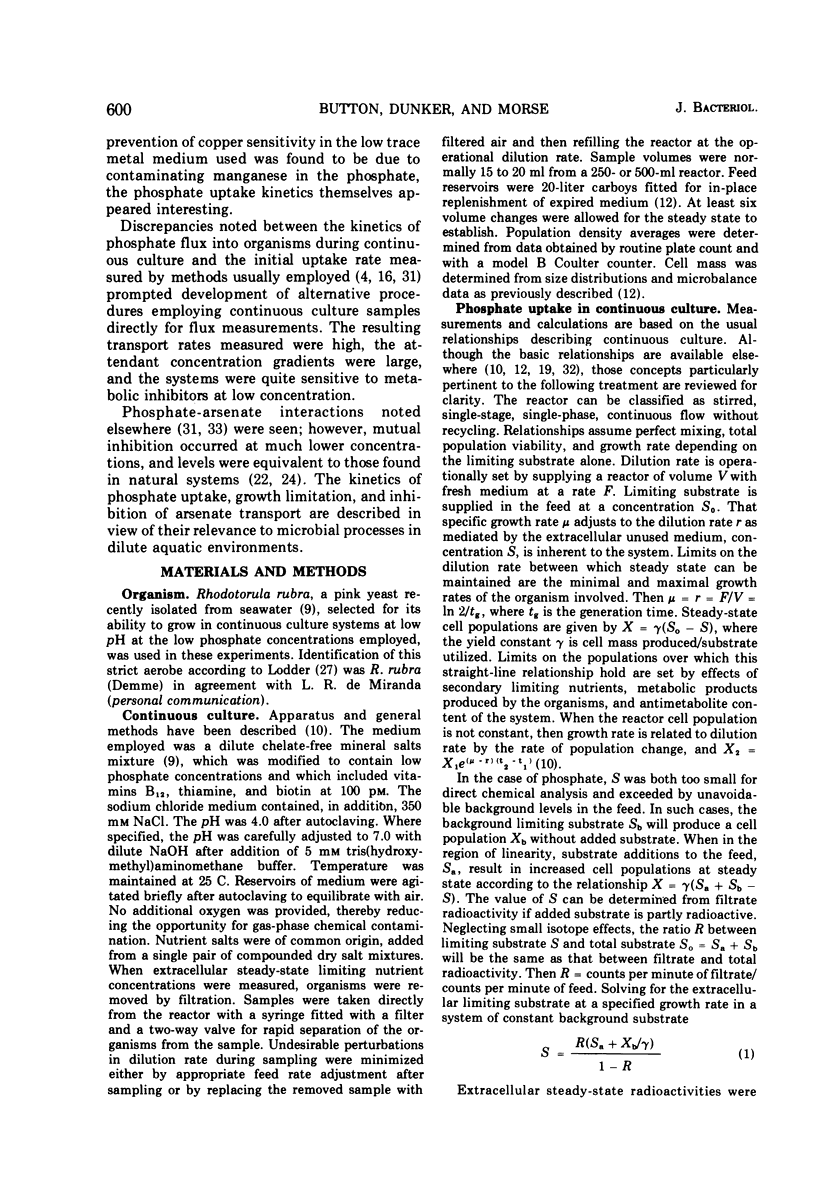
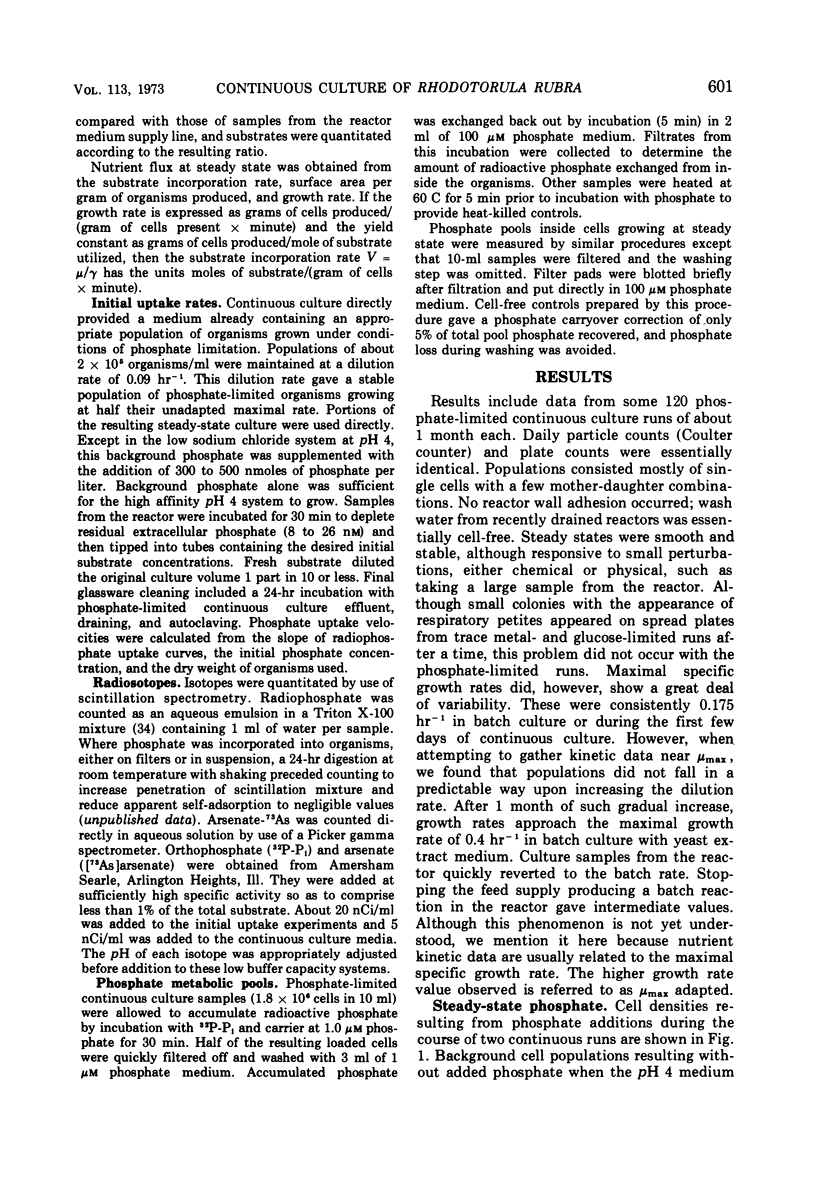
