Abstract
Free radicals have been implicated in the pathogenesis of reperfusion injury, but it is unclear how they exert their deleterious effects on cellular metabolism. Several lines of indirect evidence suggest that free radicals elevate intracellular Ca2+ concentration ([Ca2+]i) and inhibit glycolysis as part of their mechanism of injury. We tested these ideas directly in hearts subjected to hydroxyl radicals produced by the Fenton and Haber-Weiss reactions. Nuclear magnetic resonance spectra were obtained from Langendorff-perfused rabbit hearts before, during, and after 4 min of perfusion with H2O2 (0.75 mM) and Fe(3+)-chelate (0.1 mM). Isovolumic left ventricular pressure exhibited progressive functional deterioration and contracture after exposure to H2O2 + Fe3+. Phosphorus nuclear magnetic resonance (NMR) spectra revealed partial ATP depletion and sugar phosphate accumulation indicative of glycolytic inhibition. To measure [Ca2+]i, fluorine NMR spectra were acquired in a separate group of hearts loaded with the Ca2+ indicator 5F-BAPTA [5,5'-difluoro derivative of 1,2-bis-(o-aminophenoxy)ethane- N,N,N',N'-tetraacetic acid]. Mean time-averaged [Ca2+]i increased from 347 +/- 14 nM in control to 1,026 +/- 295 nM 4 min after free radical generation (means +/- SEM, n = 7), and remained elevated thereafter. We conclude that free radicals induce