Abstract
Normal red cells were incubated in the absence of glucose to develop a system in which total adenosine triphosphate (ATP) turnover could be assessed. After 1 hr, the triose pool had been completely consumed. Thereafter, the metabolism of 2,3-diphosphoglycerate (DPG) to pyruvate and lactate was the sole significant source of ATP synthesis.
10-3M CuCl2, which did not enter the cells, diminished ATP utilization by more than 50%. This could be only partially attributed to the inhibition by copper of residual acylation and cation pumping, which were already reduced by glucose depletion. Other membrane enzymes, which presumably function in the maintenance of membrane integrity, must, therefore, use a significant portion of erythrocyte ATP.
The behavior of glucose-depleted red cells with respect to cation transport was complex. The addition of ouabain did not decrease ATP utilization in these red cells. Ouabain inhibitable potassium influx was nearly normal after triose depletion, but total potassium influx was decreased. In contrast, the ouabain inhibitable sodium efflux was markedly reduced after triose depletion, although the concentration of ATP was 70% of normal. The dissociation of monovalent cation pumping suggests that the energy for active sodium transport is derived from a specific source (such as the ATP produced by the phosphoglycerate kinase reaction) distinct from that for potassium transport.
Full text
PDF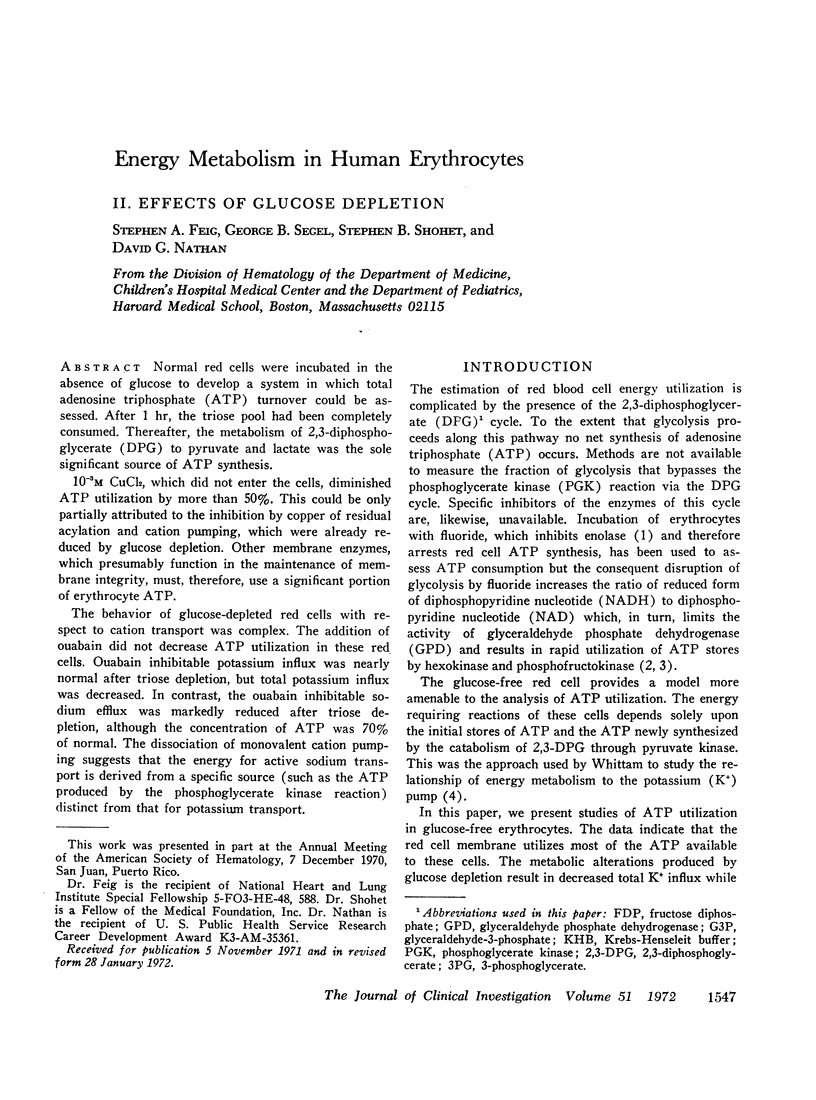
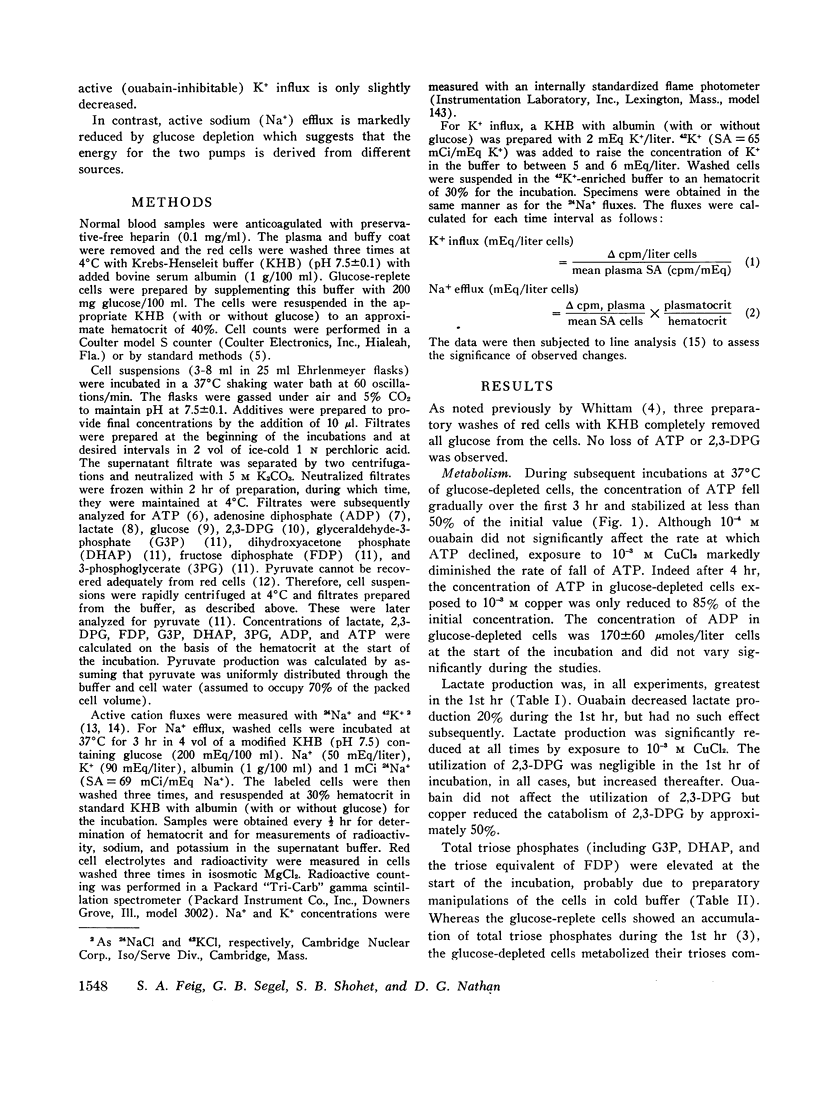
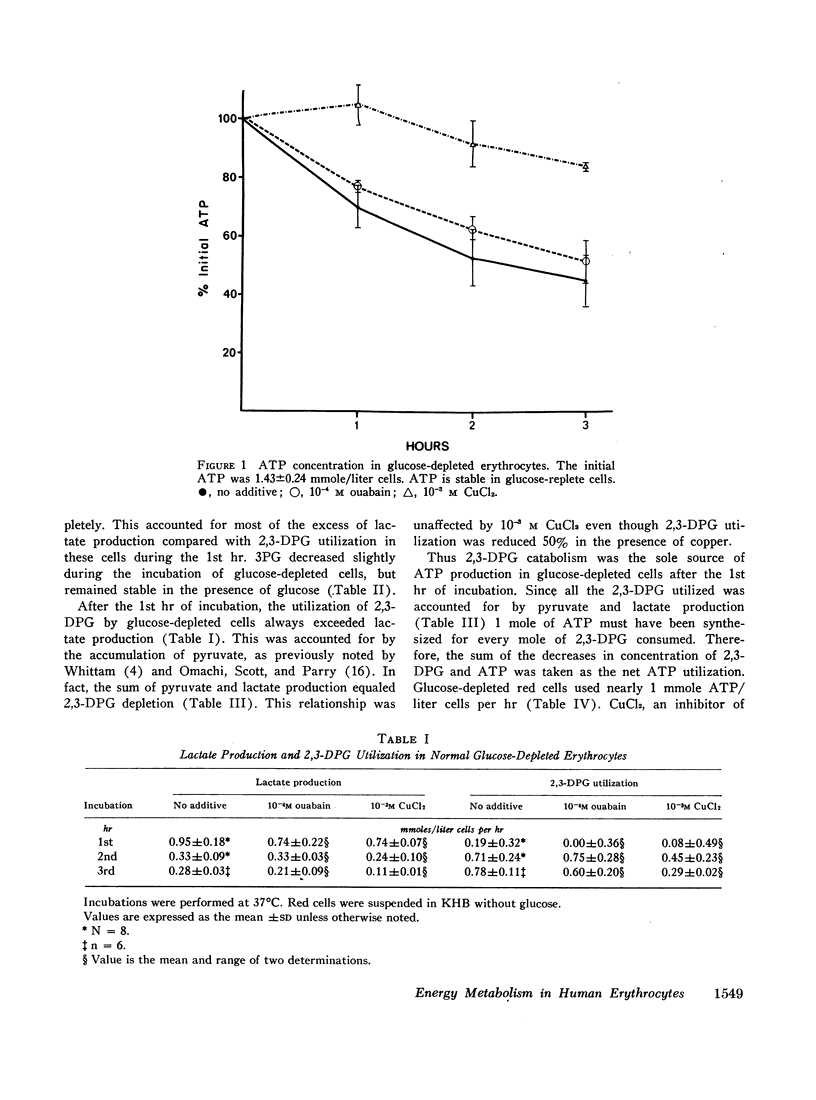
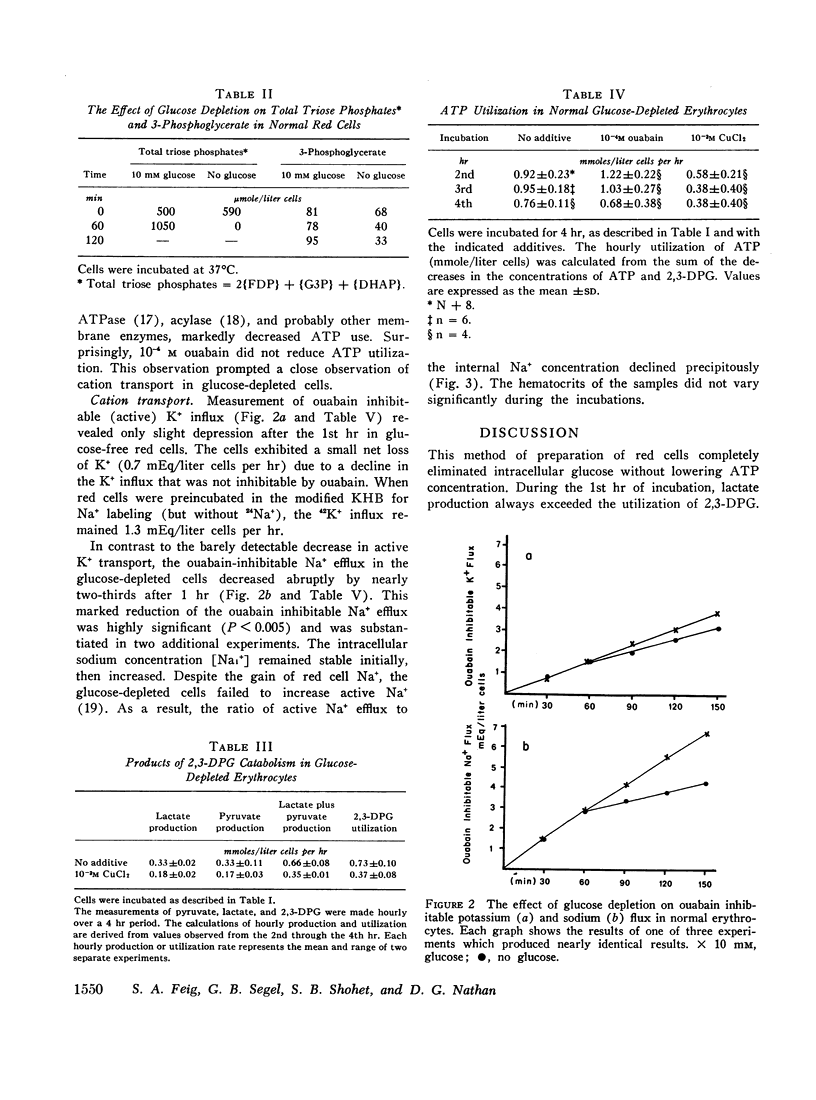
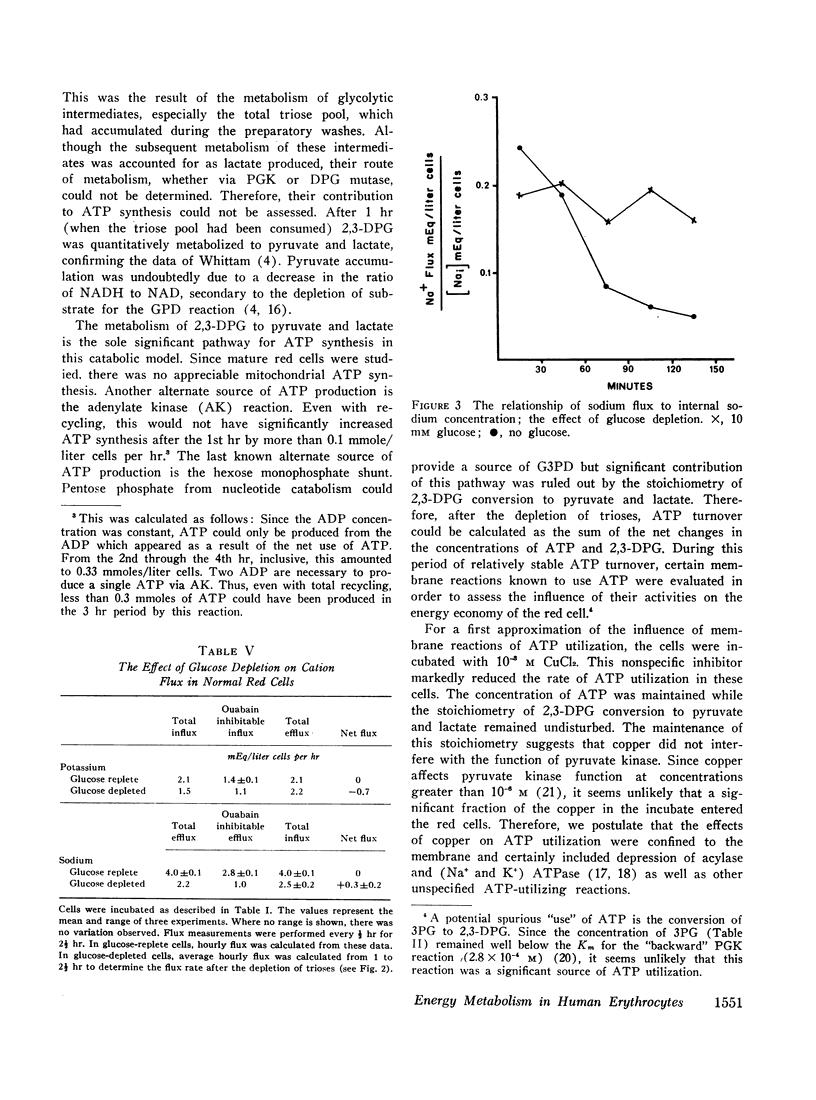
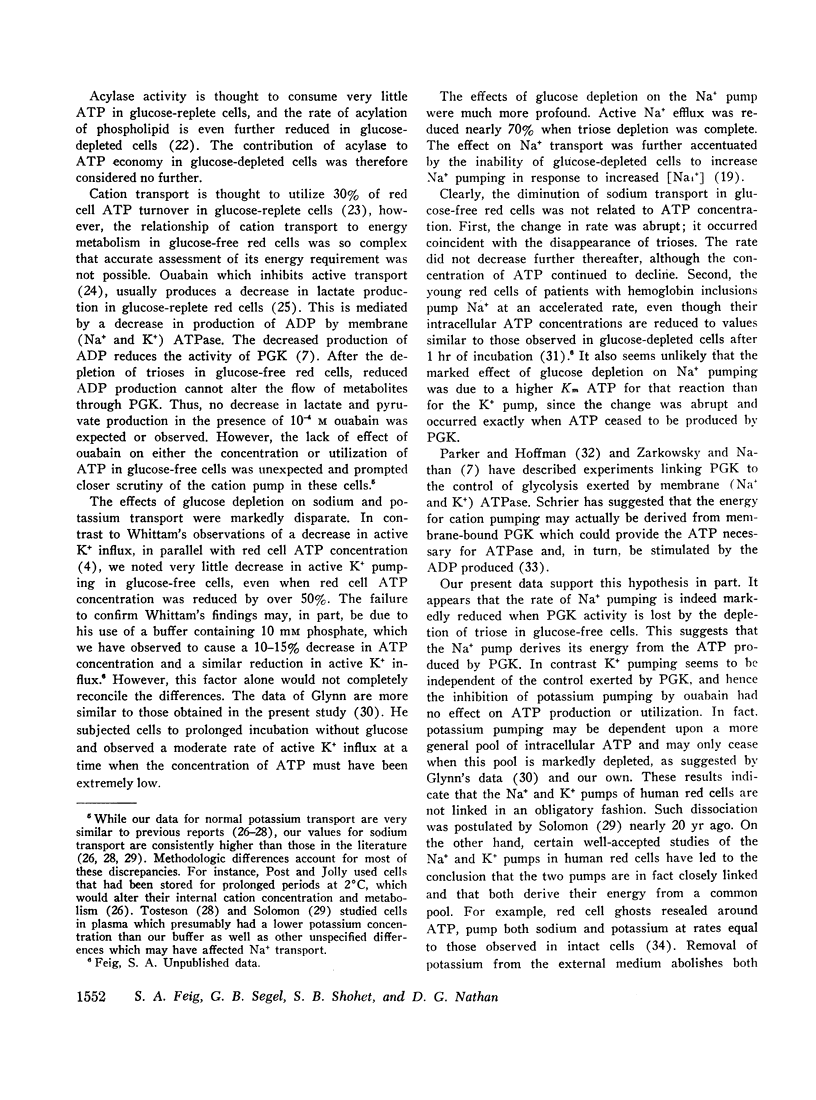
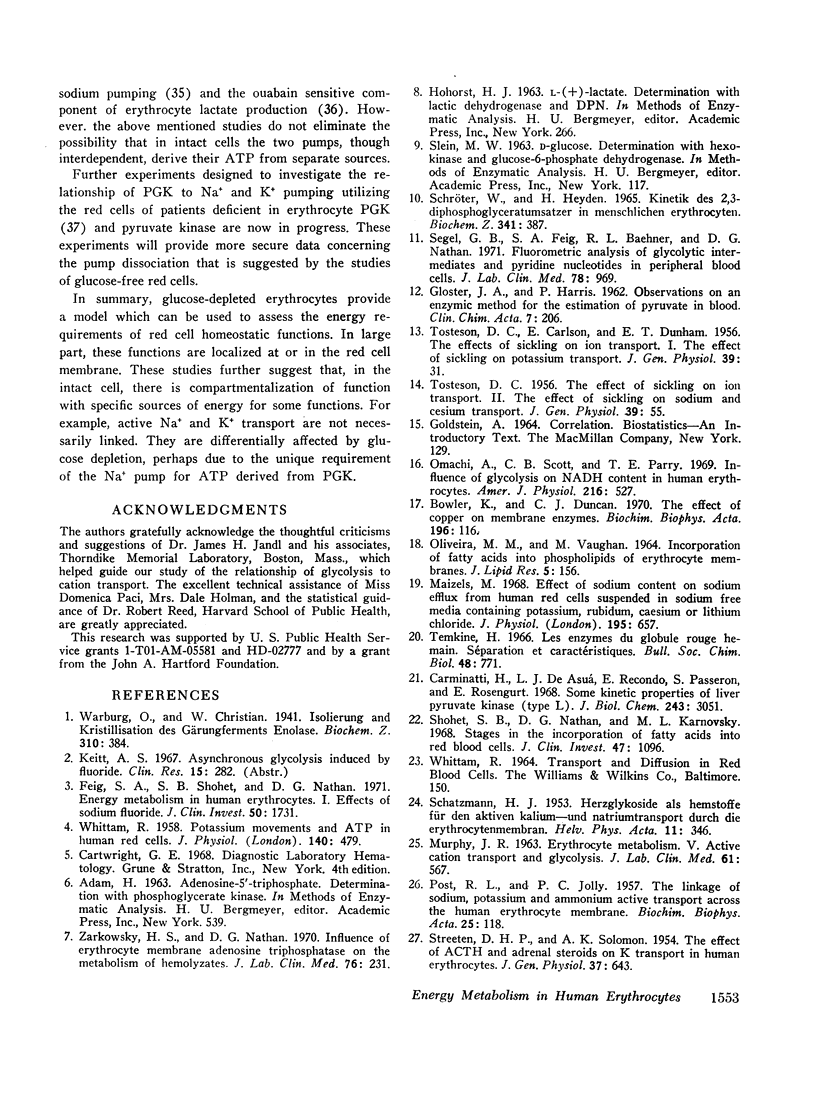
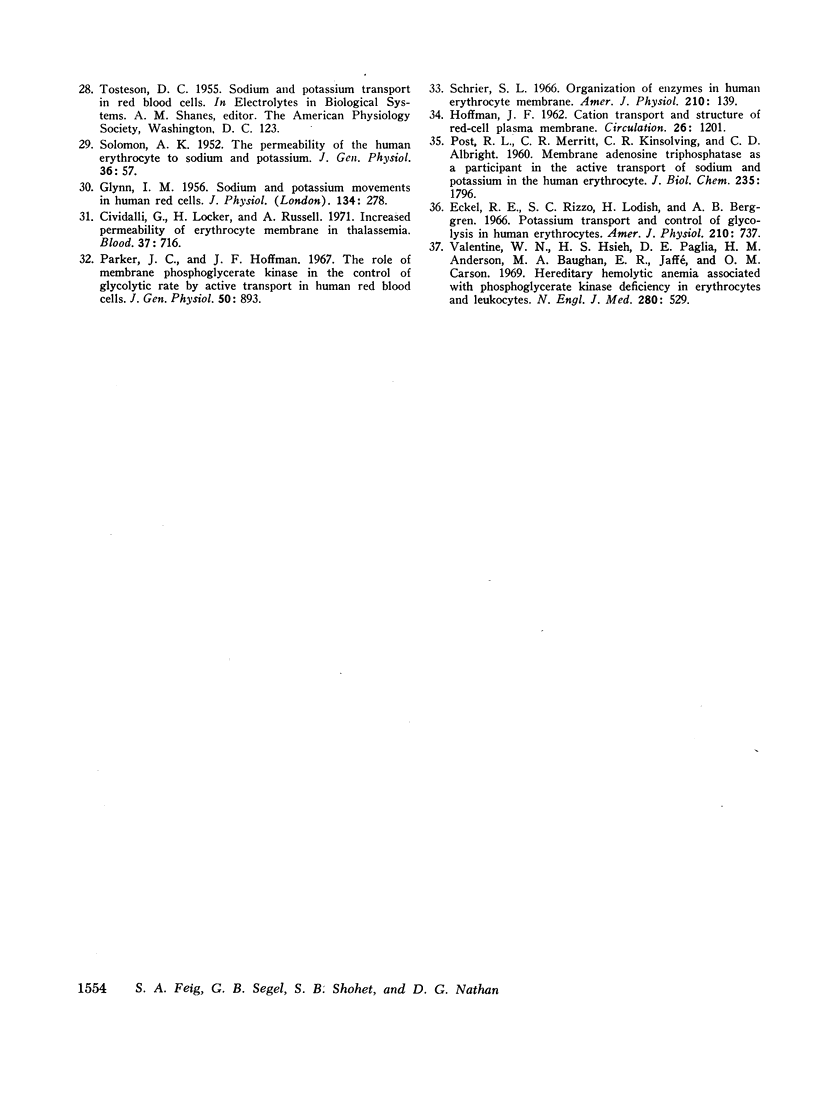