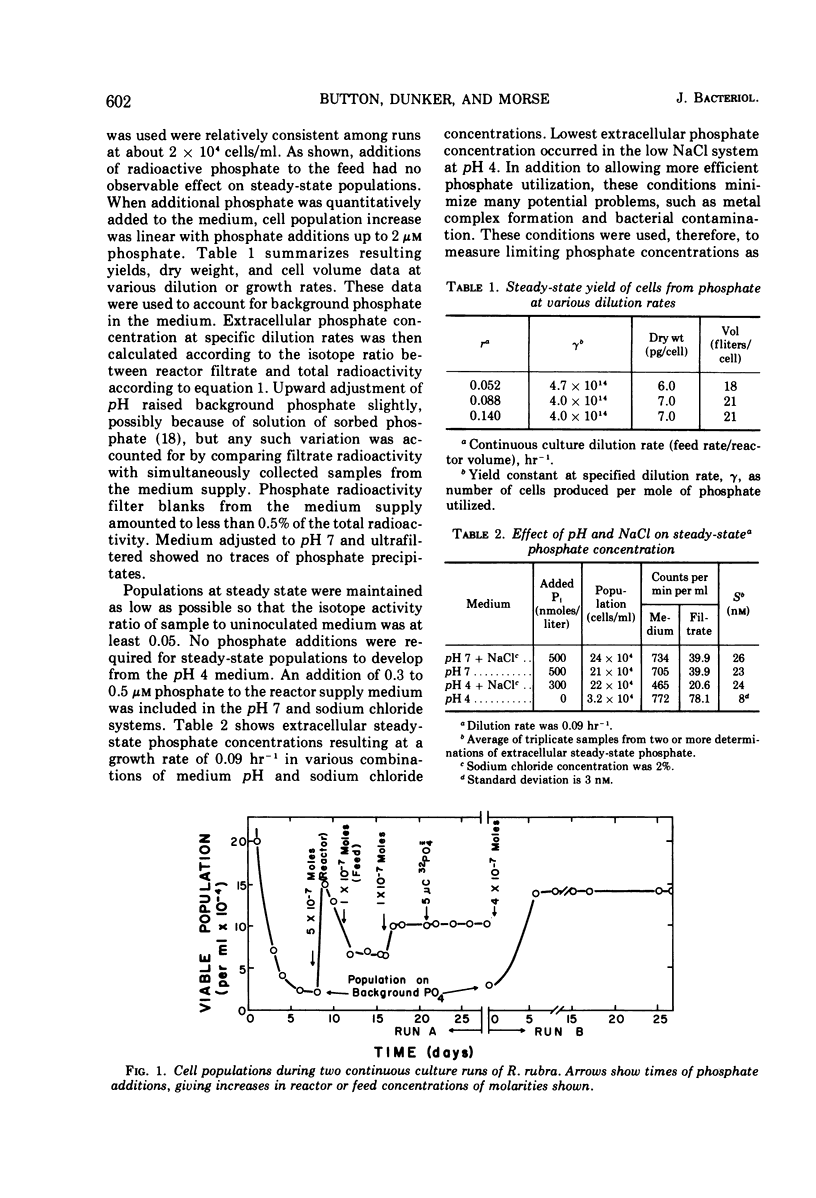
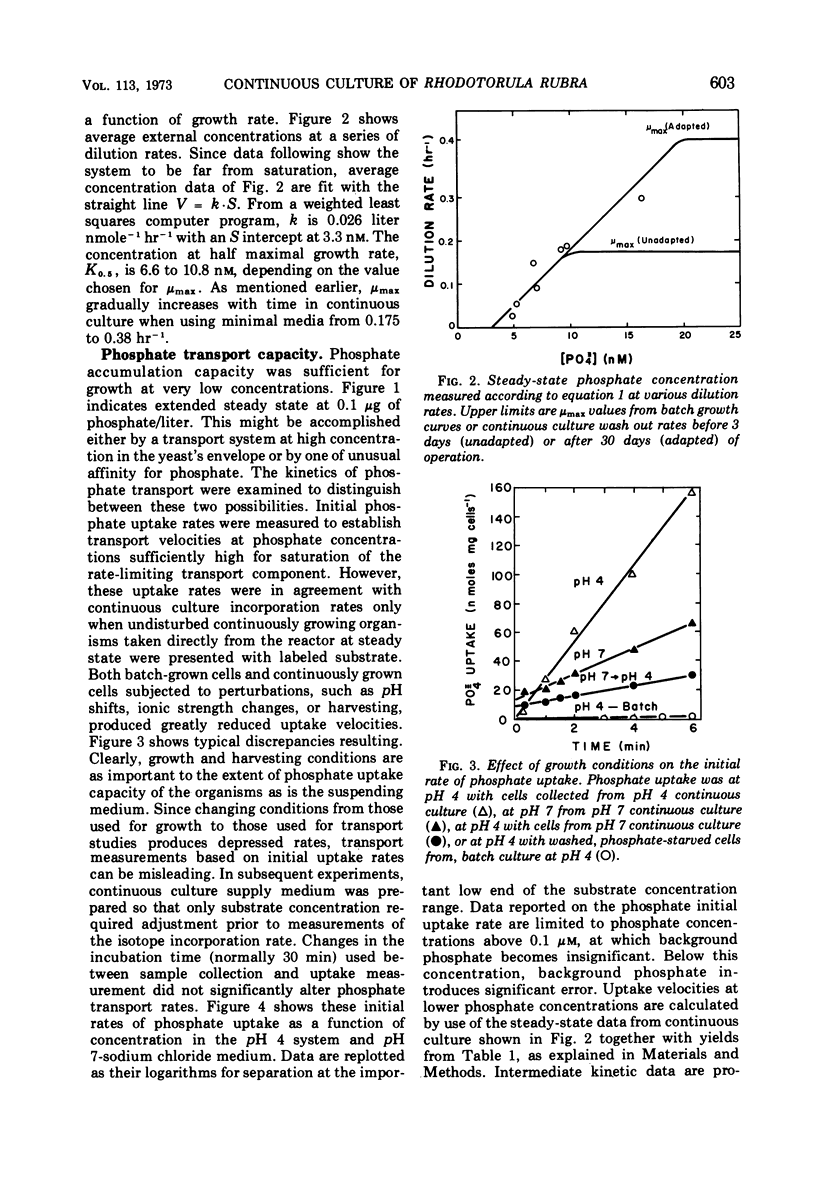
