Abstract
The mechanism of glycine action in growth inhibition was studied on eight different species of bacteria of various genera representing the four most common peptidoglycan types. To inhibit the growth of the different organisms to 80%, glycine concentrations from 0.05 to 1.33 M had to be applied. The inhibited cells showed morphological aberrations. It has been demonstrated that glycine is incorporated into the nucleotide-activated peptidoglycan precursors. The amount of incorporated glycine was equivalent to the decrease in the amount of alanine. With one exception glycine is also incorporated into the peptidoglycan. Studies on the primary structure of both the peptidoglycan precursors and the corresponding peptidoglycan have revealed that glycine can replace l-alanine in position 1 and d-alanine residues in positions 4 and 5 of the peptide subunit. Replacement of l-alanine in position 1 of the peptide subunit together with an accumulation of uridine diphosphate-muramic acid (UDP-MurNAc), indicating an inhibition of the UDP-MurNAc:l-Ala ligase, has been found in three bacteria (Staphylococcus aureus, Lactobacillus cellobiosus and L. plantarum). However, discrimination against precursors with glycine in position 1 in peptidoglycan synthesis has been observed only in S. aureus. Replacement of d-alanine residues was most common. It occurred in the peptidoglycan with one exception in all strains studied. In Corynebacterium sp., C. callunae, L. plantarum, and L. cellobiosus most of the d-alanine