clear-cut, specific derangements of cellular metabolism in the form of glycolytic inhibition and calcium overload. The observed increase in [Ca2+]i suggests that the deleterious effects of free radicals are at least partially mediated by secondary changes in cellular calcium homeostasis.
Full text
PDF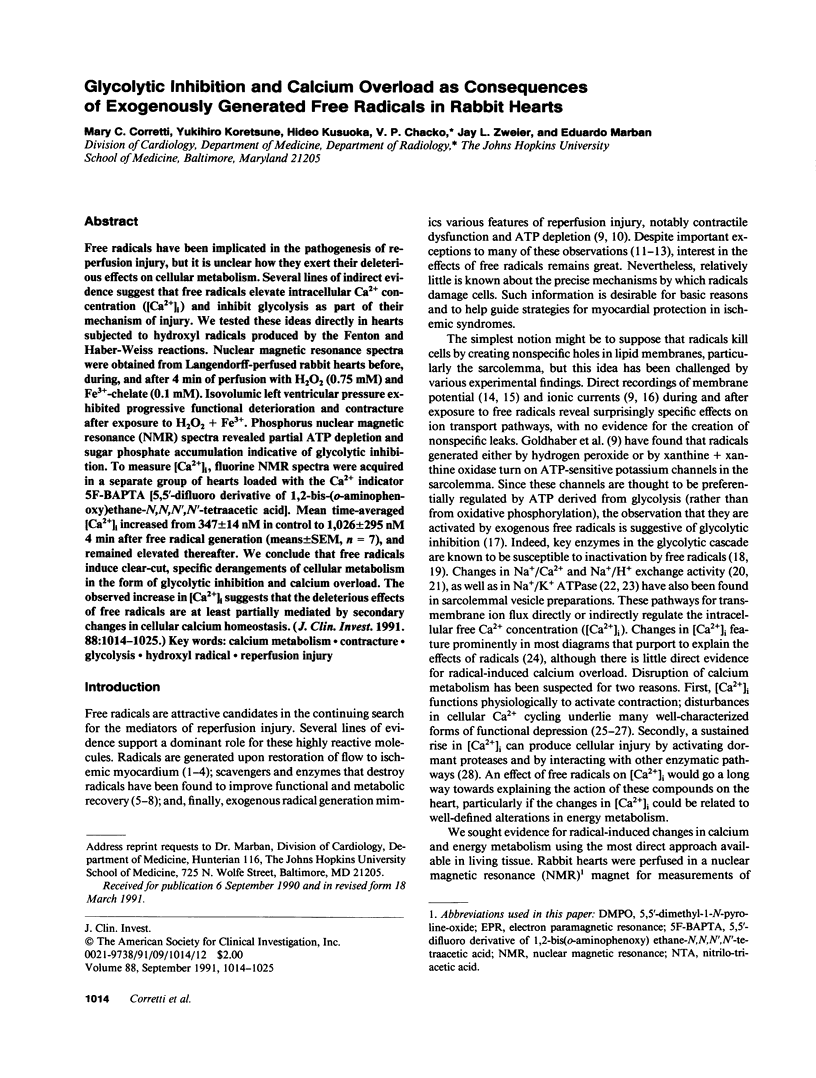
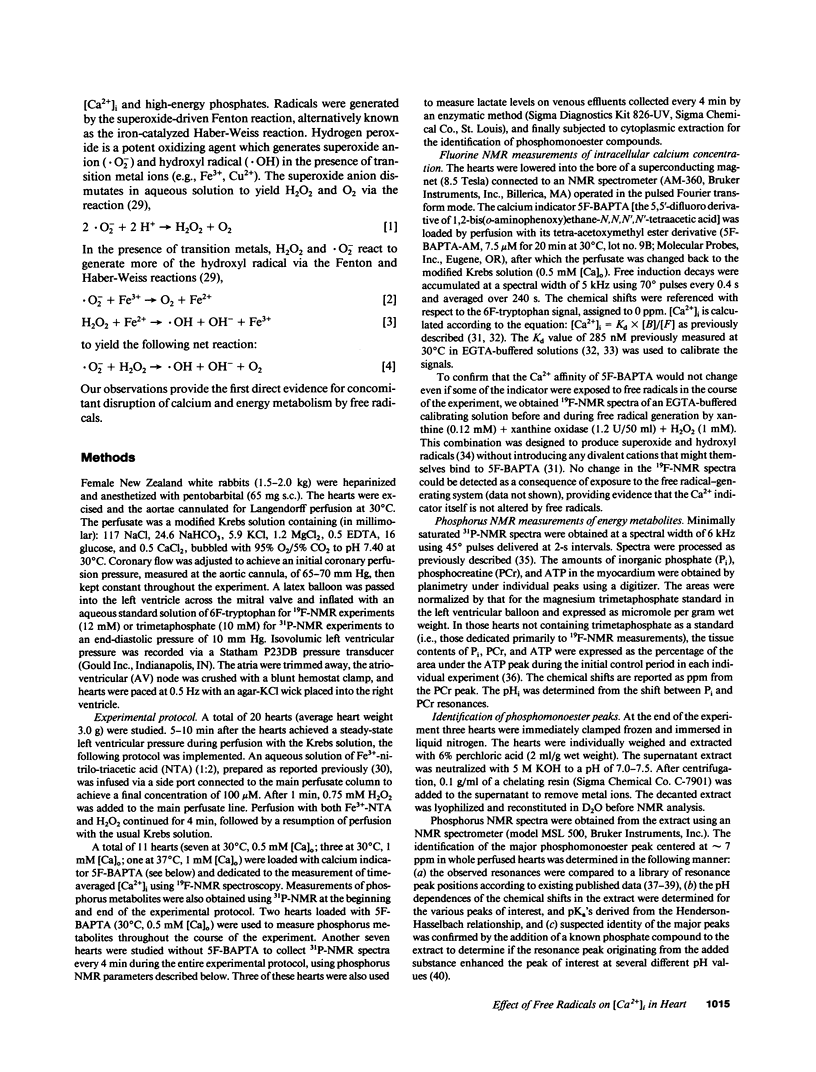
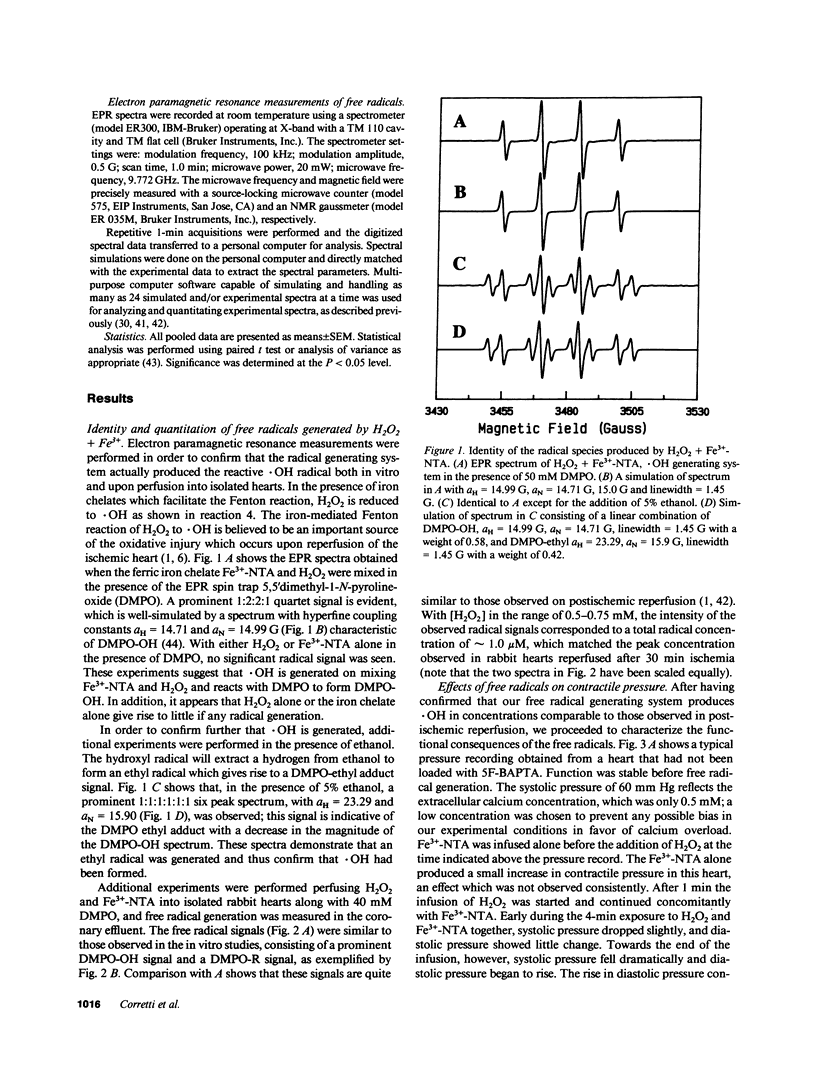
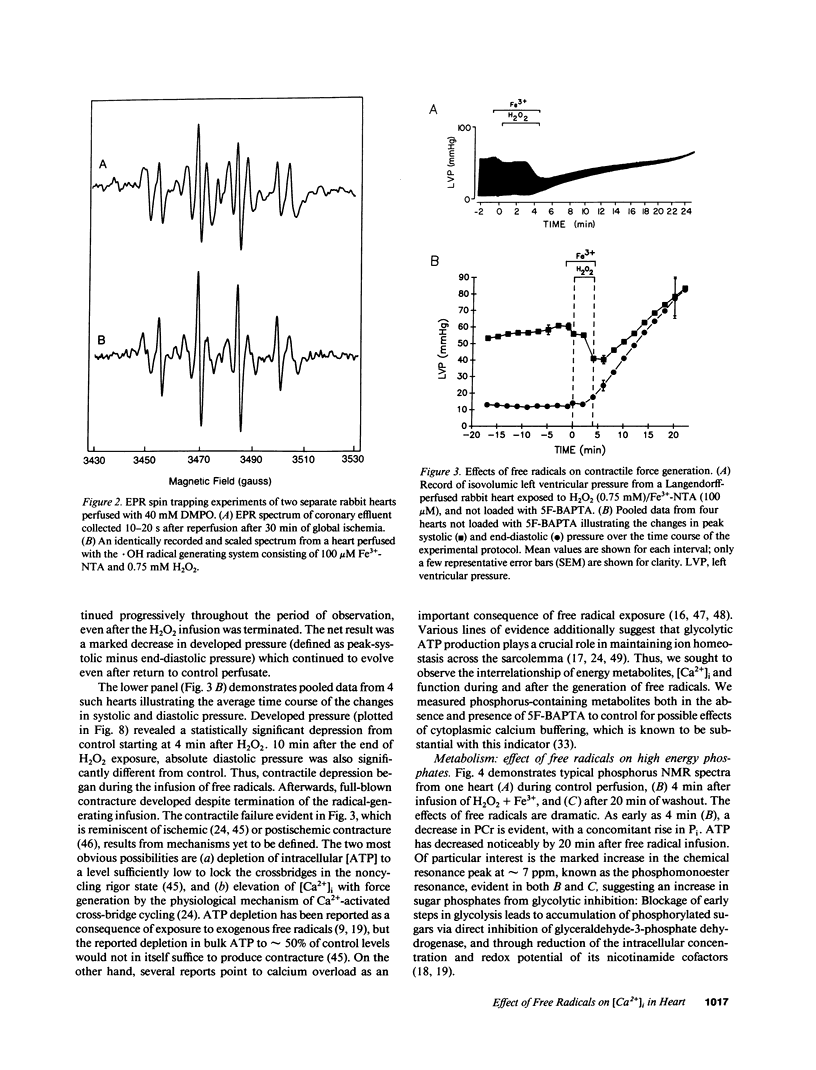