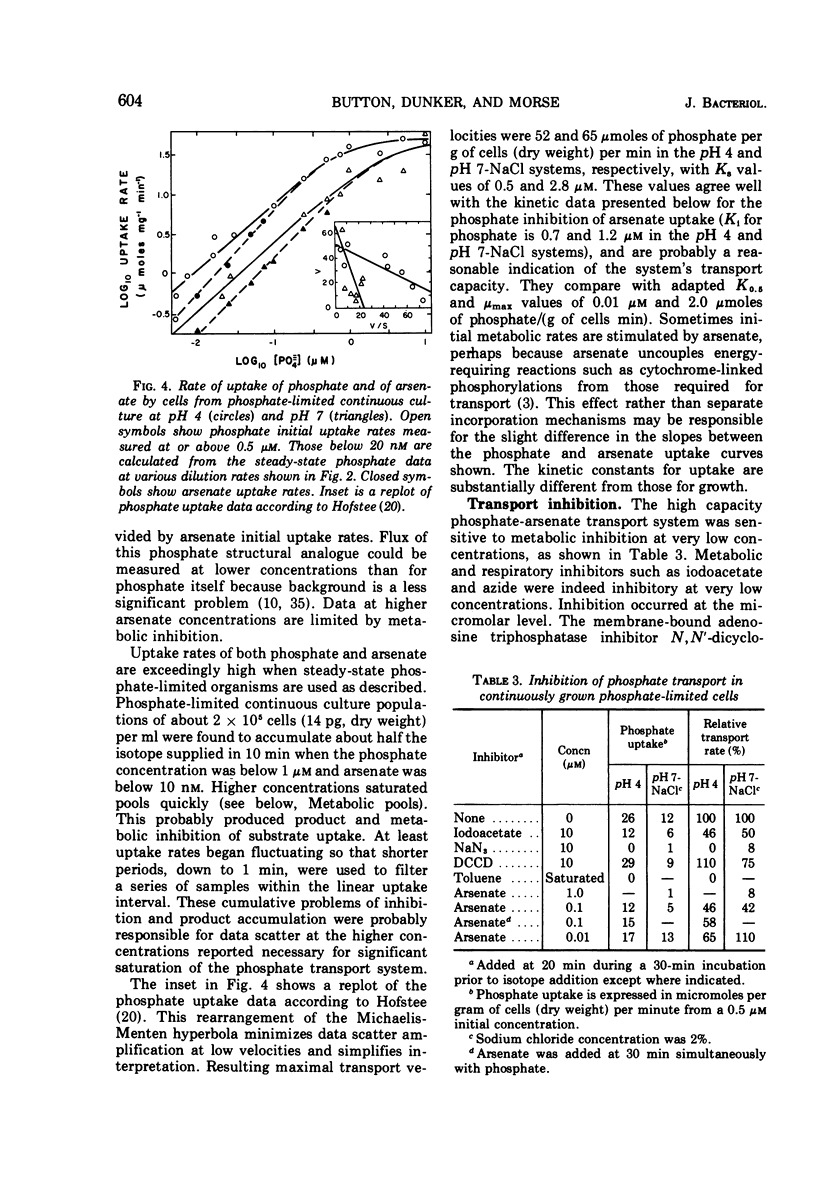
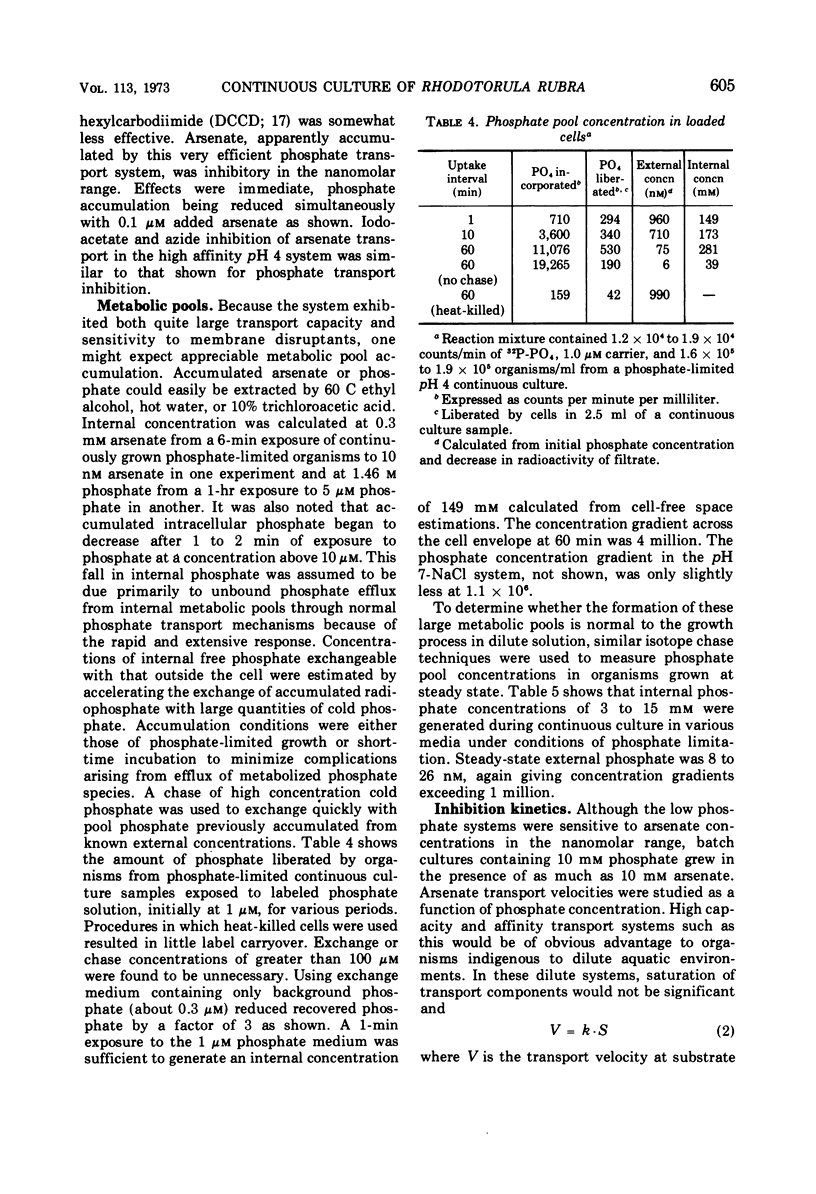