replacing glycine occurs C-terminal in position 4, and in C. insidiosum and S. aureus glycine is found C-terminal in position 5. It is suggested that the modified peptidoglycan precursors are accumulated by being poor substrates for some of the enzymes involved in peptidoglycan synthesis. Two mechanisms leading to a more loosely cross-linked peptidoglycan and to morphological changes of the cells are considered. First, the accumulation of glycine-containing precursors may lead to a disrupture of the normal balance between peptidoglycan synthesis and controlled enzymatic hydrolysis during growth. Second, the modified glycine-containing precursors may be incorporated. Since these are poor substrates in the transpeptidation reaction, a high percentage of muropeptides remains uncross-linked. The second mechanism may be the more significant in most cases.
Full text
PDF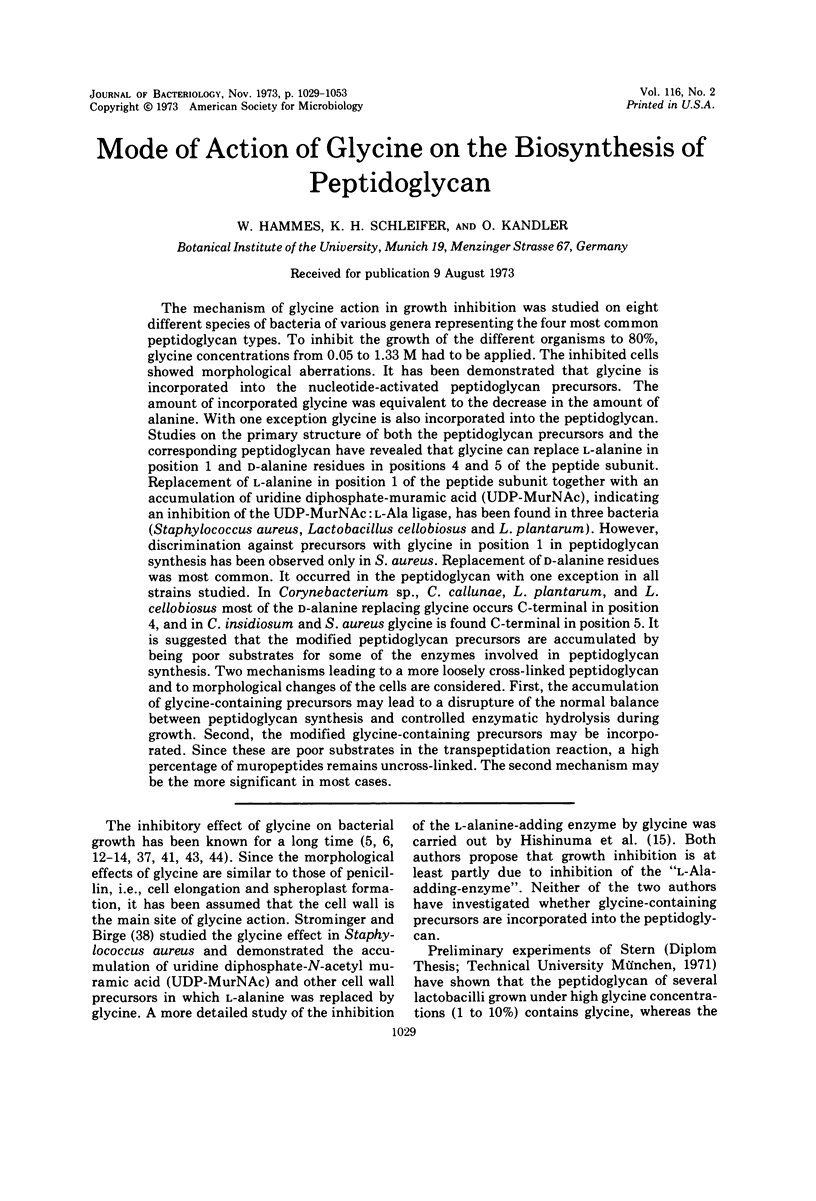
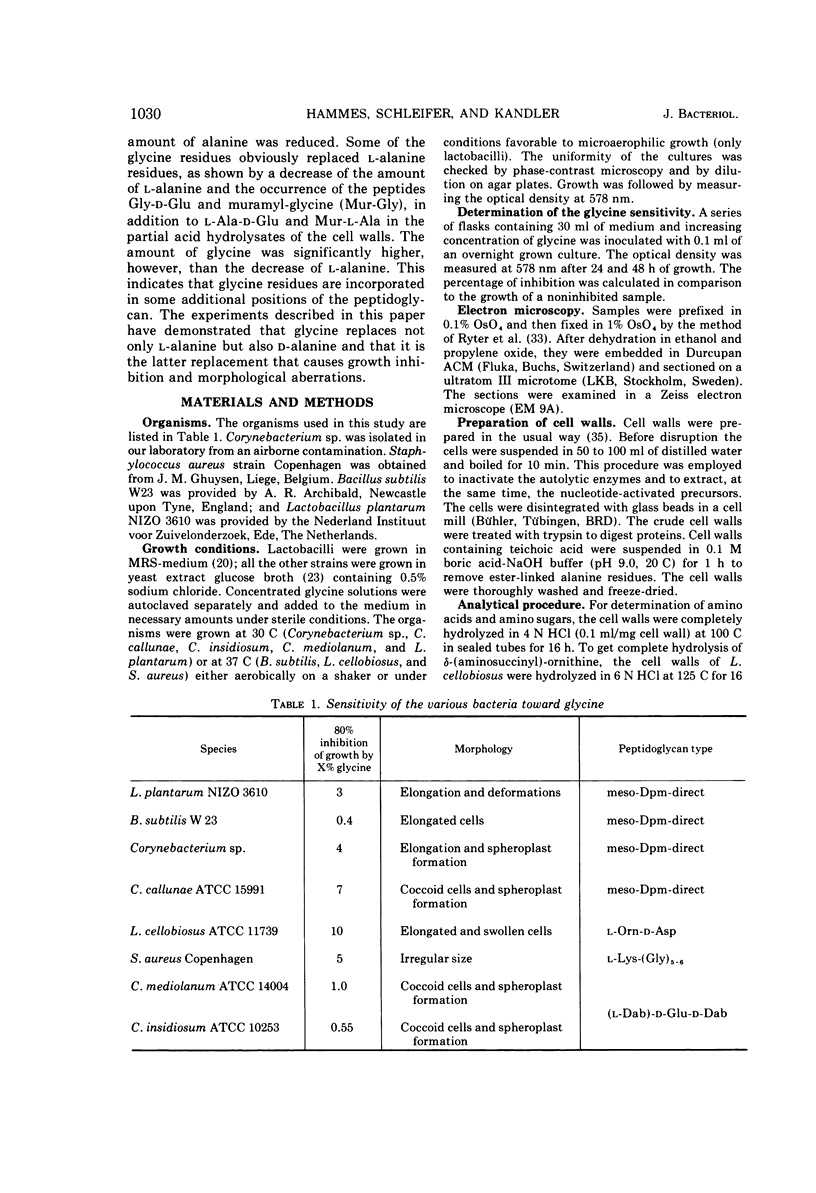
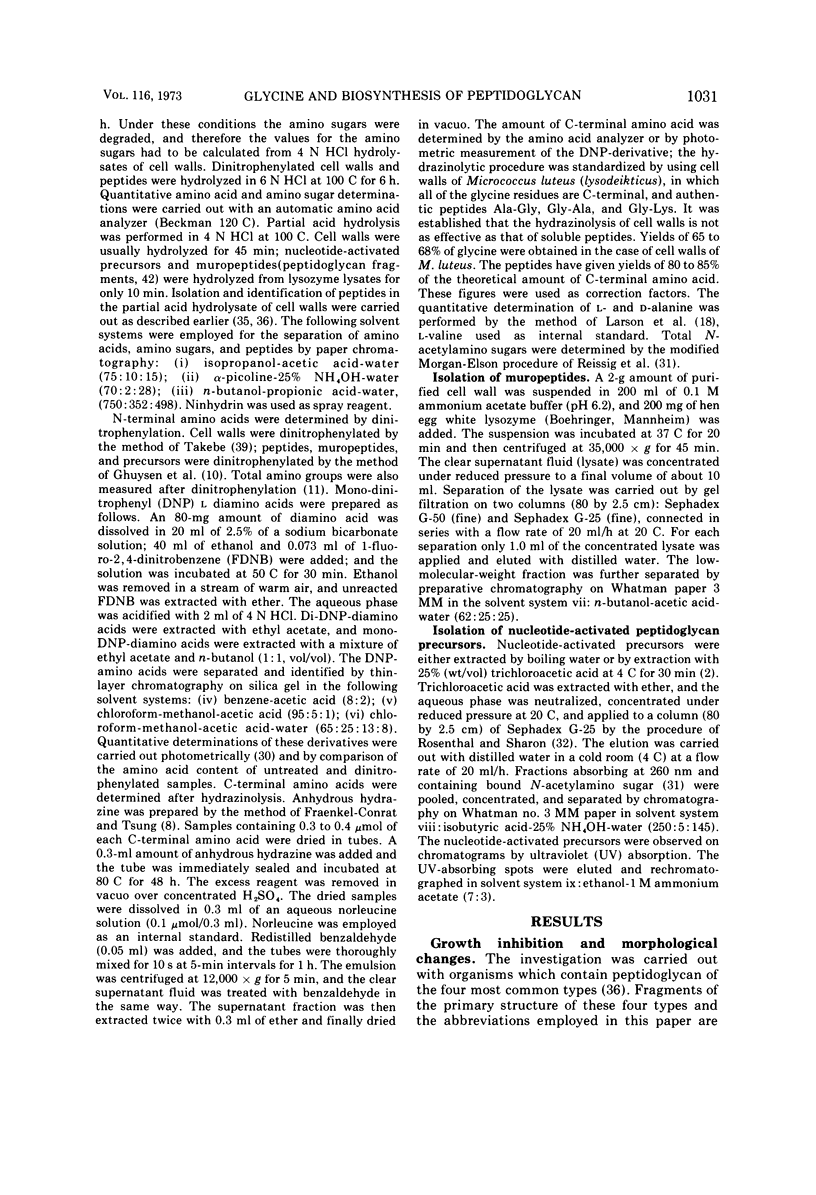
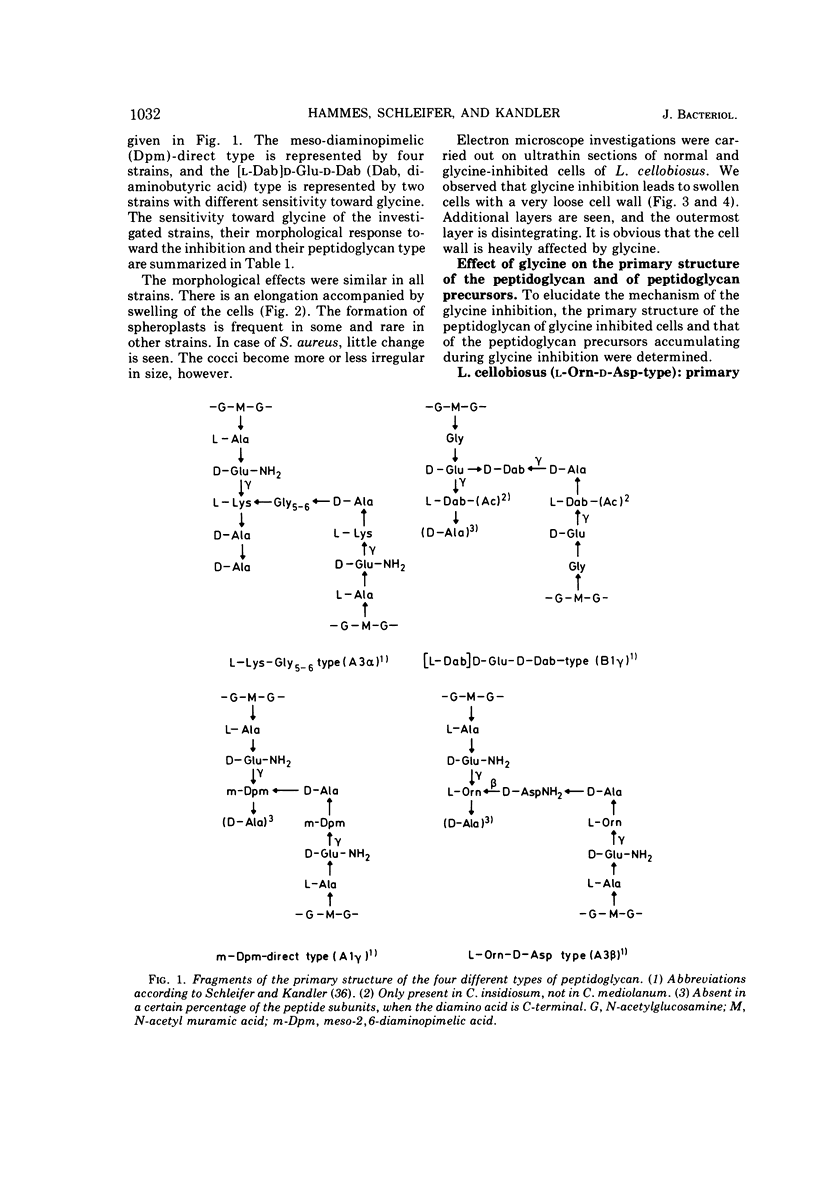