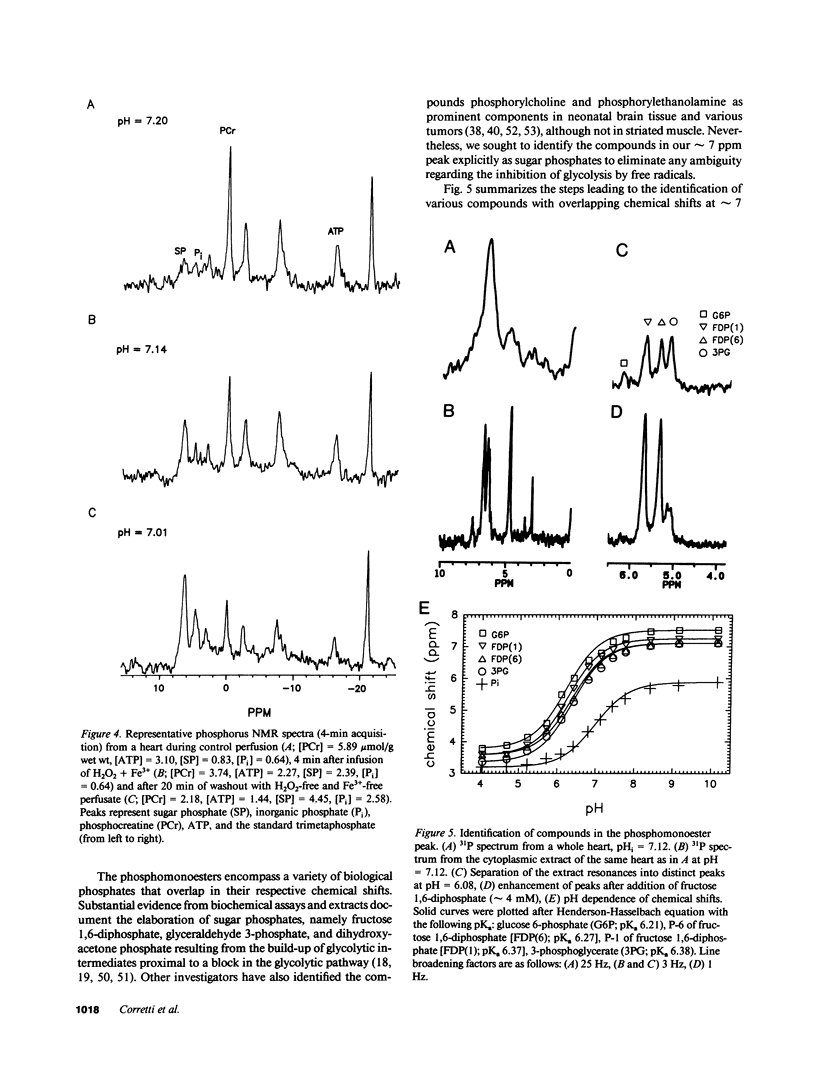
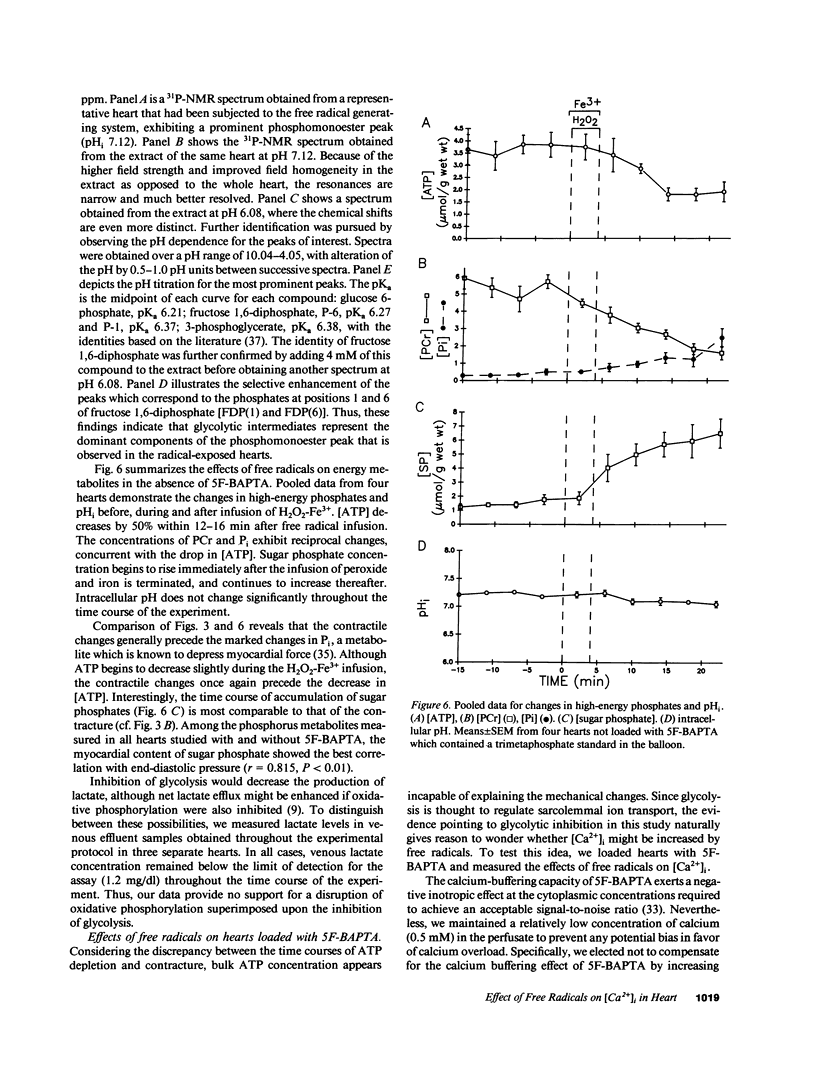
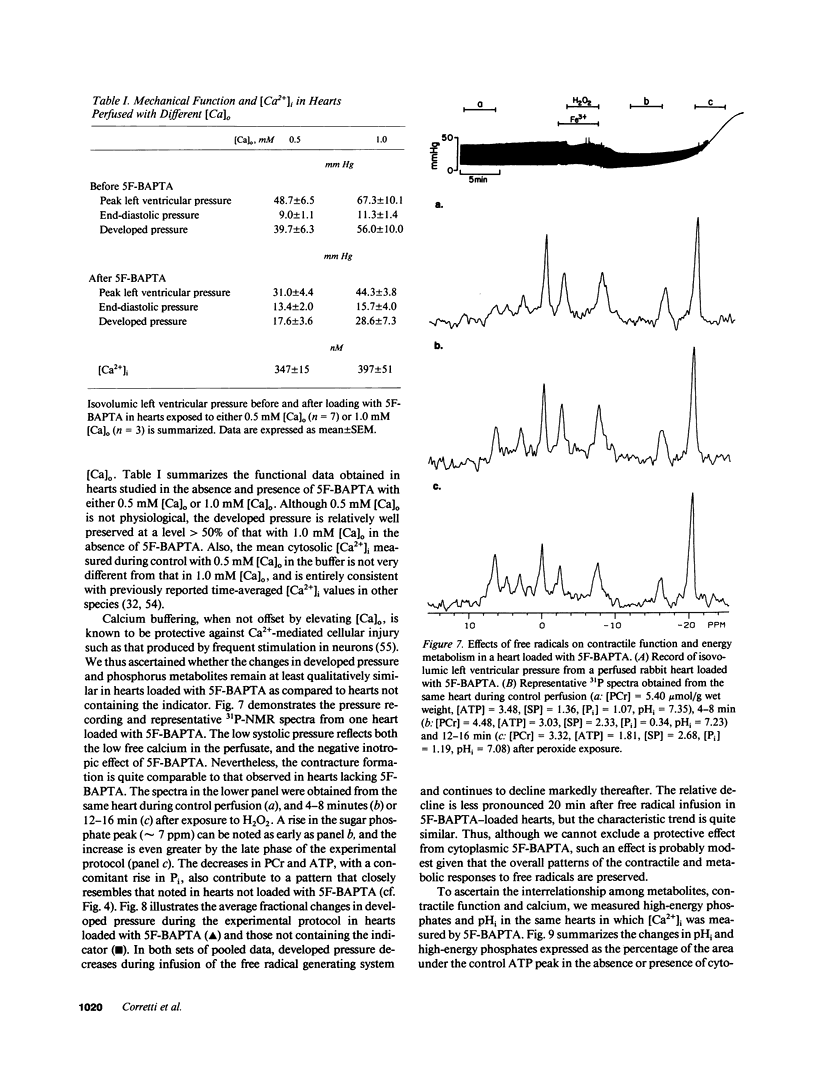
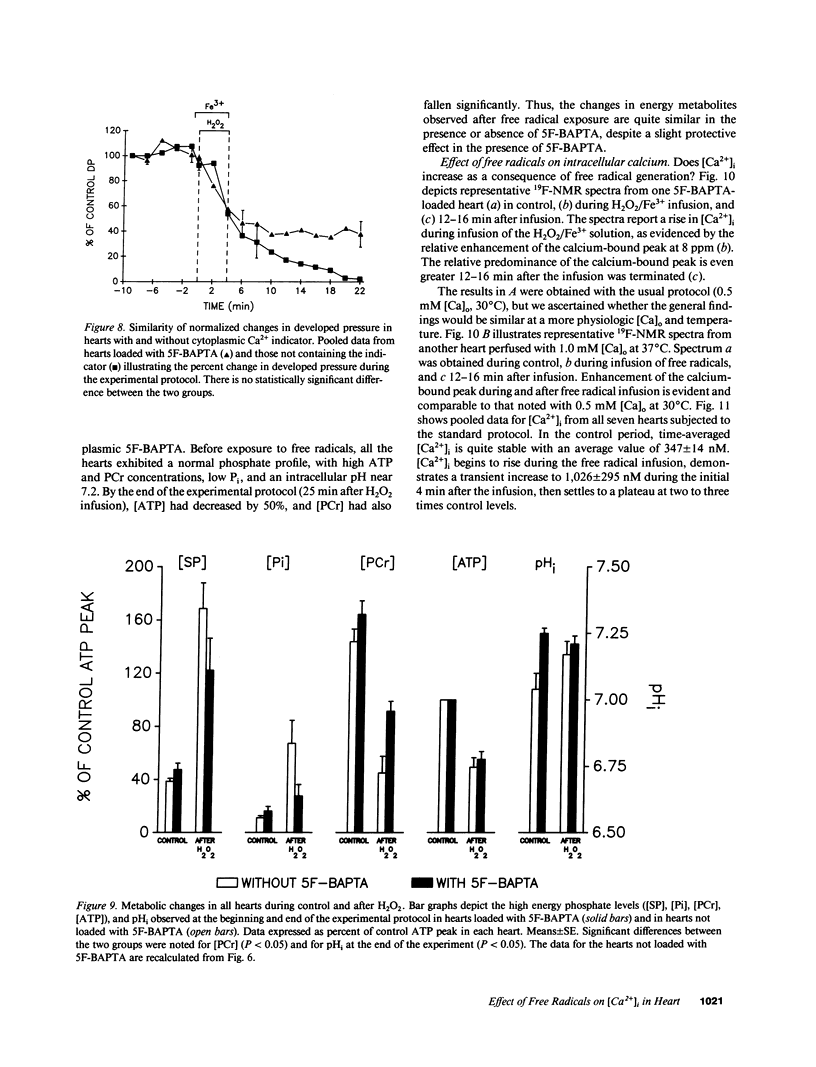
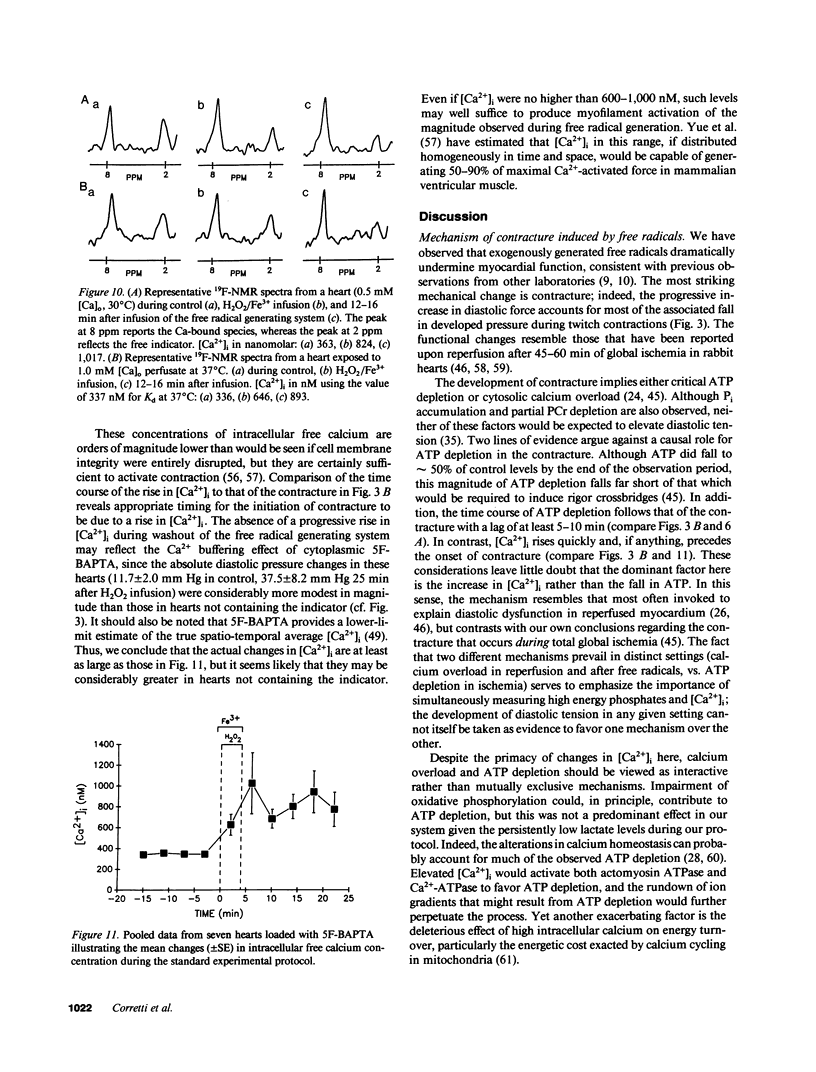
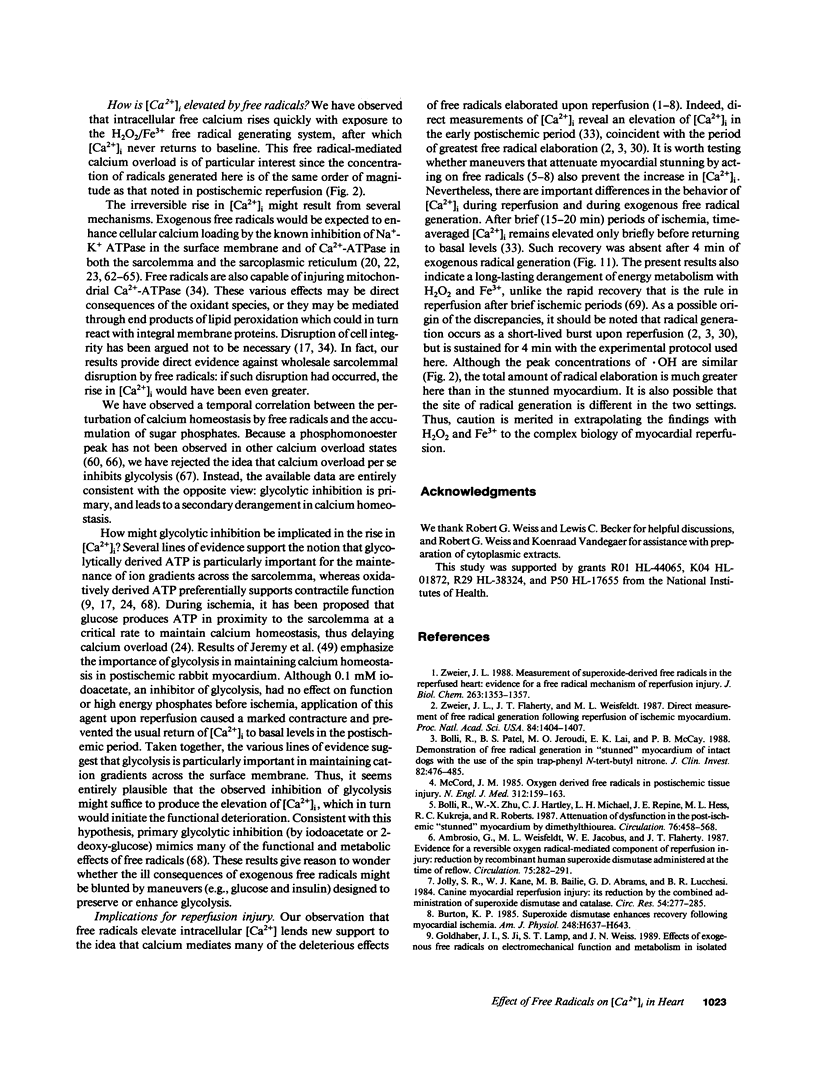
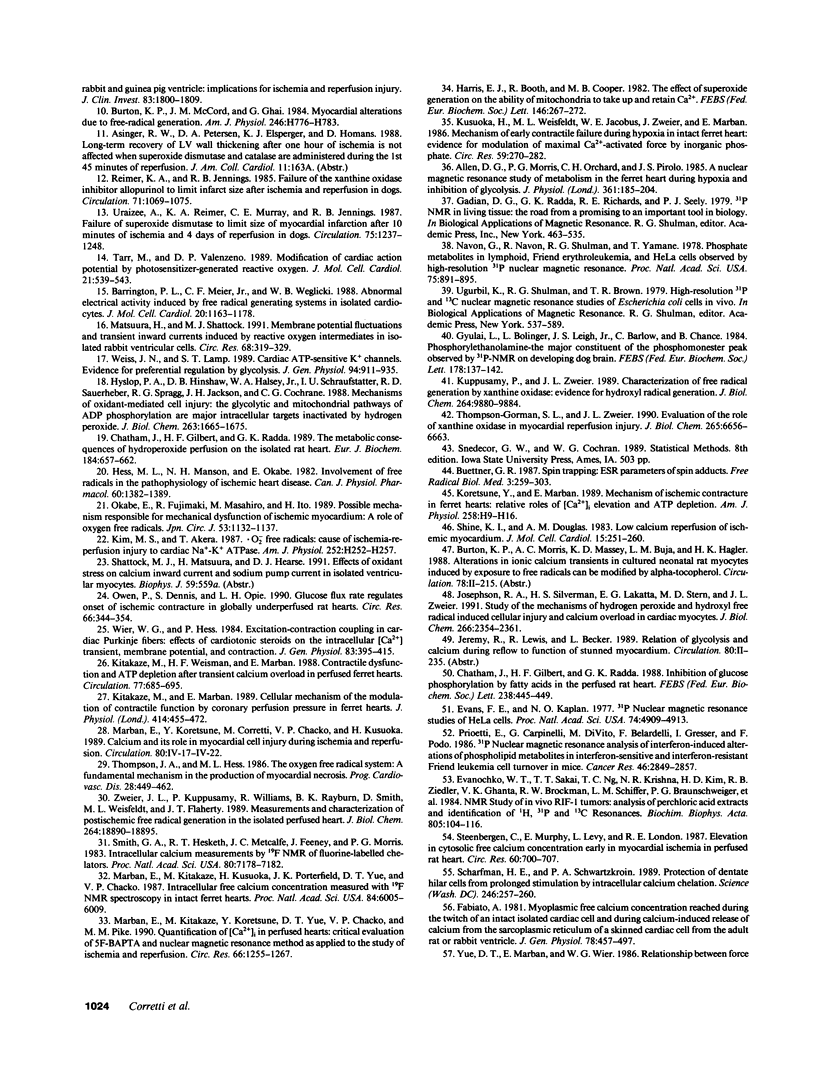
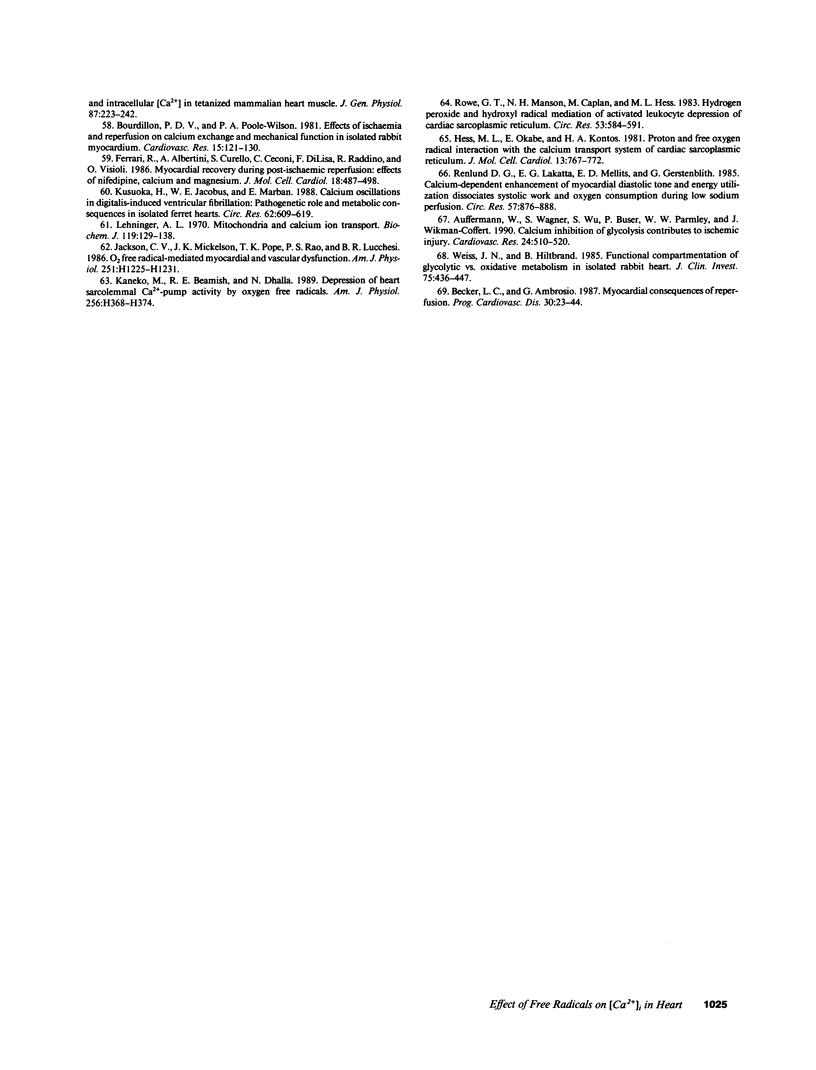
Selected References
These references are in PubMed. This may not be the complete list of references from this article.