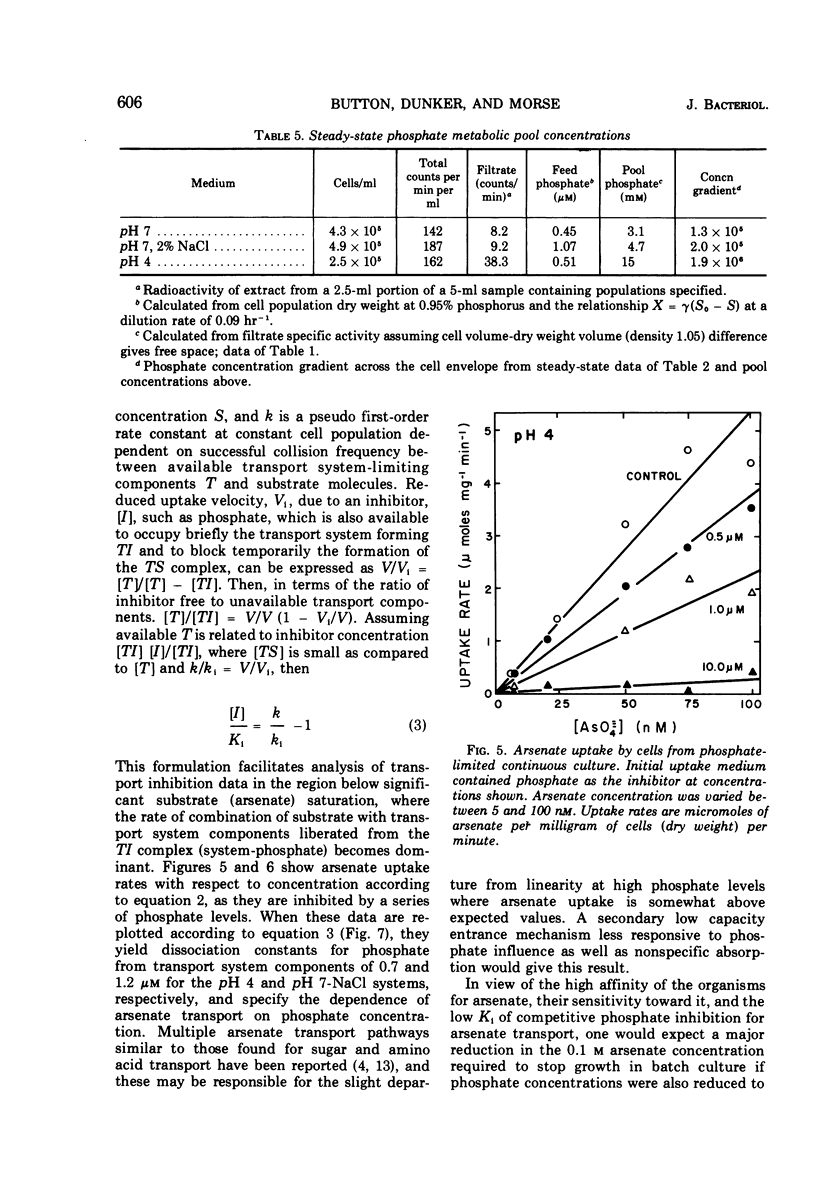
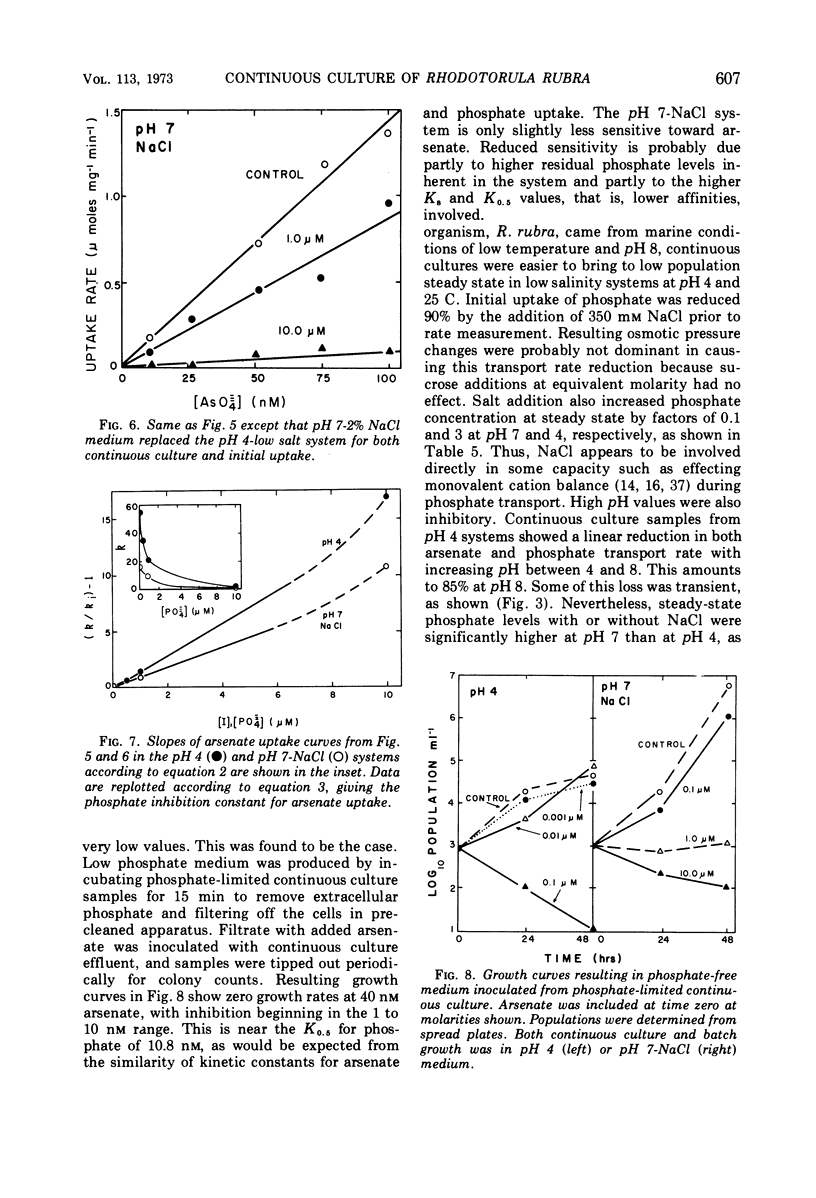
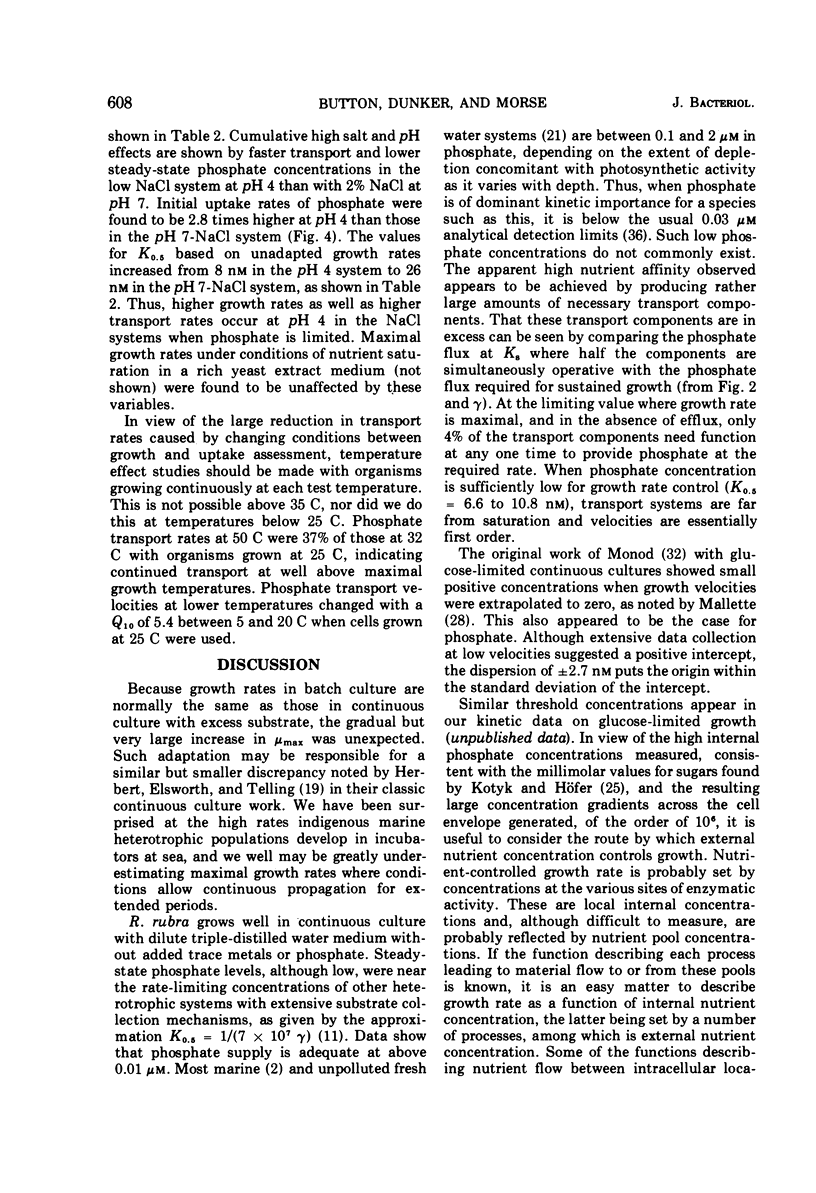