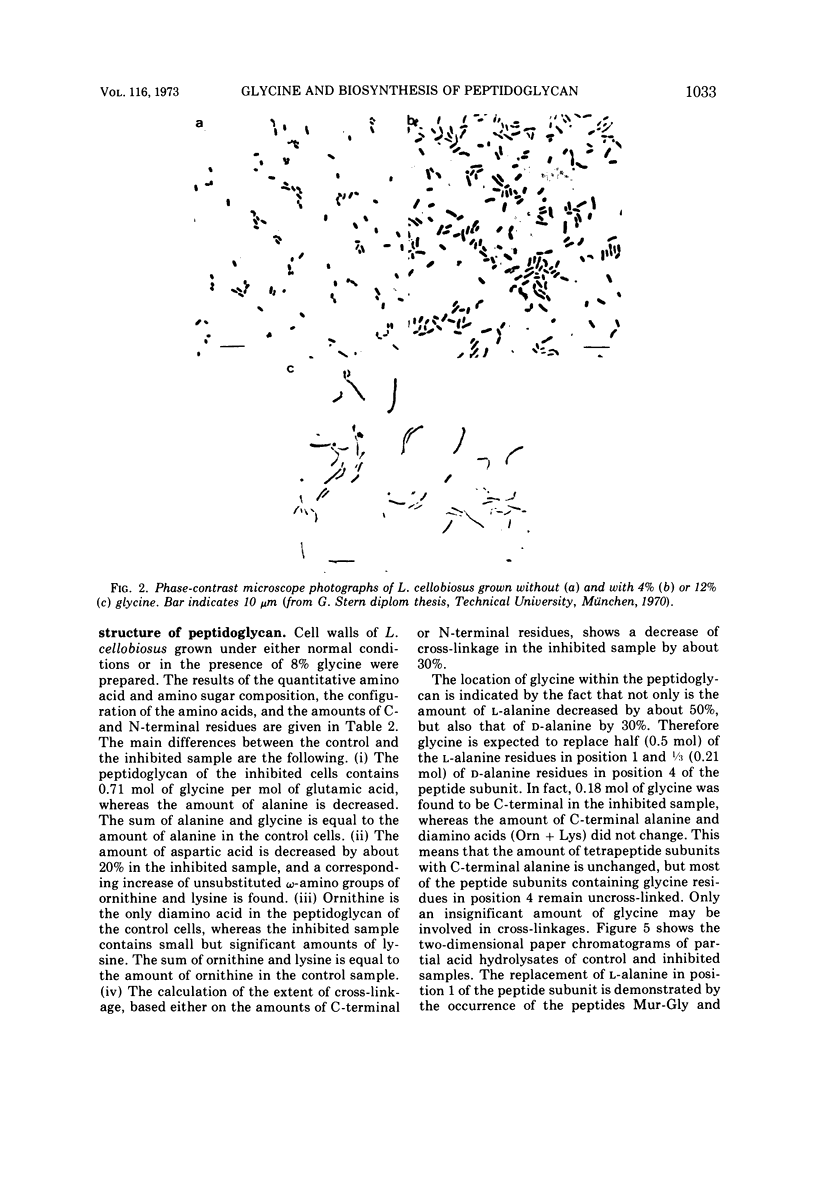
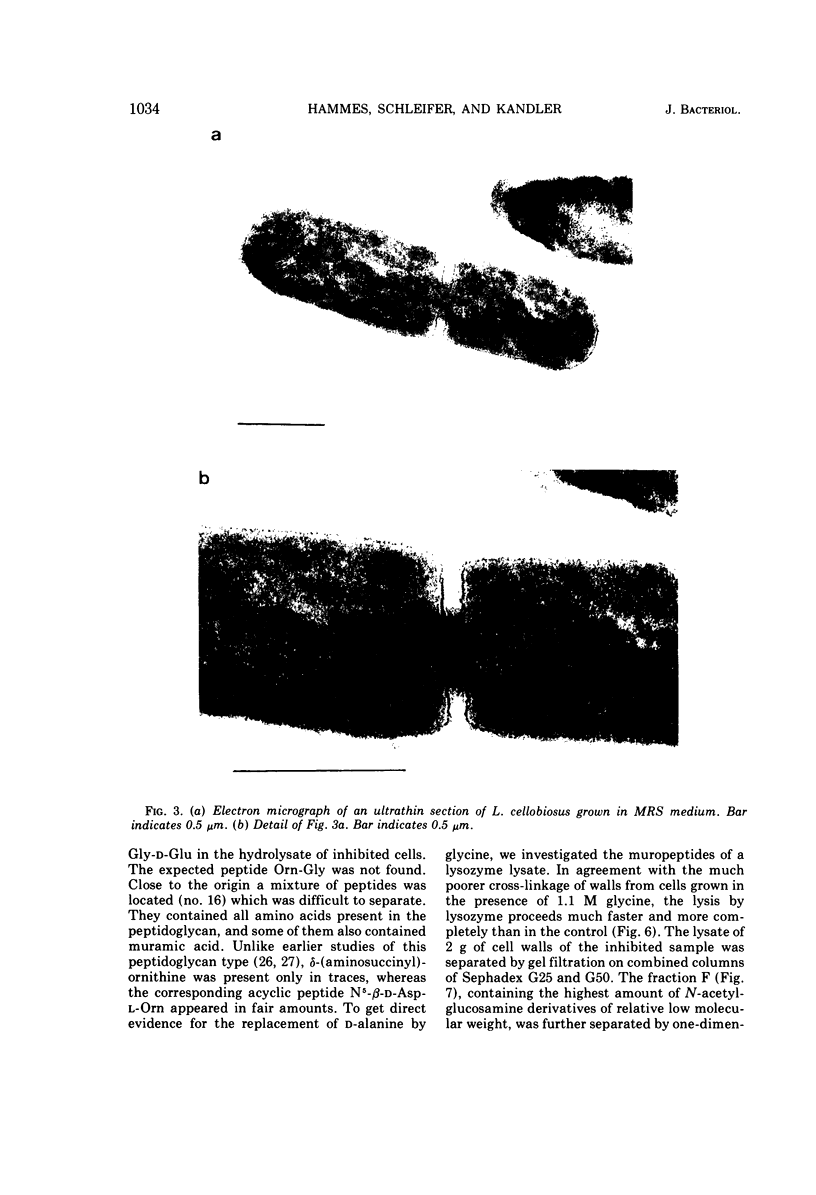
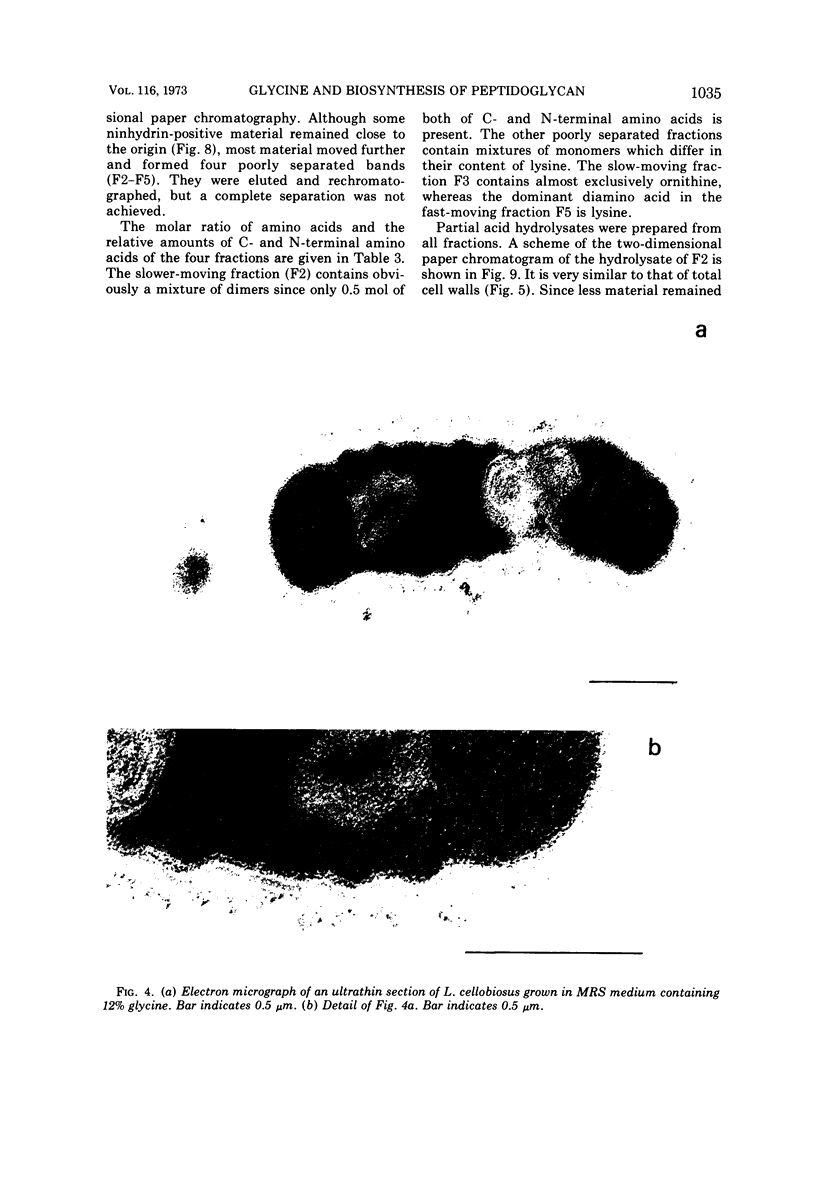
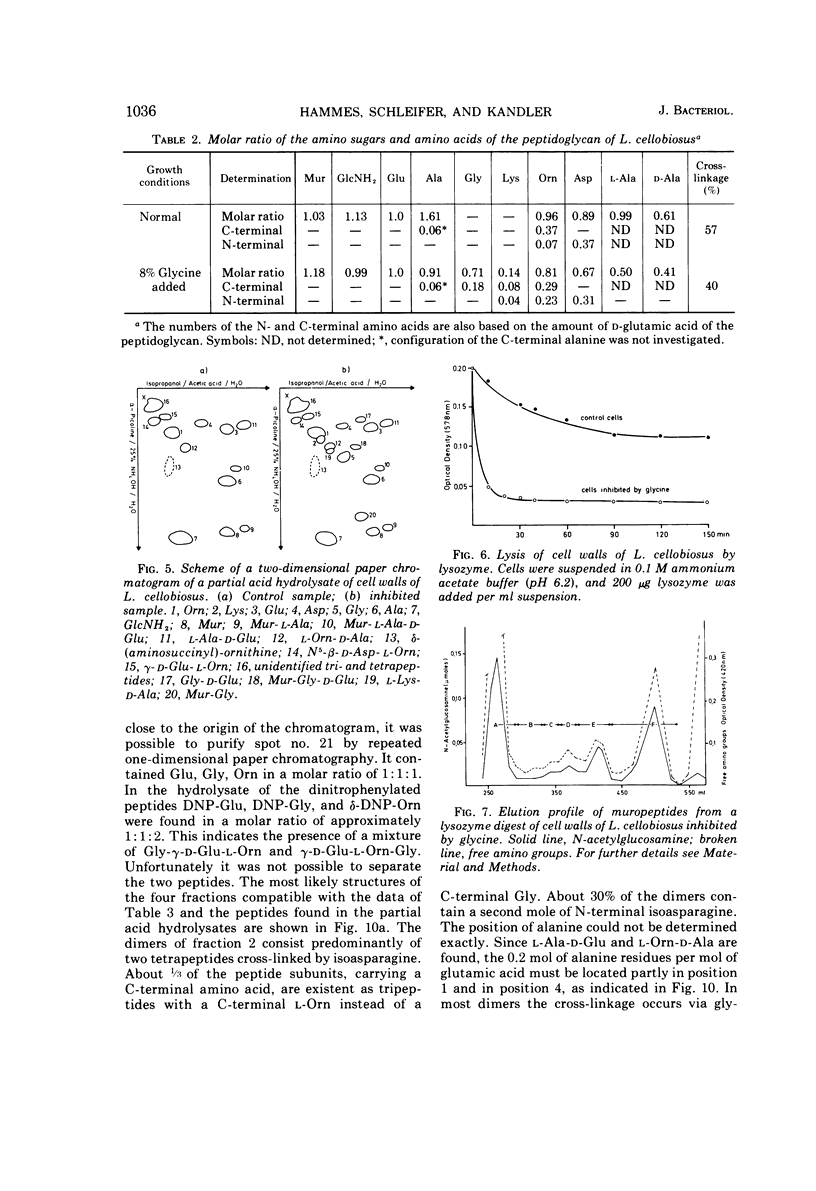
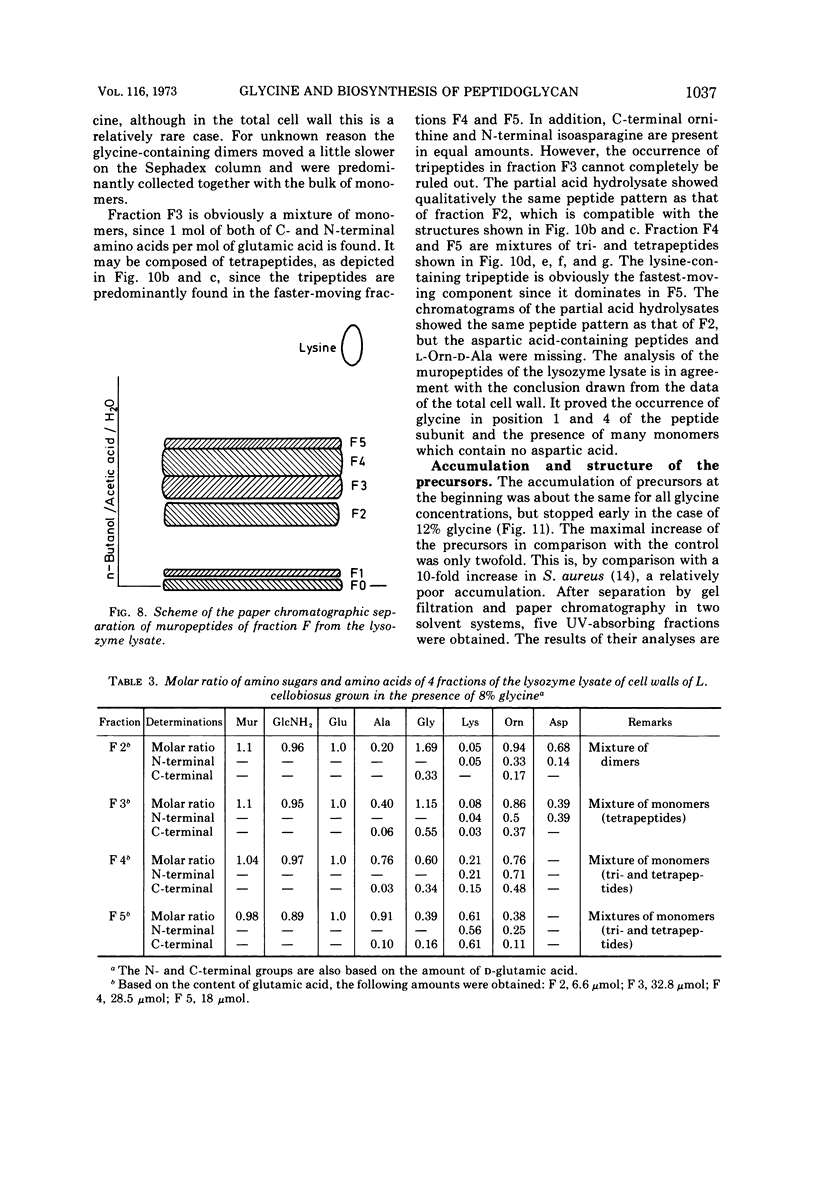
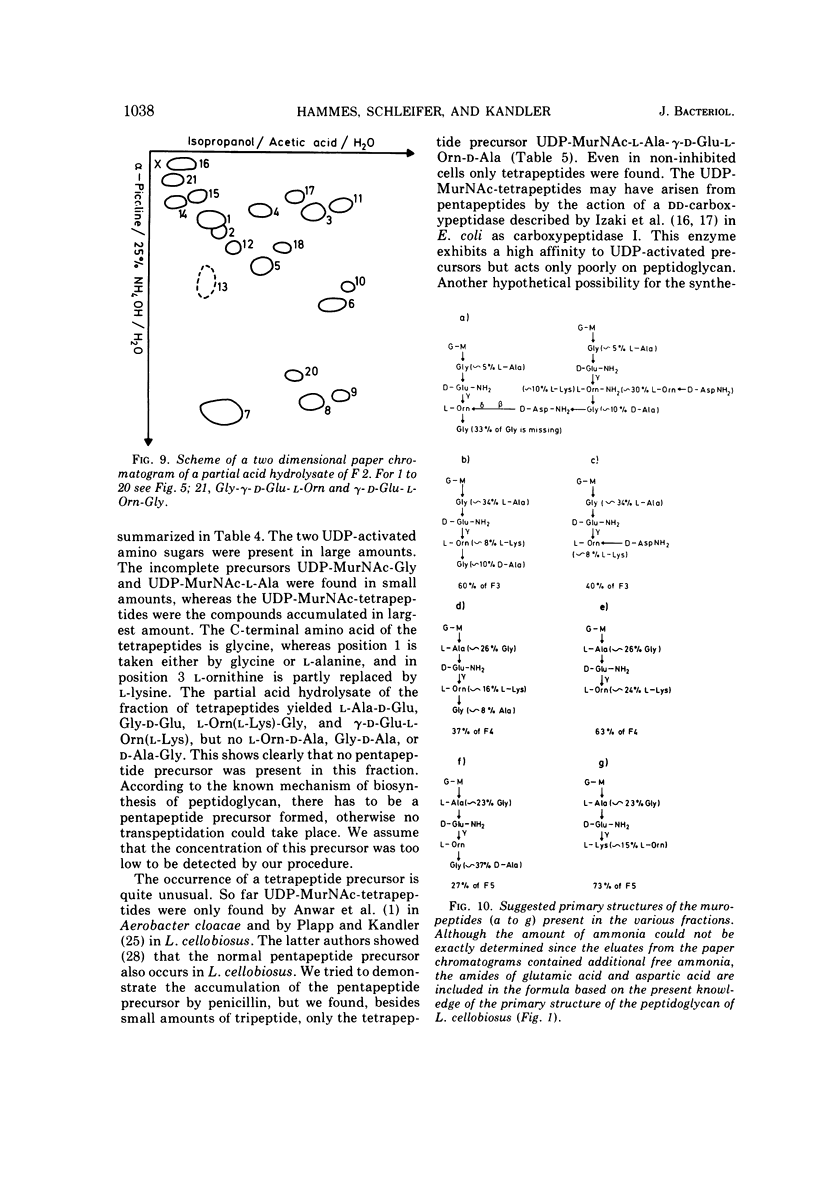
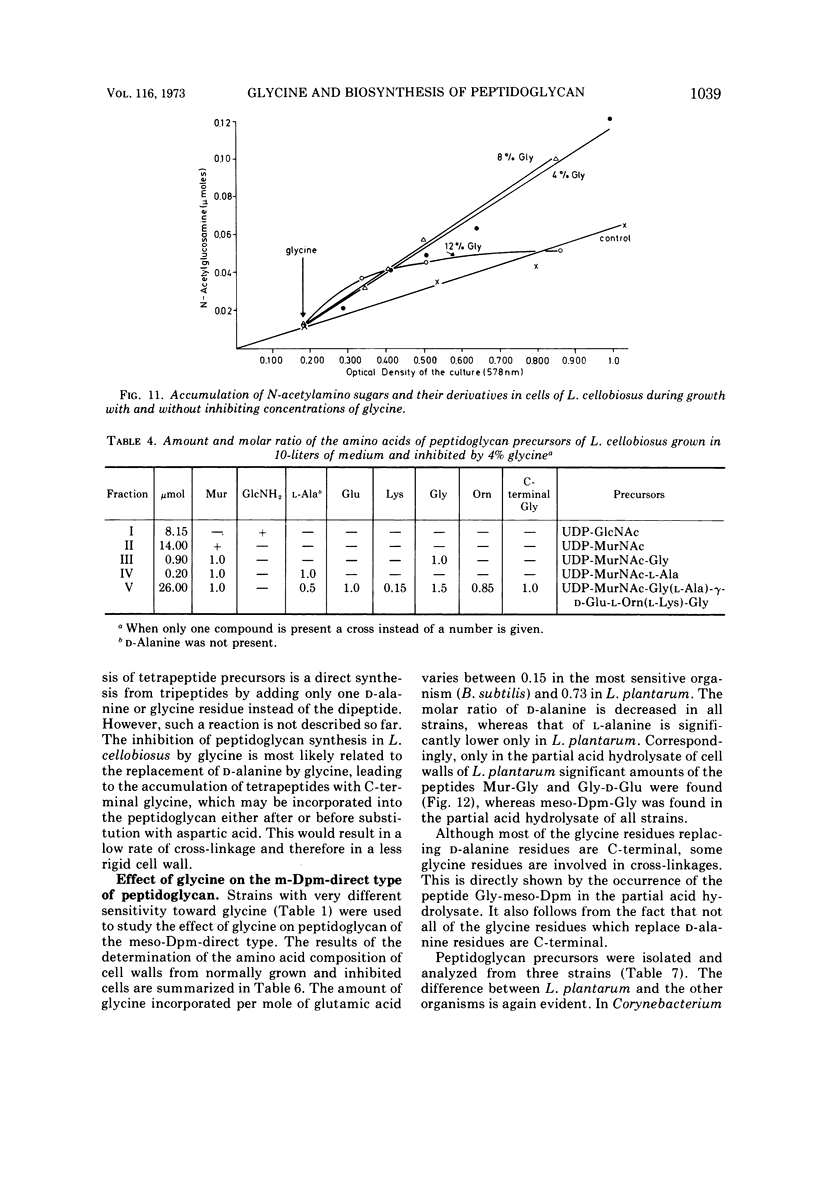
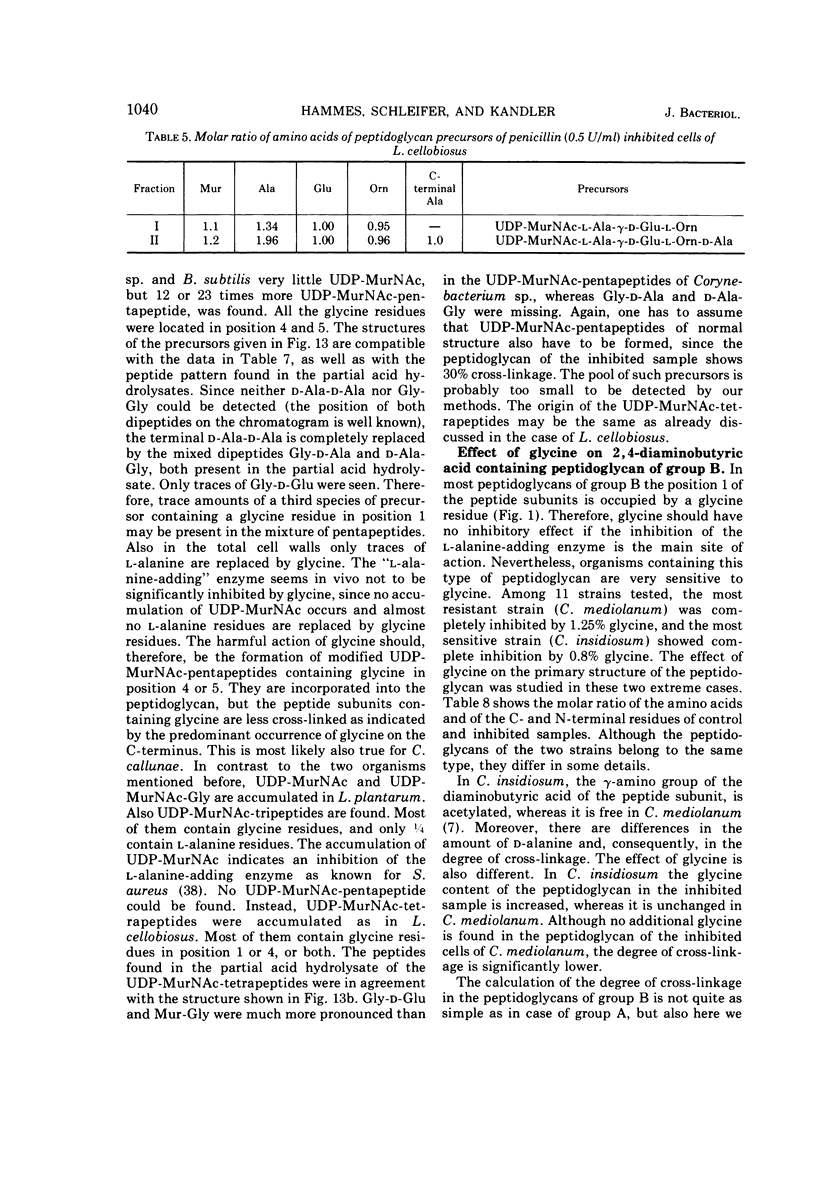
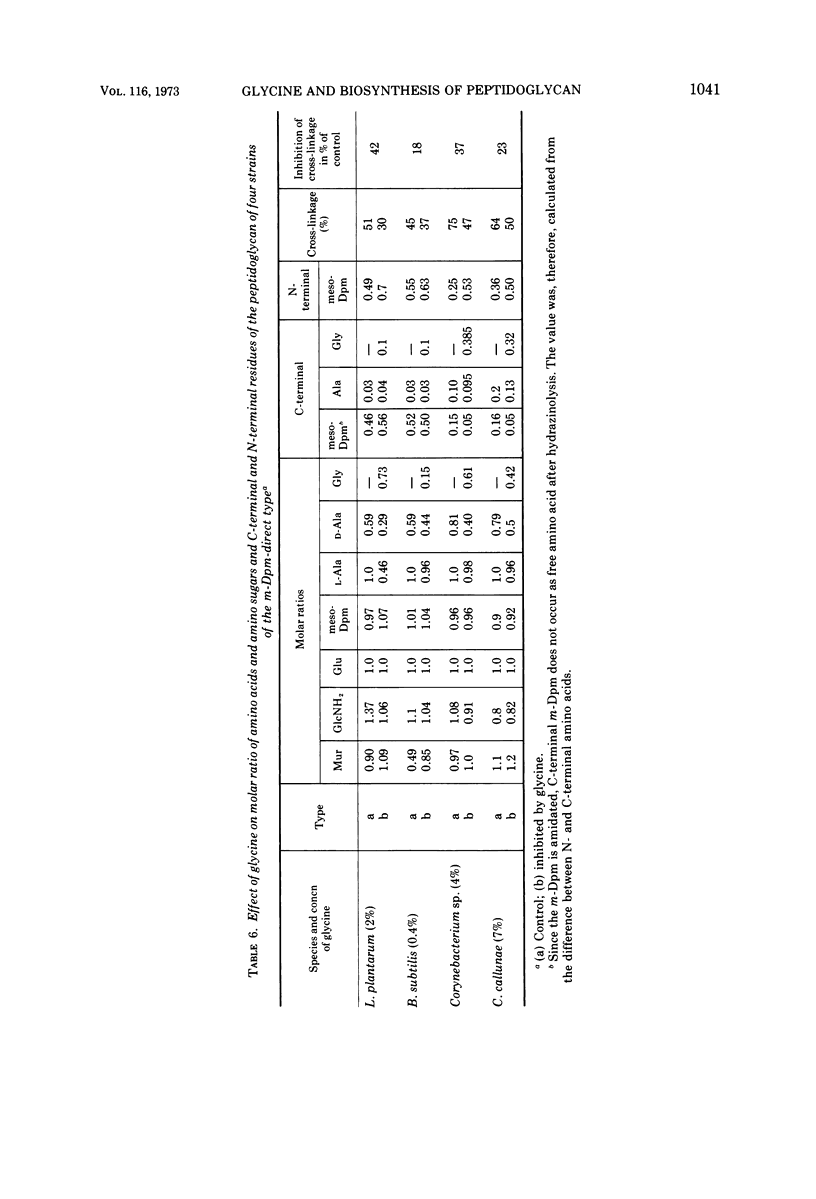
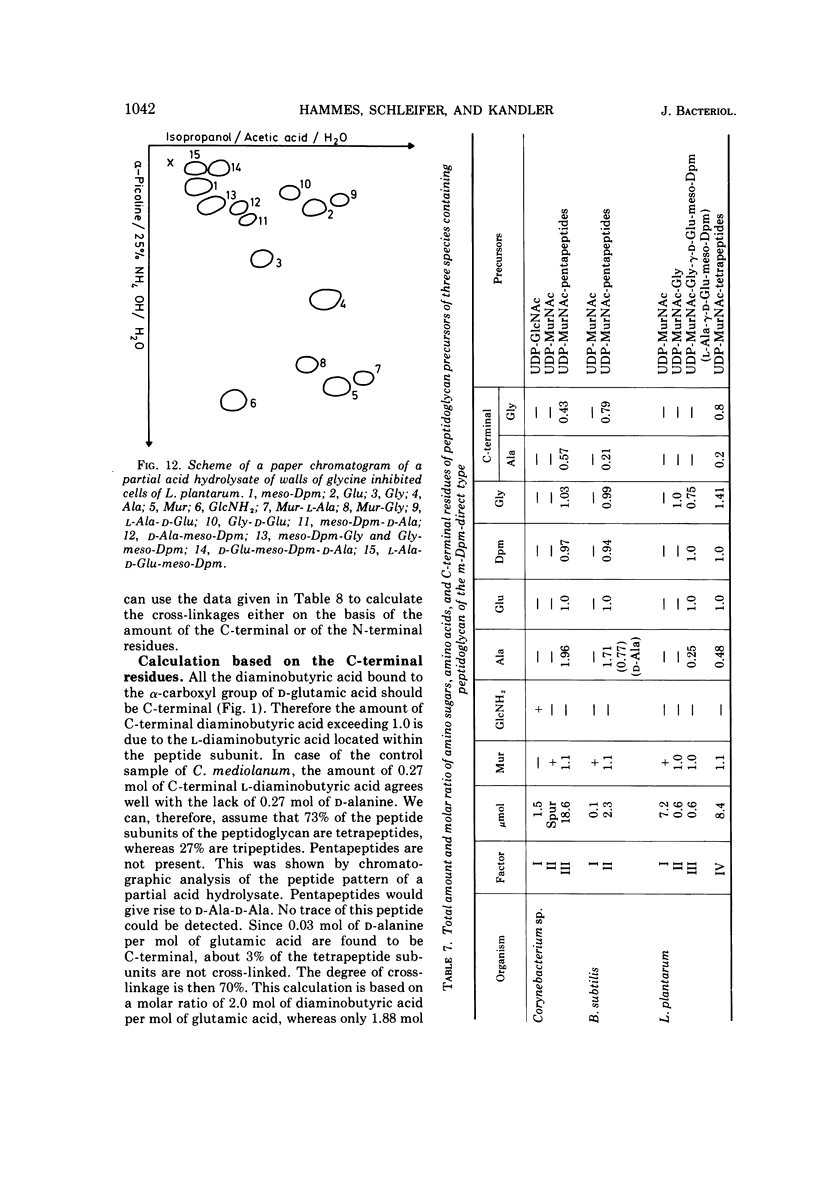
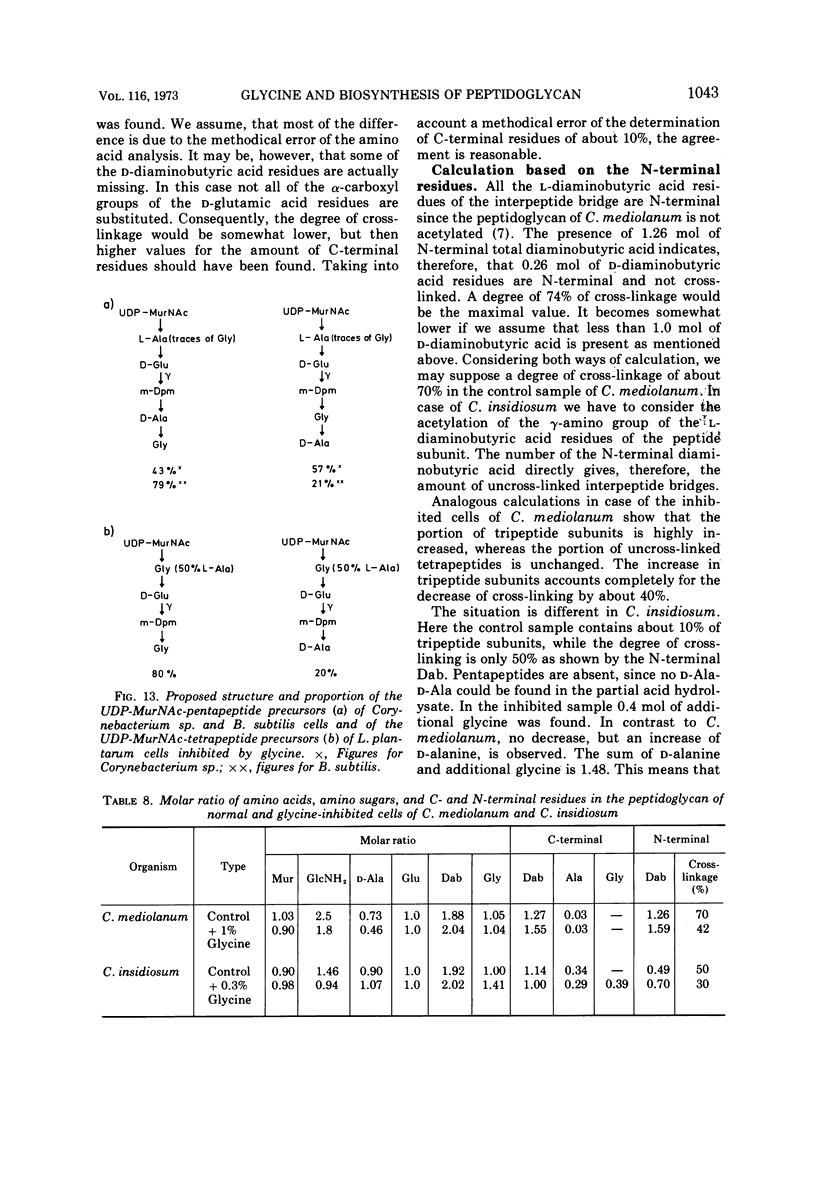
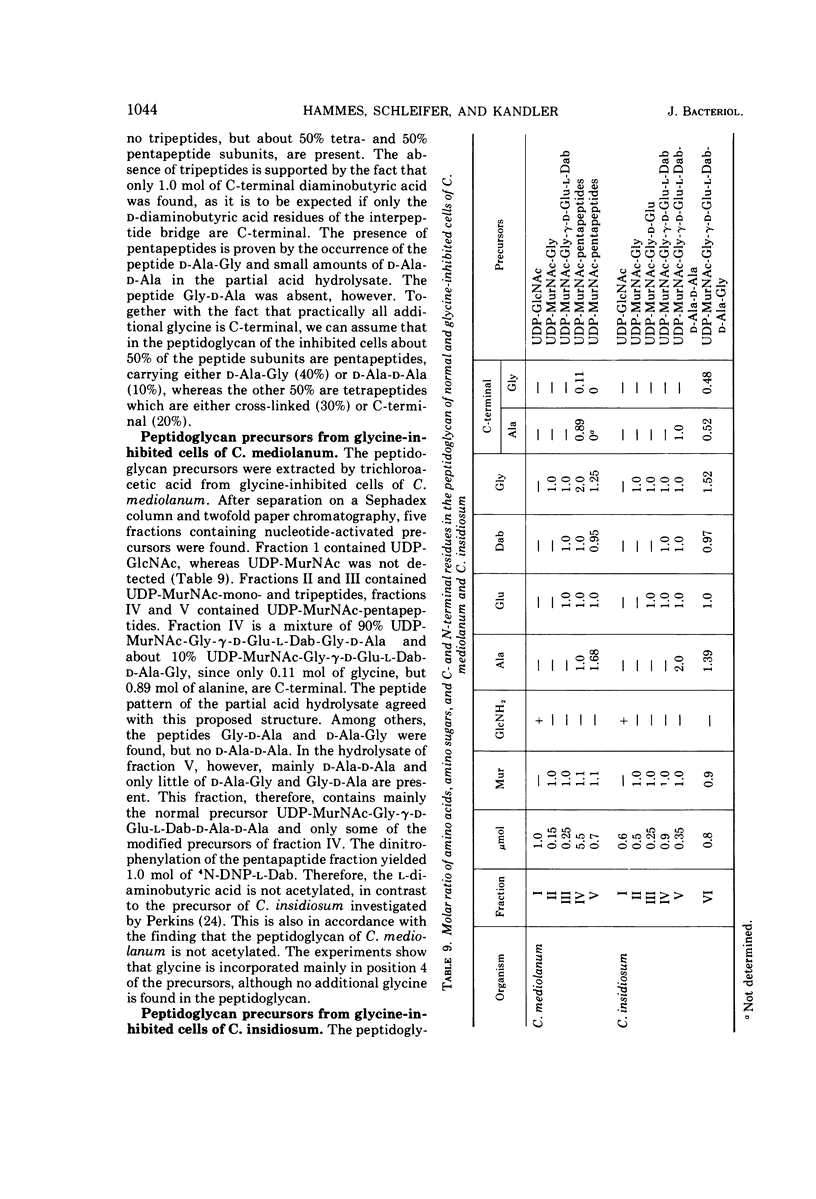