- Allen D. G., Morris P. G., Orchard C. H., Pirolo J. S. A nuclear magnetic resonance study of metabolism in the ferret heart during hypoxia and inhibition of glycolysis. J Physiol. 1985 Apr;361:185–204. doi: 10.1113/jphysiol.1985.sp015640. [DOI] [PMC free article] [PubMed] [Google Scholar]
- Ambrosio G., Weisfeldt M. L., Jacobus W. E., Flaherty J. T. Evidence for a reversible oxygen radical-mediated component of reperfusion injury: reduction by recombinant human superoxide dismutase administered at the time of reflow. Circulation. 1987 Jan;75(1):282–291. doi: 10.1161/01.cir.75.1.282. [DOI] [PubMed] [Google Scholar]
- Auffermann W., Wagner S., Wu S., Buser P., Parmley W. W., Wikman-Coffelt J. Calcium inhibition of glycolysis contributes to ischaemic injury. Cardiovasc Res. 1990 Jun;24(6):510–520. doi: 10.1093/cvr/24.6.510. [DOI] [PubMed] [Google Scholar]
- Barrington P. L., Meier C. F., Jr, Weglicki W. B. Abnormal electrical activity induced by free radical generating systems in isolated cardiocytes. J Mol Cell Cardiol. 1988 Dec;20(12):1163–1178. doi: 10.1016/0022-2828(88)90596-2. [DOI] [PubMed] [Google Scholar]
- Becker L. C., Ambrosio G. Myocardial consequences of reperfusion. Prog Cardiovasc Dis. 1987 Jul-Aug;30(1):23–44. doi: 10.1016/0033-0620(87)90009-0. [DOI] [PubMed] [Google Scholar]
- Bolli R., Patel B. S., Jeroudi M. O., Lai E. K., McCay P. B. Demonstration of free radical generation in "stunned" myocardium of intact dogs with the use of the spin trap alpha-phenyl N-tert-butyl nitrone. J Clin Invest. 1988 Aug;82(2):476–485. doi: 10.1172/JCI113621. [DOI] [PMC free article] [PubMed] [Google Scholar]
- Bolli R., Zhu W. X., Hartley C. J., Michael L. H., Repine J. E., Hess M. L., Kukreja R. C., Roberts R. Attenuation of dysfunction in the postischemic 'stunned' myocardium by dimethylthiourea. Circulation. 1987 Aug;76(2):458–468. doi: 10.1161/01.cir.76.2.458. [DOI] [PubMed] [Google Scholar]
- Bourdillon P. D., Poole-Wilson P. A. Effects of ischaemia and reperfusion on calcium exchange and mechanical function in isolated rabbit myocardium. Cardiovasc Res. 1981 Mar;15(3):121–130. doi: 10.1093/cvr/15.3.121. [DOI] [PubMed] [Google Scholar]
- Buettner G. R. Spin trapping: ESR parameters of spin adducts. Free Radic Biol Med. 1987;3(4):259–303. doi: 10.1016/s0891-5849(87)80033-3. [DOI] [PubMed] [Google Scholar]
- Burton K. P., McCord J. M., Ghai G. Myocardial alterations due to free-radical generation. Am J Physiol. 1984 Jun;246(6 Pt 2):H776–H783. doi: 10.1152/ajpheart.1984.246.6.H776. [DOI] [PubMed] [Google Scholar]
- Burton K. P. Superoxide dismutase enhances recovery following myocardial ischemia. Am J Physiol. 1985 May;248(5 Pt 2):H637–H643. doi: 10.1152/ajpheart.1985.248.5.H637. [DOI] [PubMed] [Google Scholar]
- Chatham J. C., Gilbert H. F., Radda G. K. The metabolic consequences of hydroperoxide perfusion on the isolated rat heart. Eur J Biochem. 1989 Oct 1;184(3):657–662. doi: 10.1111/j.1432-1033.1989.tb15063.x. [DOI] [PubMed] [Google Scholar]
- Chatham J., Gilbert H. F., Radda G. K. Inhibition of glucose phosphorylation by fatty acids in the perfused rat heart. FEBS Lett. 1988 Oct 10;238(2):445–449. doi: 10.1016/0014-5793(88)80529-5. [DOI] [PubMed] [Google Scholar]
- Evanochko W. T., Sakai T. T., Ng T. C., Krishna N. R., Kim H. D., Zeidler R. B., Ghanta V. K., Brockman R. W., Schiffer L. M., Braunschweiger P. G. NMR study of in vivo RIF-1 tumors. Analysis of perchloric acid extracts and identification of 1H, 31P and 13C resonances. Biochim Biophys Acta. 1984 Sep 14;805(1):104–116. doi: 10.1016/0167-4889(84)90042-9. [DOI] [PubMed] [Google Scholar]
- Evans F. E., Kaplan N. O. 31P nuclear magnetic resonance studies of HeLa cells. Proc Natl Acad Sci U S A. 1977 Nov;74(11):4909–4913. doi: 10.1073/pnas.74.11.4909. [DOI] [PMC free article] [PubMed] [Google Scholar]
- Fabiato A. Myoplasmic free calcium concentration reached during the twitch of an intact isolated cardiac cell and during calcium-induced release of calcium from the sarcoplasmic reticulum of a skinned cardiac cell from the adult rat or rabbit ventricle. J Gen Physiol. 1981 Nov;78(5):457–497. doi: 10.1085/jgp.78.5.457. [DOI] [PMC free article] [PubMed] [Google Scholar]
- Ferrari R., Albertini A., Curello S., Ceconi C., Di Lisa F., Raddino R., Visioli O. Myocardial recovery during post-ischaemic reperfusion: effects of nifedipine, calcium and magnesium. J Mol Cell Cardiol. 1986 May;18(5):487–498. doi: 10.1016/s0022-2828(86)80914-2. [DOI] [PubMed] [Google Scholar]
- Goldhaber J. I., Ji S., Lamp S. T., Weiss J. N. Effects of exogenous free radicals on electromechanical function and metabolism in isolated rabbit and guinea pig ventricle. Implications for ischemia and reperfusion injury. J Clin Invest. 1989 Jun;83(6):1800–1809. doi: 10.1172/JCI114085. [DOI] [PMC free article] [PubMed] [Google Scholar]
- Gyulai L., Bolinger L., Leigh J. S., Jr, Barlow C., Chance B. Phosphorylethanolamine--the major constituent of the phosphomonoester peak observed by 31P-NMR on developing dog brain. FEBS Lett. 1984 Dec 3;178(1):137–142. doi: 10.1016/0014-5793(84)81257-0. [DOI] [PubMed] [Google Scholar]
- Harris E. J., Booth R., Cooper M. B. The effect of superoxide generation on the ability of mitochondria to take up and retain Ca2+. FEBS Lett. 1982 Sep 20;146(2):267–272. doi: 10.1016/0014-5793(82)80932-0. [DOI] [PubMed] [Google Scholar]