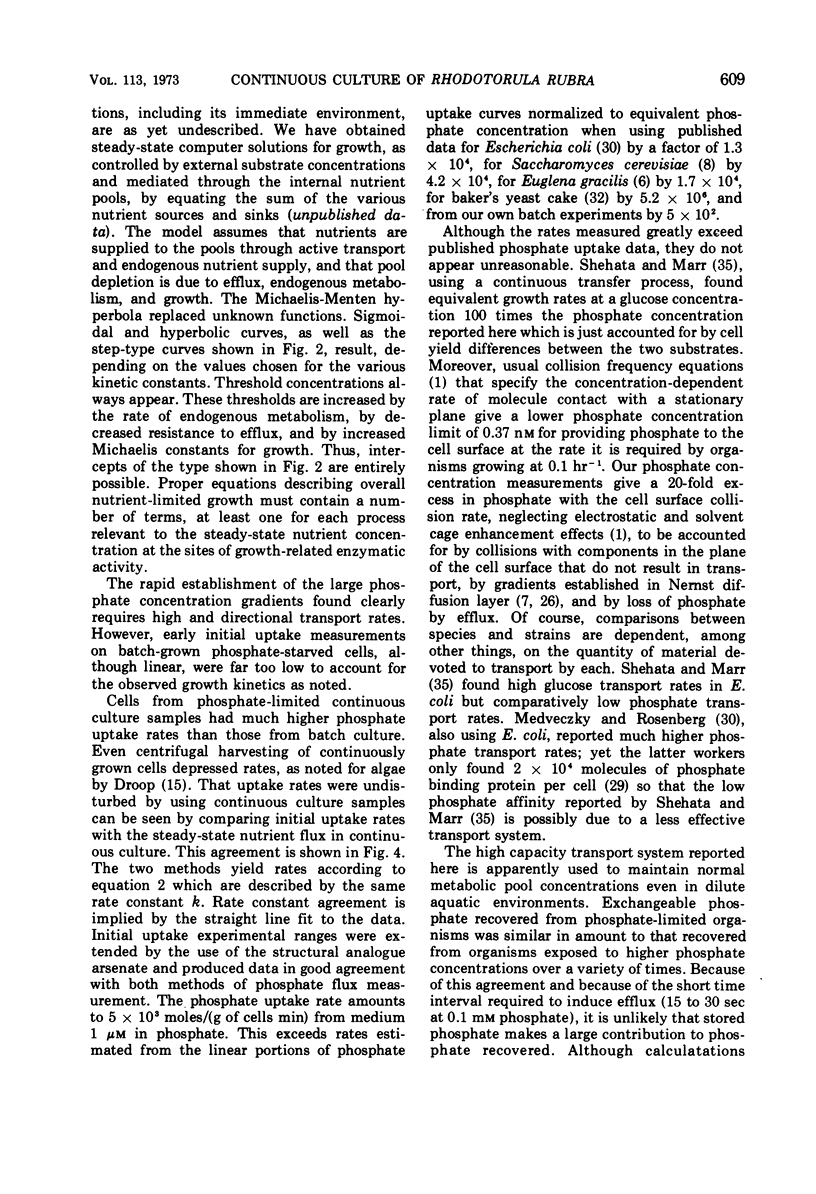
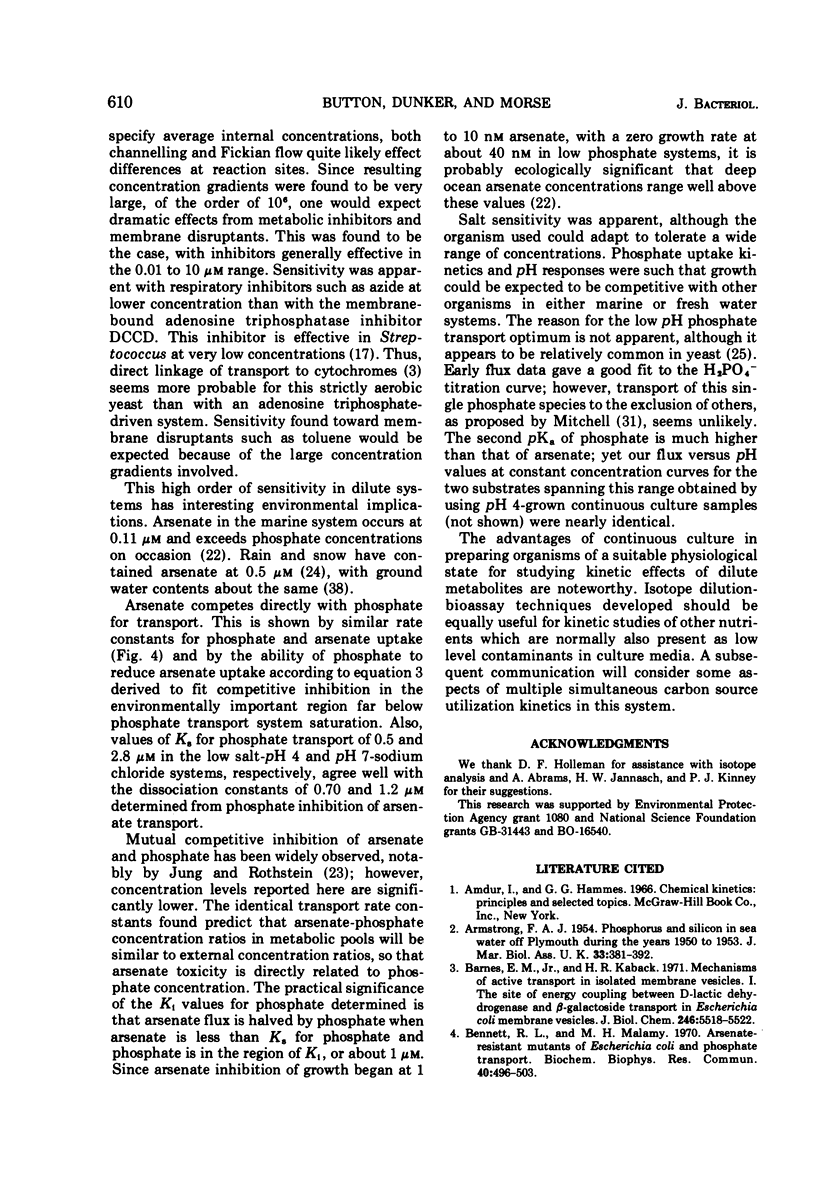