Selected References
These references are in PubMed. This may not be the complete list of references from this article.
- Bowler K., Duncan C. J. The effect of copper on membrane enzymes. Biochim Biophys Acta. 1970 Jan 6;196(1):116–119. doi: 10.1016/0005-2736(70)90174-4. [DOI] [PubMed] [Google Scholar]
- Carminatti H., Jiménez de Asúa L., Recondo E., Passeron S., Rozengurt E. Some kinetic properties of liver pyruvate kinase (type L). J Biol Chem. 1968 Jun 10;243(11):3051–3056. [PubMed] [Google Scholar]
- Cividalli G., Locker H., Russell A. Increased permeability of erythrocyte membrane in thalassemia. Blood. 1971 Jun;37(6):716–724. [PubMed] [Google Scholar]
- Eckel R. E., Rizzo S. C., Lodish H., Berggren A. B. Potassium transport and control of glycolysis in human erythrocytes. Am J Physiol. 1966 Apr;210(4):737–743. doi: 10.1152/ajplegacy.1966.210.4.737. [DOI] [PubMed] [Google Scholar]
- Feig S. A., Shohet S. B., Nathan D. G. Energy metabolism in human erythrocytes. I. Effects of sodium fluoride. J Clin Invest. 1971 Aug;50(8):1731–1737. doi: 10.1172/JCI106662. [DOI] [PMC free article] [PubMed] [Google Scholar]
- GLOSTER J. A., HARRIS P. Observations on an enzymic method for the estimation of pyruvate in blood. Clin Chim Acta. 1962 Mar;7:206–211. doi: 10.1016/0009-8981(62)90011-6. [DOI] [PubMed] [Google Scholar]
- GLYNN I. M. Sodium and potassium movements in human red cells. J Physiol. 1956 Nov 28;134(2):278–310. doi: 10.1113/jphysiol.1956.sp005643. [DOI] [PMC free article] [PubMed] [Google Scholar]
- MURPHY J. R. Erythrocyte metabolism. V. Active cation transport and glycolysis. J Lab Clin Med. 1963 Apr;61:567–577. [PubMed] [Google Scholar]