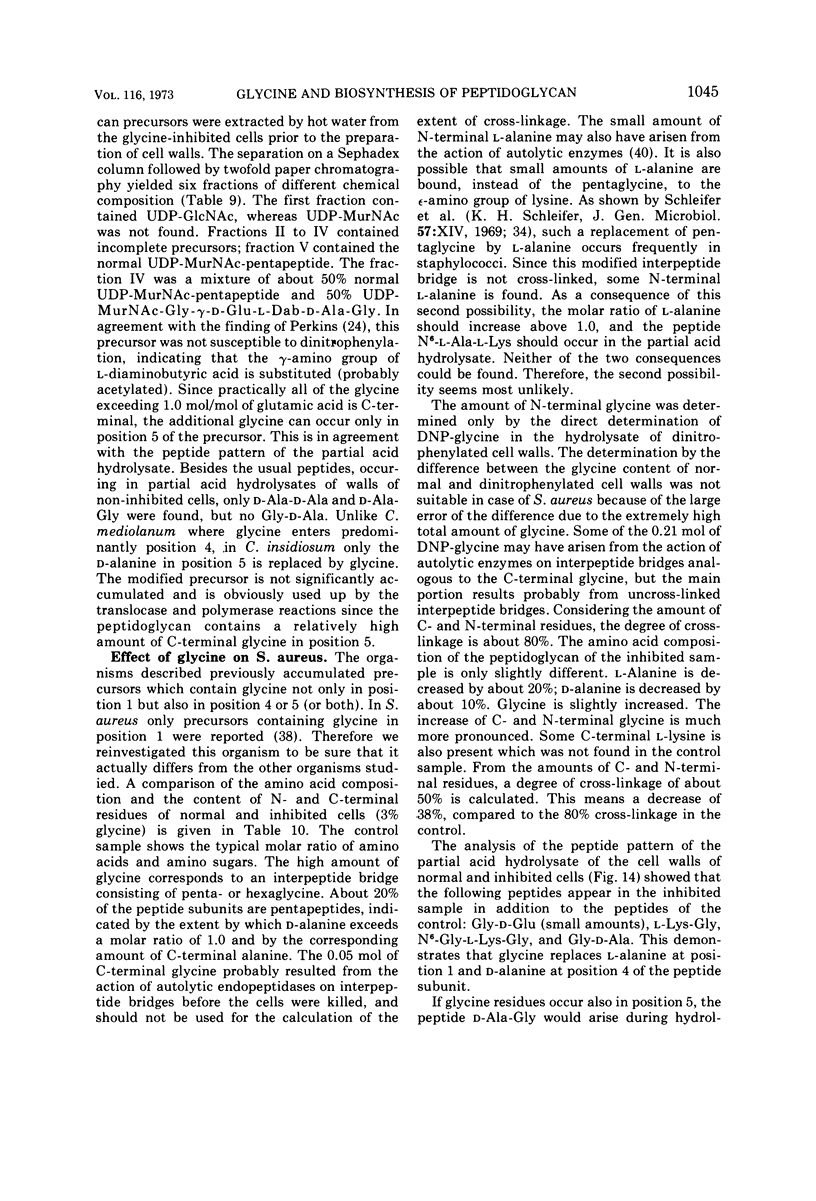
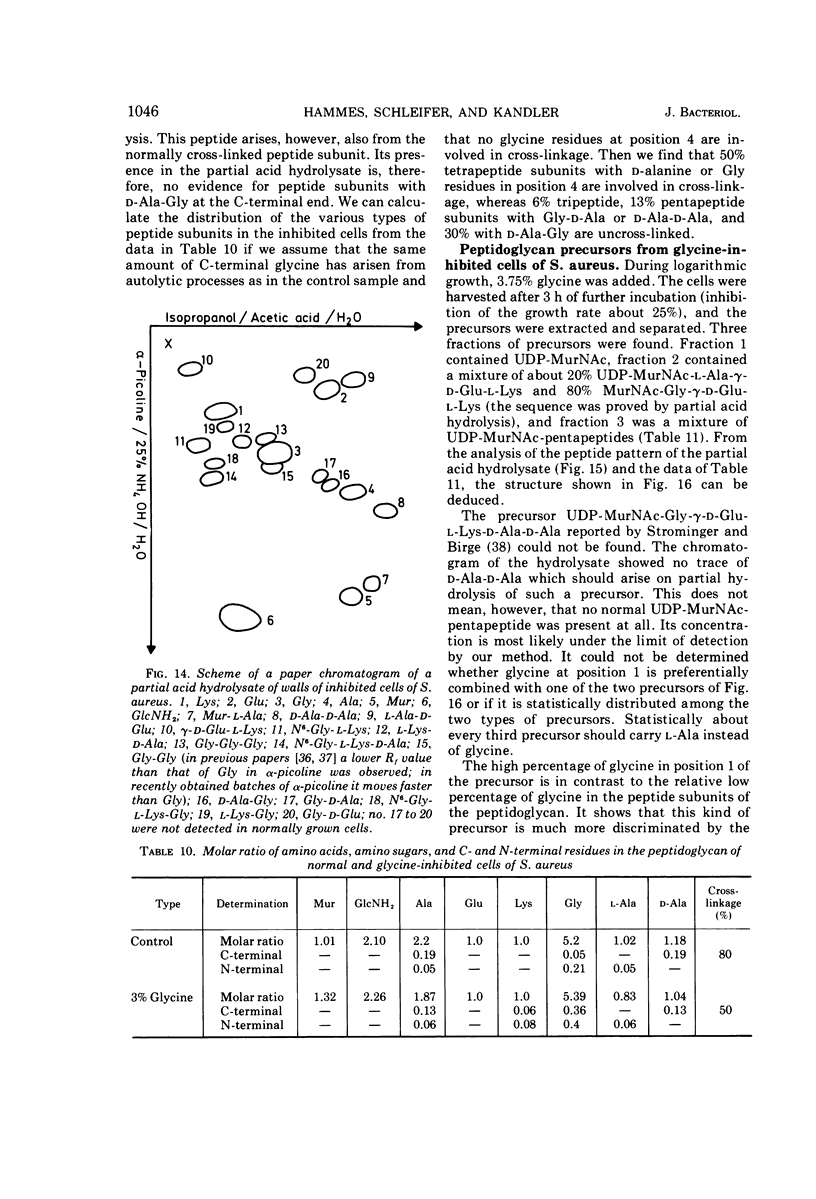
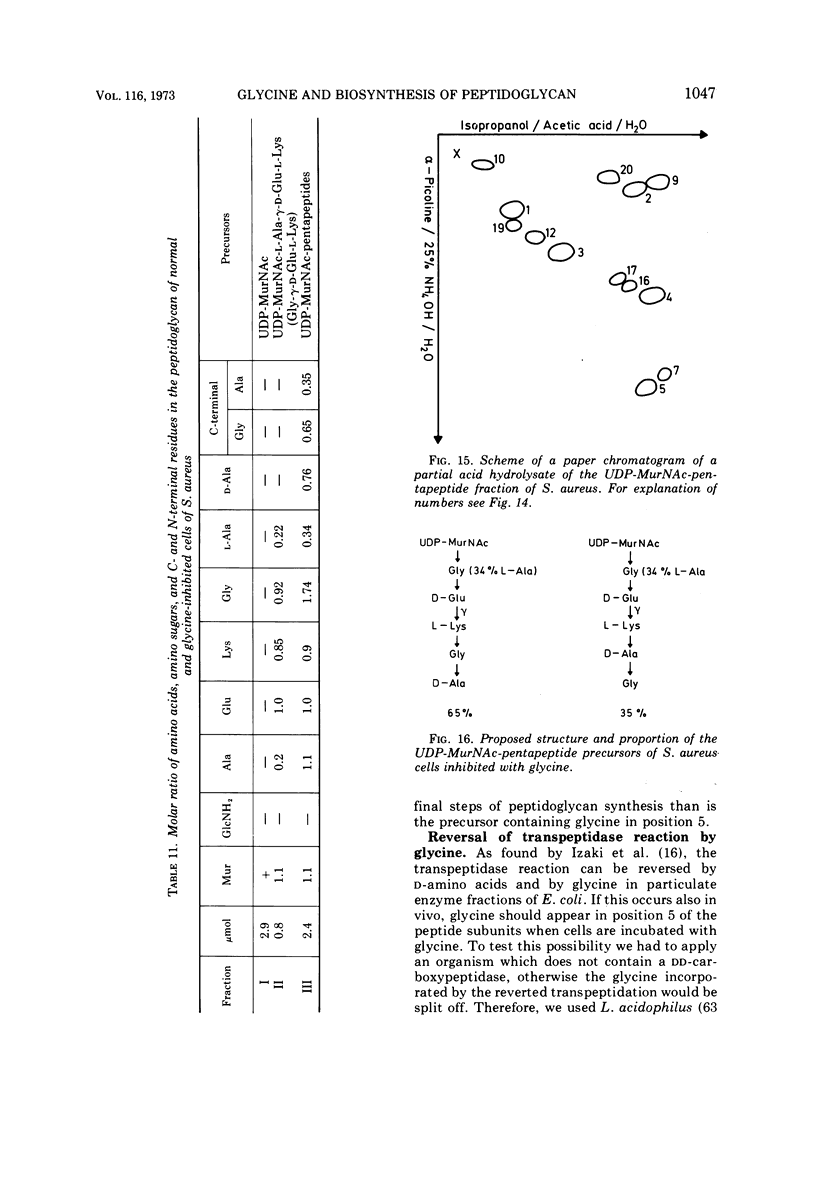
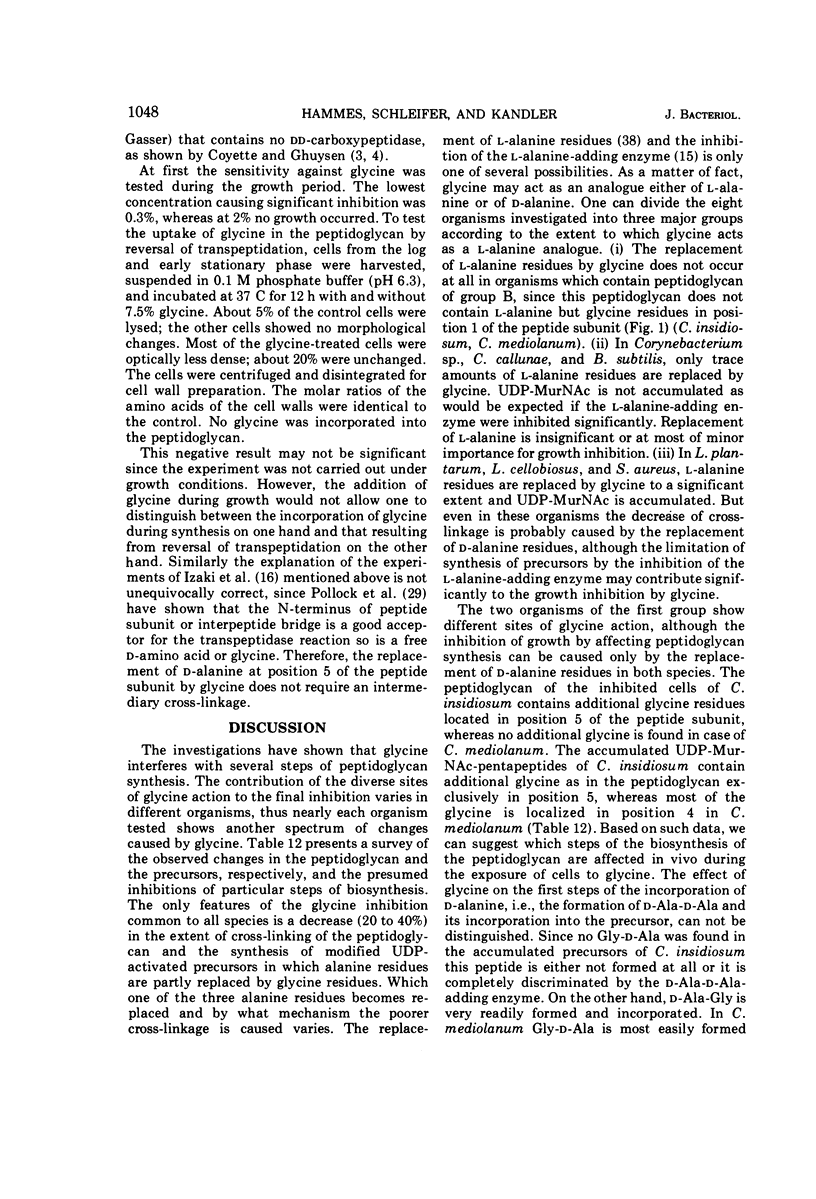
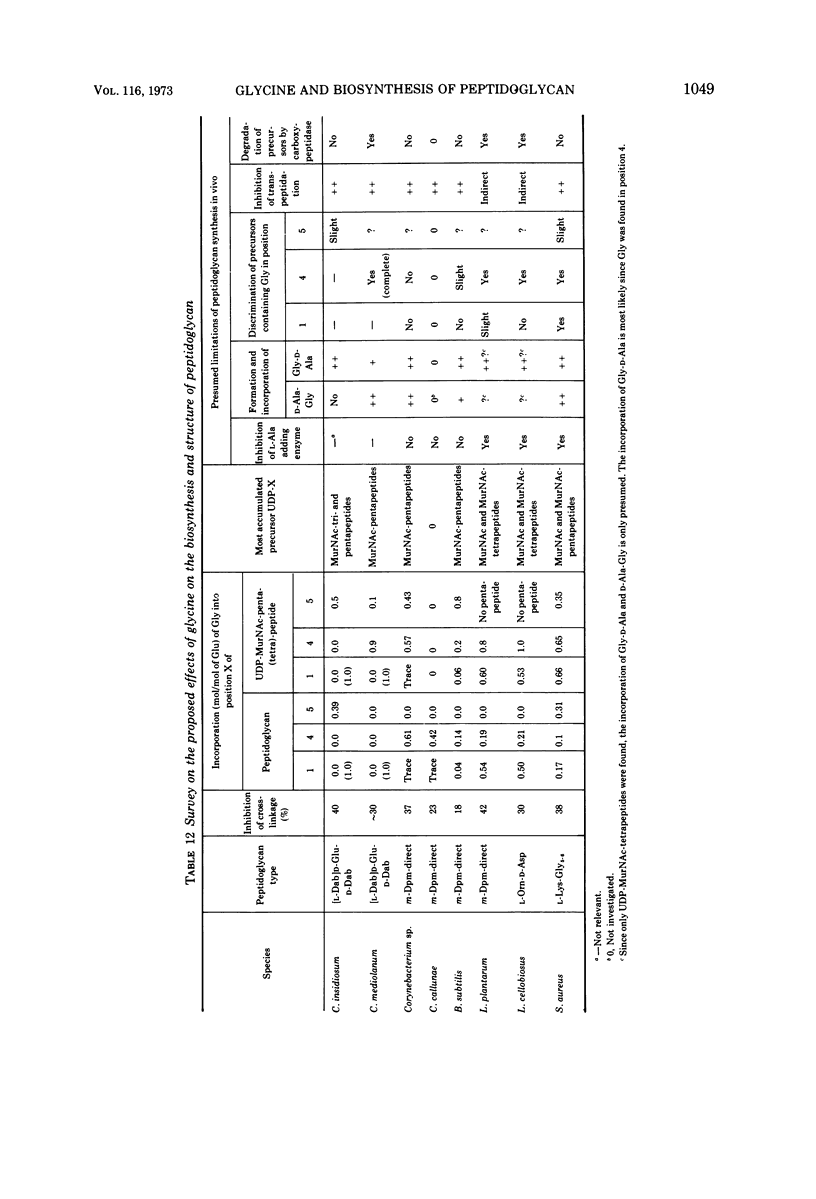
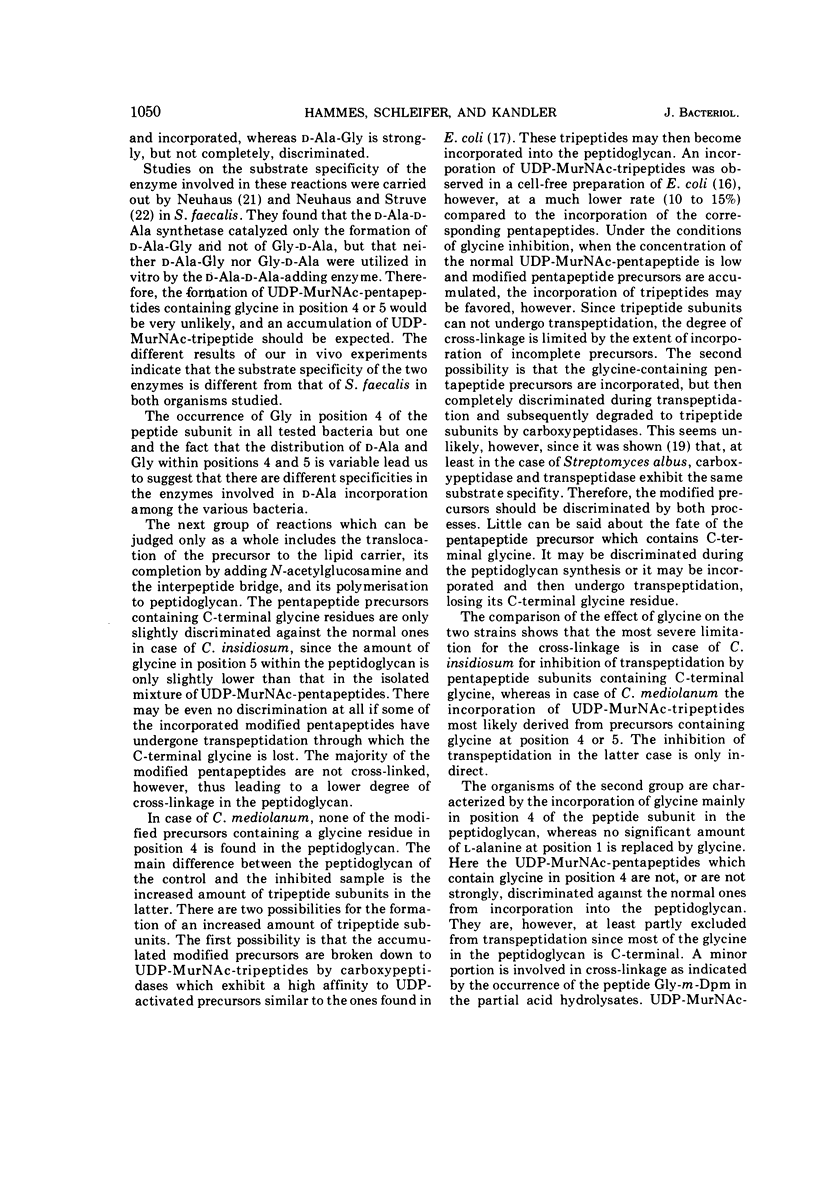
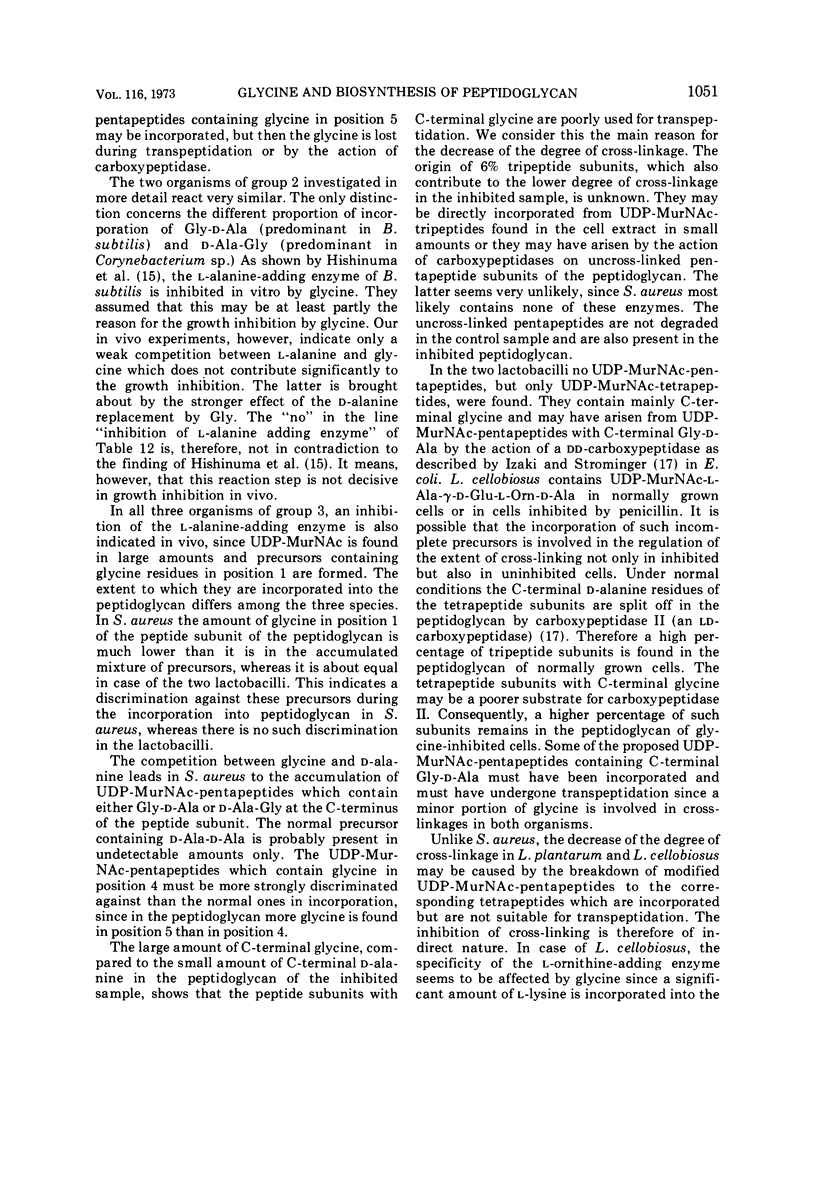
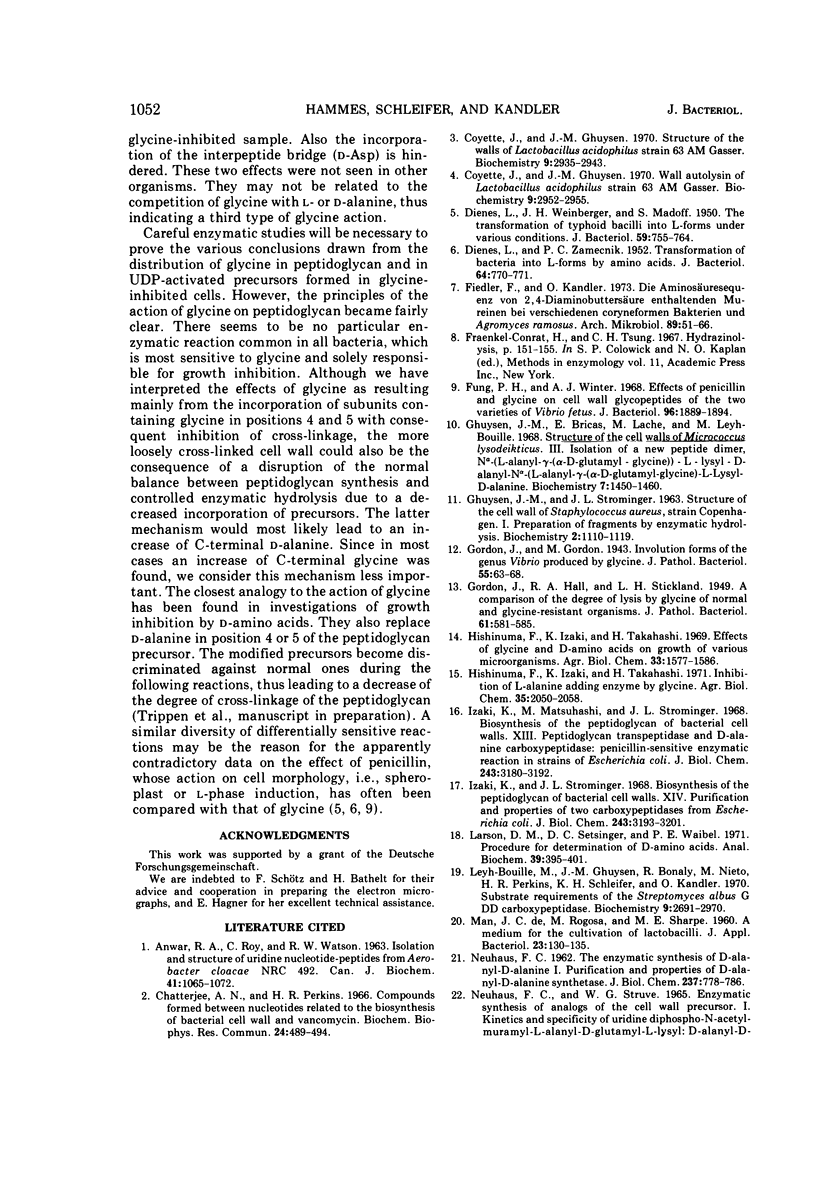
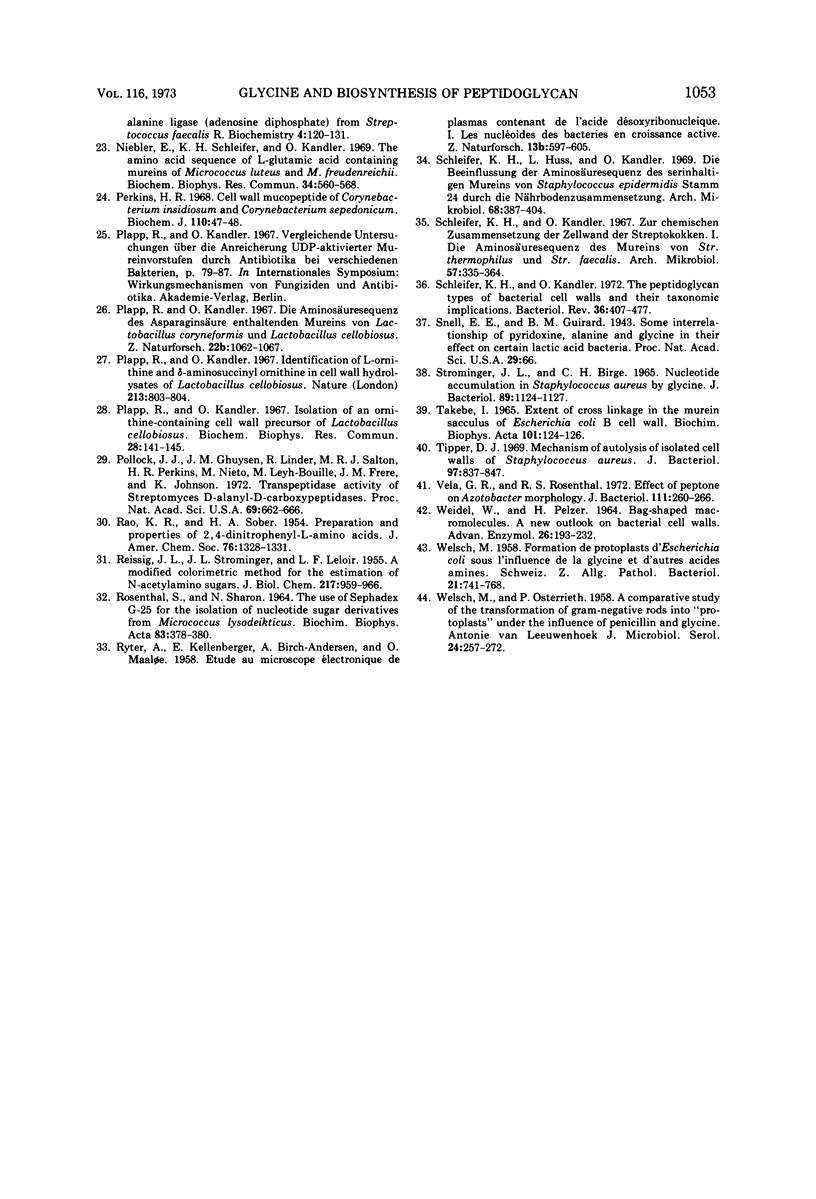
Images in this article
Selected References
These references are in PubMed. This may not be the complete list of references from this article.