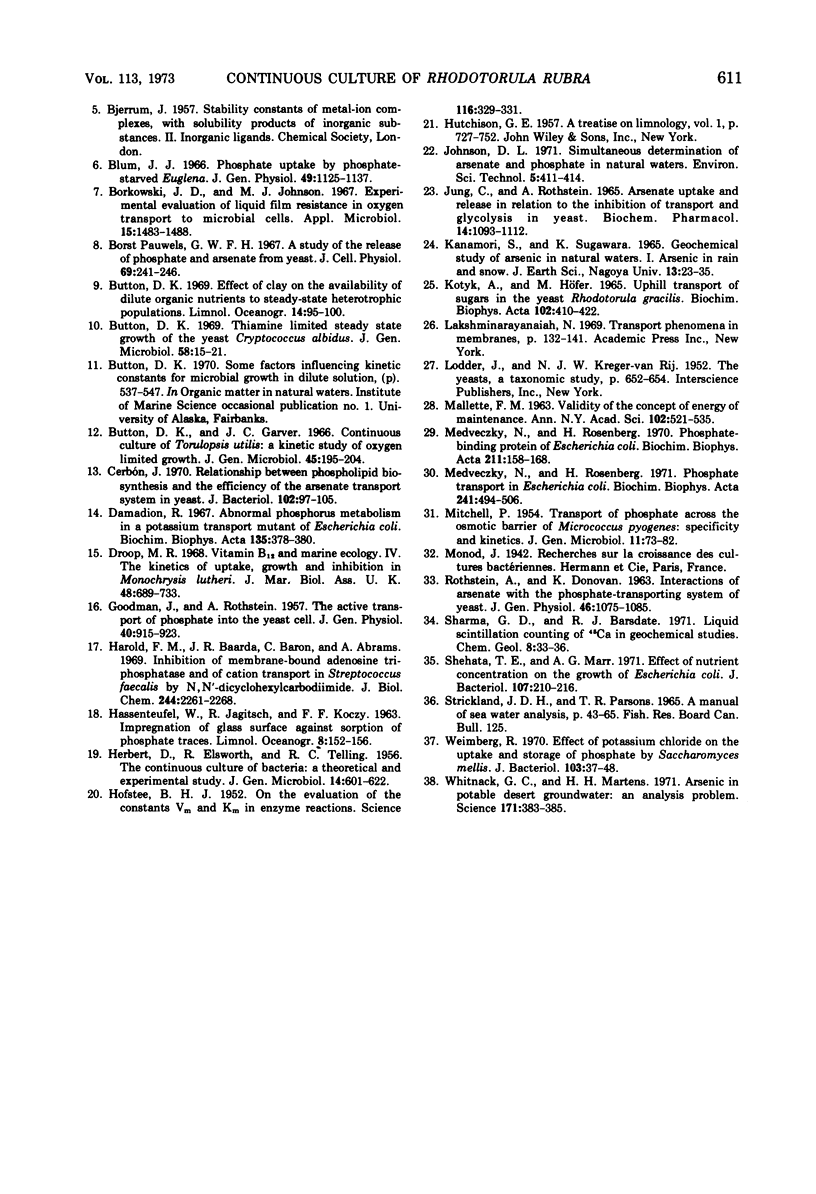
Selected References
These references are in PubMed. This may not be the complete list of references from this article.