- Hess M. L., Manson N. H., Okabe E. Involvement of free radicals in the pathophysiology of ischemic heart disease. Can J Physiol Pharmacol. 1982 Nov;60(11):1382–1389. doi: 10.1139/y82-206. [DOI] [PubMed] [Google Scholar]
- Hess M. L., Okabe E., Kontos H. A. Proton and free oxygen radical interaction with the calcium transport system of cardiac sarcoplasmic reticulum. J Mol Cell Cardiol. 1981 Aug;13(8):767–772. doi: 10.1016/0022-2828(81)90258-3. [DOI] [PubMed] [Google Scholar]
- Hyslop P. A., Hinshaw D. B., Halsey W. A., Jr, Schraufstätter I. U., Sauerheber R. D., Spragg R. G., Jackson J. H., Cochrane C. G. Mechanisms of oxidant-mediated cell injury. The glycolytic and mitochondrial pathways of ADP phosphorylation are major intracellular targets inactivated by hydrogen peroxide. J Biol Chem. 1988 Feb 5;263(4):1665–1675. [PubMed] [Google Scholar]
- Jackson C. V., Mickelson J. K., Pope T. K., Rao P. S., Lucchesi B. R. O2 free radical-mediated myocardial and vascular dysfunction. Am J Physiol. 1986 Dec;251(6 Pt 2):H1225–H1231. doi: 10.1152/ajpheart.1986.251.6.H1225. [DOI] [PubMed] [Google Scholar]
- Jolly S. R., Kane W. J., Bailie M. B., Abrams G. D., Lucchesi B. R. Canine myocardial reperfusion injury. Its reduction by the combined administration of superoxide dismutase and catalase. Circ Res. 1984 Mar;54(3):277–285. doi: 10.1161/01.res.54.3.277. [DOI] [PubMed] [Google Scholar]
- Josephson R. A., Silverman H. S., Lakatta E. G., Stern M. D., Zweier J. L. Study of the mechanisms of hydrogen peroxide and hydroxyl free radical-induced cellular injury and calcium overload in cardiac myocytes. J Biol Chem. 1991 Feb 5;266(4):2354–2361. [PubMed] [Google Scholar]
- Kaneko M., Beamish R. E., Dhalla N. S. Depression of heart sarcolemmal Ca2+-pump activity by oxygen free radicals. Am J Physiol. 1989 Feb;256(2 Pt 2):H368–H374. doi: 10.1152/ajpheart.1989.256.2.H368. [DOI] [PubMed] [Google Scholar]
- Kim M. S., Akera T. O2 free radicals: cause of ischemia-reperfusion injury to cardiac Na+-K+-ATPase. Am J Physiol. 1987 Feb;252(2 Pt 2):H252–H257. doi: 10.1152/ajpheart.1987.252.2.H252. [DOI] [PubMed] [Google Scholar]
- Kitakaze M., Marban E. Cellular mechanism of the modulation of contractile function by coronary perfusion pressure in ferret hearts. J Physiol. 1989 Jul;414:455–472. doi: 10.1113/jphysiol.1989.sp017698. [DOI] [PMC free article] [PubMed] [Google Scholar]
- Kitakaze M., Weisman H. F., Marban E. Contractile dysfunction and ATP depletion after transient calcium overload in perfused ferret hearts. Circulation. 1988 Mar;77(3):685–695. doi: 10.1161/01.cir.77.3.685. [DOI] [PubMed] [Google Scholar]
- Koretsune Y., Marban E. Mechanism of ischemic contracture in ferret hearts: relative roles of [Ca2+]i elevation and ATP depletion. Am J Physiol. 1990 Jan;258(1 Pt 2):H9–16. doi: 10.1152/ajpheart.1990.258.1.H9. [DOI] [PubMed] [Google Scholar]
- Kuppusamy P., Zweier J. L. Characterization of free radical generation by xanthine oxidase. Evidence for hydroxyl radical generation. J Biol Chem. 1989 Jun 15;264(17):9880–9884. [PubMed] [Google Scholar]
- Kusuoka H., Jacobus W. E., Marban E. Calcium oscillations in digitalis-induced ventricular fibrillation: pathogenetic role and metabolic consequences in isolated ferret hearts. Circ Res. 1988 Mar;62(3):609–619. doi: 10.1161/01.res.62.3.609. [DOI] [PubMed] [Google Scholar]
- Kusuoka H., Weisfeldt M. L., Zweier J. L., Jacobus W. E., Marban E. Mechanism of early contractile failure during hypoxia in intact ferret heart: evidence for modulation of maximal Ca2+-activated force by inorganic phosphate. Circ Res. 1986 Sep;59(3):270–282. doi: 10.1161/01.res.59.3.270. [DOI] [PubMed] [Google Scholar]
- Lehninger A. L. Mitochondria and calcium ion transport. Biochem J. 1970 Sep;119(2):129–138. doi: 10.1042/bj1190129. [DOI] [PMC free article] [PubMed] [Google Scholar]
- Marban E., Kitakaze M., Koretsune Y., Yue D. T., Chacko V. P., Pike M. M. Quantification of [Ca2+]i in perfused hearts. Critical evaluation of the 5F-BAPTA and nuclear magnetic resonance method as applied to the study of ischemia and reperfusion. Circ Res. 1990 May;66(5):1255–1267. doi: 10.1161/01.res.66.5.1255. [DOI] [PubMed] [Google Scholar]
- Marban E., Kitakaze M., Kusuoka H., Porterfield J. K., Yue D. T., Chacko V. P. Intracellular free calcium concentration measured with 19F NMR spectroscopy in intact ferret hearts. Proc Natl Acad Sci U S A. 1987 Aug;84(16):6005–6009. doi: 10.1073/pnas.84.16.6005. [DOI] [PMC free article] [PubMed] [Google Scholar]
- Matsuura H., Shattock M. J. Membrane potential fluctuations and transient inward currents induced by reactive oxygen intermediates in isolated rabbit ventricular cells. Circ Res. 1991 Feb;68(2):319–329. doi: 10.1161/01.res.68.2.319. [DOI] [PubMed] [Google Scholar]
- McCord J. M. Oxygen-derived free radicals in postischemic tissue injury. N Engl J Med. 1985 Jan 17;312(3):159–163. doi: 10.1056/NEJM198501173120305. [DOI] [PubMed] [Google Scholar]
- Navon G., Navon R., Shulman R. G., Yamane T. Phosphate metabolites in lymphoid, Friend erythroleukemia, and HeLa cells observed by high-resolution 31P nuclear magnetic resonance. Proc Natl Acad Sci U S A. 1978 Feb;75(2):891–895. doi: 10.1073/pnas.75.2.891. [DOI] [PMC free article] [PubMed] [Google Scholar]