- ANWAR R. A., ROY C., WATSON R. W. Isolation and structure of uridine nucleotide-peptides from Aerobacter cloacae NRC 492. Can J Biochem Physiol. 1963 Apr;41:1065–1072. [PubMed] [Google Scholar]
- Chatterjee A. N., Perkins H. R. Compounds formed between nucleotides related to the biosynthesis of bacterial cell wall and vancomycin. Biochem Biophys Res Commun. 1966 Aug 12;24(3):489–494. doi: 10.1016/0006-291x(66)90188-4. [DOI] [PubMed] [Google Scholar]
- Coyette J., Ghuysen J. M. Structure of the walls of Lactobacillus acidophilus strain 63 AM Gasser. Biochemistry. 1970 Jul 21;9(15):2935–2943. doi: 10.1021/bi00817a001. [DOI] [PubMed] [Google Scholar]
- Coyette J., Ghuysen J. M. Wall autolysin of Lactobacillus acidophilus strain 63 AM gasser. Biochemistry. 1970 Jul 21;9(15):2952–2955. doi: 10.1021/bi00817a003. [DOI] [PubMed] [Google Scholar]
- DIENES L., WEINBERGER H. J., MADOFF S. The transformation of typhoid bacilli into L forms under various conditions. J Bacteriol. 1950 Jun;59(6):755–764. doi: 10.1128/jb.59.6.755-764.1950. [DOI] [PMC free article] [PubMed] [Google Scholar]
- DIENES L., ZAMECNIK P. C. Transformation of bacteria into L forms by amino acids. J Bacteriol. 1952 Nov;64(5):770–771. doi: 10.1128/jb.64.5.770-771.1952. [DOI] [PMC free article] [PubMed] [Google Scholar]
- Fiedler F., Kandler O. Die Aminosäuresequenz von 2,4-diaminobuttersäure enthaltenden Mureinen bei verschiedenen coryneformen Bakterien und Agromyces ramosus. Arch Mikrobiol. 1973;89(1):51–66. [PubMed] [Google Scholar]
- Fung P. H., Winter A. J. Effects of penicillin and glycine on cell wall glycopeptides of the two varieties of Vibrio fetus. J Bacteriol. 1968 Dec;96(6):1889–1894. doi: 10.1128/jb.96.6.1889-1894.1968. [DOI] [PMC free article] [PubMed] [Google Scholar]
- GHUYSEN J. M., STROMINGER J. L. STRUCTURE OF THE CELL WALL OF STAPHYLOCOCCUS AUREUS, STRAIN COPENHAGEN. I. PREPARATION OF FRAGMENTS BY ENZYMATIC HYDROLYSIS. Biochemistry. 1963 Sep-Oct;2:1110–1119. doi: 10.1021/bi00905a035. [DOI] [PubMed] [Google Scholar]
- Ghuysen J. M., Bricas E., Lache M., Leyh-Bouille M. Structure of the cell walls of Micrococcus lysodeikticus. 3. Isolation of a new peptide dimer, N-alpha-[L-alanyl-gamma-(alpha-D-glutamylglycine)]-L-lysyl-D-alanyl-N-alpha-[L-alanyl-gamma-(alpha-D-glutamylglycine)]-L-lysyl-D-alanine. Biochemistry. 1968 Apr;7(4):1450–1460. doi: 10.1021/bi00844a030. [DOI] [PubMed] [Google Scholar]
- Izaki K., Matsuhashi M., Strominger J. L. Biosynthesis of the peptidoglycan of bacterial cell walls. 8. Peptidoglycan transpeptidase and D-alanine carboxypeptidase: penicillin-sensitive enzymatic reaction in strains of Escherichia coli. J Biol Chem. 1968 Jun 10;243(11):3180–3192. [PubMed] [Google Scholar]
- Izaki K., Strominger J. L. Biosynthesis of the peptidoglycan of bacterial cell walls. XIV. Purification and properties of two D-alanine carboxypeptidases from Escherichia coli. J Biol Chem. 1968 Jun 10;243(11):3193–3201. [PubMed] [Google Scholar]
- Larson D. M., Snetsinger D. C., Waibel P. E. Procedure for determination of D-amino acids. Anal Biochem. 1971 Feb;39(2):395–401. doi: 10.1016/0003-2697(71)90429-5. [DOI] [PubMed] [Google Scholar]
- Leyh-Bouille M., Ghuysen J. M., Bonaly R., Nieto M., Perkins H. R., Schleifer K. H., Kandler O. Substrate requirements of the Streptomyces albus G DD carboxypeptidase. Biochemistry. 1970 Jul 21;9(15):2961–2970. doi: 10.1021/bi00817a005. [DOI] [PubMed] [Google Scholar]
- NEUHAUS F. C., STRUVE W. G. ENZYMATIC SYNTHESIS OF ANALOGS OF THE CELL-WALL PRECURSOR. I. KINETICS AND SPECIFICITY OF URIDINE DIPHOSPHO-N-ACETYLMURAMYL-L-ALANYL-D-GLUTAMYL-L-LYSINE:D-ALANYL-D-ALANINE LIGASE (ADENOSINE DIPHOSPHATE) FROM STREPTOCOCCUS FAECALIS R. Biochemistry. 1965 Jan;4:120–131. doi: 10.1021/bi00877a020. [DOI] [PubMed] [Google Scholar]
- NEUHAUS F. C. The enzymatic synthesis of D-alanyl-D-alanine. I. Purification and properties of D-alanyl-D-alanine synthetase. J Biol Chem. 1962 Mar;237:778–786. [PubMed] [Google Scholar]
- Niebler E., Schleifer K. H., Kandler O. The amino acid sequence of the L-glutamic acid containing mureins of Micrococcus luteus and M. freudenreichii. Biochem Biophys Res Commun. 1969 Mar 10;34(5):560–568. doi: 10.1016/0006-291x(69)90774-8. [DOI] [PubMed] [Google Scholar]
- Plapp R., Kandler O. Die Aminosäuresequenz des Asparaginsäure enthaltenden Mureins von Lactobacillus coryniformis und Lactobacillus cellobiosus. Z Naturforsch B. 1967 Oct;22(10):1062–1067. [PubMed] [Google Scholar]
- Plapp R., Kandler O. Identification of L-ornithine and delta-aminosuccinyl ornithine in cell wall hydrolysates of Lactobacillus cellobiosus. Nature. 1967 Feb 25;213(5078):803–804. doi: 10.1038/213803a0. [DOI] [PubMed] [Google Scholar]
- Plapp R., Kandler O. Isolation of an ornithine-containing cell wall precursor of Lactobacillus cellobiosus. Biochem Biophys Res Commun. 1967 Jul 21;28(2):141–145. doi: 10.1016/0006-291x(67)90420-2. [DOI] [PubMed] [Google Scholar]
- Pollock J. J., Ghuysen J. M., Linder R., Salton M. R., Perkins H. R., Nieto M., Leyh-Bouille M., Frere J. M., Johnson K. Transpeptidase activity of Streptomyces D-alanyl-D carboxypeptidases. Proc Natl Acad Sci U S A. 1972 Mar;69(3):662–666. doi: 10.1073/pnas.69.3.662. [DOI] [PMC free article] [PubMed] [Google Scholar]
- REISSIG J. L., STORMINGER J. L., LELOIR L. F. A modified colorimetric method for the estimation of N-acetylamino sugars. J Biol Chem. 1955 Dec;217(2):959–966. [PubMed] [Google Scholar]
- ROSENTHAL S., SHARON N. THE USE OF SEPHADEX G-25 FOR THE ISOLATION OF NUCLEOTIDE SUGAR DERIVATIVES FROM MICROCOCCUS LYSODEIKTICUS. Biochim Biophys Acta. 1964 Nov 1;83:378–380. doi: 10.1016/0926-6526(64)90025-4. [DOI] [PubMed] [Google Scholar]
- RYTER A., KELLENBERGER E., BIRCHANDERSEN A., MAALOE O. Etude au microscope électronique de plasmas contenant de l'acide désoxyribonucliéique. I. Les nucléoides des bactéries en croissance active. Z Naturforsch B. 1958 Sep;13B(9):597–605. [PubMed] [Google Scholar]
- STROMINGER J. L., BIRGE C. H. NUCLEOTIDE ACCUMULATION INDUCED IN STAPHYLOCOCCUS AUREUS BY GLYCINE. J Bacteriol. 1965 Apr;89:1124–1127. doi: 10.1128/jb.89.4.1124-1127.1965. [DOI] [PMC free article] [PubMed] [Google Scholar]
- Schleifer K. H., Huss L., Kandler O. Die Beeinflussung der Aminosäuresequenz des serinhaltigen Mureins von Staphylococcus epidermidis Stamm 24 durch die Nährobodenzusammenstzung. Arch Mikrobiol. 1969;68(4):387–404. [PubMed] [Google Scholar]
- Schleifer K. H., Kandler O. Peptidoglycan types of bacterial cell walls and their taxonomic implications. Bacteriol Rev. 1972 Dec;36(4):407–477. doi: 10.1128/br.36.4.407-477.1972. [DOI] [PMC free article] [PubMed] [Google Scholar]
- Schleifer K. H., Kandler O. Zur chemischen Zusammensetzung der Zellwand der Streptokokken. I. Die Aminosäuresequenz des Mureins von Str. thermophilus und Str. faecalis. Arch Mikrobiol. 1967 Jul 6;57(4):335–364. [PubMed] [Google Scholar]
- Snell E. E., Guirard B. M. Some Interrelationships of Pyridoxine, Alanine and Glycine in Their Effect on Certain Lactic Acid Bacteria. Proc Natl Acad Sci U S A. 1943 Feb;29(2):66–73. doi: 10.1073/pnas.29.2.66. [DOI] [PMC free article] [PubMed] [Google Scholar]
- TAKEBE I. EXTENT OF CROSS LINKAGE IN THE MUREIN SACCULUS OF ESCHERICHIA COLI B CELL WALL. Biochim Biophys Acta. 1965 Mar 1;101:124–126. doi: 10.1016/0926-6534(65)90038-2. [DOI] [PubMed] [Google Scholar]
- Tipper D. J. Mechanism of autolysis of isolated cell walls of Staphylococcus aureus. J Bacteriol. 1969 Feb;97(2):837–847. doi: 10.1128/jb.97.2.837-847.1969. [DOI] [PMC free article] [PubMed] [Google Scholar]
- Vela G. R., Rosenthal R. S. Effect of peptone on Azotobacter morphology. J Bacteriol. 1972 Jul;111(1):260–266. doi: 10.1128/jb.111.1.260-266.1972. [DOI] [PMC free article] [PubMed] [Google Scholar]
- WEIDEL W., PELZER H. BAGSHAPED MACROMOLECULES--A NEW OUTLOOK ON BACTERIAL CELL WALLS. Adv Enzymol Relat Areas Mol Biol. 1964;26:193–232. doi: 10.1002/9780470122716.ch5. [DOI] [PubMed] [Google Scholar]
- WELSCH M. Formation de protoplastes d'Escherichia coli sous l'influence de la glycine et d'autres acides aminés. Schweiz Z Pathol Bakteriol. 1958;21(3):741–768. [PubMed] [Google Scholar]