- Barnes E. M., Jr, Kaback H. R. Mechanisms of active transport in isolated membrane vesicles. I. The site of energy coupling between D-lactic dehydrogenase and beta-galactoside transport in Escherichia coli membrane vesicles. J Biol Chem. 1971 Sep 10;246(17):5518–5522. [PubMed] [Google Scholar]
- Bennett R. L., Malamy M. H. Arsenate resistant mutants of Escherichia coli and phosphate transport. Biochem Biophys Res Commun. 1970 Jul 27;40(2):496–503. doi: 10.1016/0006-291x(70)91036-3. [DOI] [PubMed] [Google Scholar]
- Blum J. J. Phosphate uptake by phosphate-starved Euglena. J Gen Physiol. 1966 Jul;49(6):1125–1137. doi: 10.1085/jgp.0491125. [DOI] [PMC free article] [PubMed] [Google Scholar]
- Borkowski J. D., Johnson M. J. Experimental evaluation of liquid film resistance in oxygen transport to microbial cells. Appl Microbiol. 1967 Nov;15(6):1483–1488. doi: 10.1128/am.15.6.1483-1488.1967. [DOI] [PMC free article] [PubMed] [Google Scholar]
- Borst Pauwels G. W. A study of the release of phosphate and arsenate from yeast. J Cell Physiol. 1967 Apr;69(2):241–246. doi: 10.1002/jcp.1040690214. [DOI] [PubMed] [Google Scholar]
- Button D. K., Garver J. C. Continuous culture of Torulopsis utilis: a kinetic study of oxygen limited growth. J Gen Microbiol. 1966 Nov;45(2):195–204. doi: 10.1099/00221287-45-2-195. [DOI] [PubMed] [Google Scholar]
- Button D. K. Thiamine limited steady state growth of the yeast Cryptococcus albidus. J Gen Microbiol. 1969 Sep;58(1):15–21. doi: 10.1099/00221287-58-1-15. [DOI] [PubMed] [Google Scholar]
- Damadian R. Abnormal phosphorus metabolism in a potassium transport mutant of Escherichia coli. Biochim Biophys Acta. 1967 May 2;135(2):378–380. doi: 10.1016/0005-2736(67)90137-x. [DOI] [PubMed] [Google Scholar]
- GOODMAN J., ROTHSTEIN A. The active transport of phosphate into the yeast cell. J Gen Physiol. 1957 Jul 20;40(6):915–923. doi: 10.1085/jgp.40.6.915. [DOI] [PMC free article] [PubMed] [Google Scholar]
- HERBERT D., ELSWORTH R., TELLING R. C. The continuous culture of bacteria; a theoretical and experimental study. J Gen Microbiol. 1956 Jul;14(3):601–622. doi: 10.1099/00221287-14-3-601. [DOI] [PubMed] [Google Scholar]
- HOFSTEE B. H. J. On the evaluation of the constants Vm and KM in enzyme reactions. Science. 1952 Sep 26;116(3013):329–331. doi: 10.1126/science.116.3013.329. [DOI] [PubMed] [Google Scholar]
- Harold F. M., Baarda J. R., Baron C., Abrams A. Inhibition of membrane-bound adenosine triphosphatase and of cation transport in Streptococcus faecalis by N,N'-dicyclohexylcarbodiimide. J Biol Chem. 1969 May 10;244(9):2261–2268. [PubMed] [Google Scholar]
- Jung C., Rothstein A. Arsenate uptake and release in relation to the inhibition of transport and glycolysis in yeast. Biochem Pharmacol. 1965 Jul;14(7):1093–1112. doi: 10.1016/0006-2952(65)90039-0. [DOI] [PubMed] [Google Scholar]
- Kotyk A., Höfer M. Uphill transport of sugars in the yeast Rhodotorula gracilis. Biochim Biophys Acta. 1965 Jul 22;102(2):410–422. doi: 10.1016/0926-6585(65)90131-7. [DOI] [PubMed] [Google Scholar]
- MALLETTE M. F. Validity of the concept of energy of maintenance. Ann N Y Acad Sci. 1963 Jan 21;102:521–535. doi: 10.1111/j.1749-6632.1963.tb13658.x. [DOI] [PubMed] [Google Scholar]
- MITCHELL P. Transport of phosphate across the osmotic barrier of Micrococcus pyogenes; specificity and kinetics. J Gen Microbiol. 1954 Aug;11(1):73–82. doi: 10.1099/00221287-11-1-73. [DOI] [PubMed] [Google Scholar]
- Medveczky N., Rosenberg H. Phosphate transport in Escherichia coli. Biochim Biophys Acta. 1971 Aug 13;241(2):494–506. doi: 10.1016/0005-2736(71)90048-4. [DOI] [PubMed] [Google Scholar]
- ROTHSTEIN A. Interactions of arsenate with the phosphate-transporting system of yeast. J Gen Physiol. 1963 May;46:1075–1085. doi: 10.1085/jgp.46.5.1075. [DOI] [PMC free article] [PubMed] [Google Scholar]
- Shehata T. E., Marr A. G. Effect of nutrient concentration on the growth of Escherichia coli. J Bacteriol. 1971 Jul;107(1):210–216. doi: 10.1128/jb.107.1.210-216.1971. [DOI] [PMC free article] [PubMed] [Google Scholar]
- Weimberg R. Effect of potassium chloride on the uptake and storage of phosphate by Saccharomyces mellis. J Bacteriol. 1970 Jul;103(1):37–48. doi: 10.1128/jb.103.1.37-48.1970. [DOI] [PMC free article] [PubMed] [Google Scholar]
- Whitnack G. C., Martens H. H. Arsenic in potable desert groundwater: an analysis problem. Science. 1971 Jan 29;171(3969):383–385. doi: 10.1126/science.171.3969.383. [DOI] [PubMed] [Google Scholar]