- Okabe E., Fujimaki R., Murayama M., Ito H. Possible mechanism responsible for mechanical dysfunction of ischemic myocardium: a role of oxygen free radicals. Jpn Circ J. 1989 Sep;53(9):1132–1137. doi: 10.1253/jcj.53.1132. [DOI] [PubMed] [Google Scholar]
- Owen P., Dennis S., Opie L. H. Glucose flux rate regulates onset of ischemic contracture in globally underperfused rat hearts. Circ Res. 1990 Feb;66(2):344–354. doi: 10.1161/01.res.66.2.344. [DOI] [PubMed] [Google Scholar]
- Proietti E., Carpinelli G., Di Vito M., Belardelli F., Gresser I., Podo F. 31P-nuclear magnetic resonance analysis of interferon-induced alterations of phospholipid metabolites in interferon-sensitive and interferon-resistant Friend leukemia cell tumors in mice. Cancer Res. 1986 Jun;46(6):2849–2857. [PubMed] [Google Scholar]
- Reimer K. A., Jennings R. B. Failure of the xanthine oxidase inhibitor allopurinol to limit infarct size after ischemia and reperfusion in dogs. Circulation. 1985 May;71(5):1069–1075. doi: 10.1161/01.cir.71.5.1069. [DOI] [PubMed] [Google Scholar]
- Renlund D. G., Lakatta E. G., Mellits E. D., Gerstenblith G. Calcium-dependent enhancement of myocardial diastolic tone and energy utilization dissociates systolic work and oxygen consumption during low sodium perfusion. Circ Res. 1985 Dec;57(6):876–888. doi: 10.1161/01.res.57.6.876. [DOI] [PubMed] [Google Scholar]
- Rowe G. T., Manson N. H., Caplan M., Hess M. L. Hydrogen peroxide and hydroxyl radical mediation of activated leukocyte depression of cardiac sarcoplasmic reticulum. Participation of the cyclooxygenase pathway. Circ Res. 1983 Nov;53(5):584–591. doi: 10.1161/01.res.53.5.584. [DOI] [PubMed] [Google Scholar]
- Scharfman H. E., Schwartzkroin P. A. Protection of dentate hilar cells from prolonged stimulation by intracellular calcium chelation. Science. 1989 Oct 13;246(4927):257–260. doi: 10.1126/science.2508225. [DOI] [PubMed] [Google Scholar]
- Shine K. I., Douglas A. M. Low calcium reperfusion of ischemic myocardium. J Mol Cell Cardiol. 1983 Apr;15(4):251–260. doi: 10.1016/0022-2828(83)90280-8. [DOI] [PubMed] [Google Scholar]
- Smith G. A., Hesketh R. T., Metcalfe J. C., Feeney J., Morris P. G. Intracellular calcium measurements by 19F NMR of fluorine-labeled chelators. Proc Natl Acad Sci U S A. 1983 Dec;80(23):7178–7182. doi: 10.1073/pnas.80.23.7178. [DOI] [PMC free article] [PubMed] [Google Scholar]
- Steenbergen C., Murphy E., Levy L., London R. E. Elevation in cytosolic free calcium concentration early in myocardial ischemia in perfused rat heart. Circ Res. 1987 May;60(5):700–707. doi: 10.1161/01.res.60.5.700. [DOI] [PubMed] [Google Scholar]
- Tarr M. T., Valenzeno D. P. Modification of cardiac action potential by photosensitizer-generated reactive oxygen. J Mol Cell Cardiol. 1989 Jun;21(6):539–543. doi: 10.1016/0022-2828(89)90819-5. [DOI] [PubMed] [Google Scholar]
- Thompson-Gorman S. L., Zweier J. L. Evaluation of the role of xanthine oxidase in myocardial reperfusion injury. J Biol Chem. 1990 Apr 25;265(12):6656–6663. [PubMed] [Google Scholar]
- Thompson J. A., Hess M. L. The oxygen free radical system: a fundamental mechanism in the production of myocardial necrosis. Prog Cardiovasc Dis. 1986 May-Jun;28(6):449–462. doi: 10.1016/0033-0620(86)90027-7. [DOI] [PubMed] [Google Scholar]
- Uraizee A., Reimer K. A., Murry C. E., Jennings R. B. Failure of superoxide dismutase to limit size of myocardial infarction after 40 minutes of ischemia and 4 days of reperfusion in dogs. Circulation. 1987 Jun;75(6):1237–1248. doi: 10.1161/01.cir.75.6.1237. [DOI] [PubMed] [Google Scholar]
- Weiss J. N., Lamp S. T. Cardiac ATP-sensitive K+ channels. Evidence for preferential regulation by glycolysis. J Gen Physiol. 1989 Nov;94(5):911–935. doi: 10.1085/jgp.94.5.911. [DOI] [PMC free article] [PubMed] [Google Scholar]
- Weiss J., Hiltbrand B. Functional compartmentation of glycolytic versus oxidative metabolism in isolated rabbit heart. J Clin Invest. 1985 Feb;75(2):436–447. doi: 10.1172/JCI111718. [DOI] [PMC free article] [PubMed] [Google Scholar]
- Wier W. G., Hess P. Excitation-contraction coupling in cardiac Purkinje fibers. Effects of cardiotonic steroids on the intracellular [Ca2+] transient, membrane potential, and contraction. J Gen Physiol. 1984 Mar;83(3):395–415. doi: 10.1085/jgp.83.3.395. [DOI] [PMC free article] [PubMed] [Google Scholar]
- Yue D. T., Marban E., Wier W. G. Relationship between force and intracellular [Ca2+] in tetanized mammalian heart muscle. J Gen Physiol. 1986 Feb;87(2):223–242. doi: 10.1085/jgp.87.2.223. [DOI] [PMC free article] [PubMed] [Google Scholar]
- Zweier J. L., Flaherty J. T., Weisfeldt M. L. Direct measurement of free radical generation following reperfusion of ischemic myocardium. Proc Natl Acad Sci U S A. 1987 Mar;84(5):1404–1407. doi: 10.1073/pnas.84.5.1404. [DOI] [PMC free article] [PubMed] [Google Scholar]
- Zweier J. L., Kuppusamy P., Williams R., Rayburn B. K., Smith D., Weisfeldt M. L., Flaherty J. T. Measurement and characterization of postischemic free radical generation in the isolated perfused heart. J Biol Chem. 1989 Nov 15;264(32):18890–18895. [PubMed] [Google Scholar]
- Zweier J. L. Measurement of superoxide-derived free radicals in the reperfused heart. Evidence for a free radical mechanism of reperfusion injury. J Biol Chem. 1988 Jan 25;263(3):1353–1357. [PubMed] [Google Scholar]