- Maizels M. Effect of sodium content on sodium efflux from human red cells suspended in sodium-free media containing potassium, rubidium, caesium or lithium chloride. J Physiol. 1968 Apr;195(3):657–679. doi: 10.1113/jphysiol.1968.sp008481. [DOI] [PMC free article] [PubMed] [Google Scholar]
- OLIVEIRA M. M., VAUGHAN M. INCORPORATION OF FATTY ACIDS INTO PHOSPHOLIPIDS OF ERYTHROCYTE MEMBRANES. J Lipid Res. 1964 Apr;5:156–162. [PubMed] [Google Scholar]
- Omachi A., Scott C. B., Parry T. E. Influence of glycolysis on NADH content in human erythrocytes. Am J Physiol. 1969 Mar;216(3):527–530. doi: 10.1152/ajplegacy.1969.216.3.527. [DOI] [PubMed] [Google Scholar]
- POST R. L., JOLLY P. C. The linkage of sodium, potassium, and ammonium active transport across the human erythrocyte membrane. Biochim Biophys Acta. 1957 Jul;25(1):118–128. doi: 10.1016/0006-3002(57)90426-2. [DOI] [PubMed] [Google Scholar]
- POST R. L., MERRITT C. R., KINSOLVING C. R., ALBRIGHT C. D. Membrane adenosine triphosphatase as a participant in the active transport of sodium and potassium in the human erythrocyte. J Biol Chem. 1960 Jun;235:1796–1802. [PubMed] [Google Scholar]
- Parker J. C., Hoffman J. F. The role of membrane phosphoglycerate kinase in the control of glycolytic rate by active cation transport in human red blood cells. J Gen Physiol. 1967 Mar;50(4):893–916. doi: 10.1085/jgp.50.4.893. [DOI] [PMC free article] [PubMed] [Google Scholar]
- SCHATZMANN H. J. Herzglykoside als Hemmstoffe für den aktiven Kalium- und Natriumtransport durch die Erythrocytenmembran. Helv Physiol Pharmacol Acta. 1953;11(4):346–354. [PubMed] [Google Scholar]
- SOLOMON A. K. The permeability of the human erythrocyte to sodium and potassium. J Gen Physiol. 1952 May;36(1):57–110. doi: 10.1085/jgp.36.1.57. [DOI] [PMC free article] [PubMed] [Google Scholar]
- STREETEN D. H., SOLOMON A. K. The effect of ACTH and adrenal steroids on K transport in human erythrocytes. J Gen Physiol. 1954 May 20;37(5):643–661. doi: 10.1085/jgp.37.5.643. [DOI] [PMC free article] [PubMed] [Google Scholar]
- Schrier S. L. Organization of enzymes in human erythrocyte membranes. Am J Physiol. 1966 Jan;210(1):139–145. doi: 10.1152/ajplegacy.1966.210.1.139. [DOI] [PubMed] [Google Scholar]
- Segel G. B., Feig S. A., Baehner R. L., Nathan D. G. Fluorometric analysis of glycolytic intermediates and pyridine nucleotides in peripheral blood cells. J Lab Clin Med. 1971 Dec;78(6):969–976. [PubMed] [Google Scholar]
- Shohet S. B., Nathan D. G., Karnovsky M. L. Stages in the incorporation of fatty acids into red blood cells. J Clin Invest. 1968 May;47(5):1096–1108. doi: 10.1172/JCI105799. [DOI] [PMC free article] [PubMed] [Google Scholar]
- TOSTESON D. C., CARLSEN E., DUNHAM E. T. The effects of sickling on ion transport. I. Effect of sickling on potassium transport. J Gen Physiol. 1955 Sep 20;39(1):31–53. doi: 10.1085/jgp.39.1.31. [DOI] [PMC free article] [PubMed] [Google Scholar]
- TOSTESON D. C. The effects of sickling on ion transport. II. The effect of sickling on sodium and cesium transport. J Gen Physiol. 1955 Sep 20;39(1):55–67. doi: 10.1085/jgp.39.1.55. [DOI] [PMC free article] [PubMed] [Google Scholar]
- Temkine H. Les enzymes du globule rouge humain. Séparation et caractéristiques. Bull Soc Chim Biol (Paris) 1966;48(6):771–786. [PubMed] [Google Scholar]
- WHITTAM R. Potassium movements and ATP in human red cells. J Physiol. 1958 Mar 11;140(3):479–497. [PMC free article] [PubMed] [Google Scholar]
- Zarkowsky H. S., Nathan D. G. Influence of erythrocyte membrane adenosine triphosphatase on the metabolism of hemolysates. J Lab Clin Med. 1970 Aug;76(2):231–239. [PubMed] [Google Scholar]