Abstract
We tested the hypothesis that glycolytic inhibition by 2-deoxyglucose causes greater impairment of diastolic relaxation and intracellular calcium handling in well-oxygenated hypertrophied adult rat myocytes compared with control myocytes. We simultaneously measured cell motion and intracellular free calcium concentration ([Ca2+]i) with indo-1 in isolated paced myocytes from aortic-banded rats and sham-operated rats. There was no difference in either the end-diastolic or peak-systolic [Ca2+]i between control and hypertrophied myocytes (97 +/- 18 vs. 105 +/- 15 nM, 467 +/- 92 vs. 556 +/- 67 nM, respectively). Myocytes were first superfused with oxygenated Hepes-buffered solution containing 1.2 mM CaCl2, 5.6 mM glucose, and 5 mM acetate, and paced at 3 Hz at 36 degrees C. Exposure to 20 mM 2-deoxyglucose as substitution of glucose for 15 min caused an upward shift of end-diastolic cell position in both control (n = 5) and hypertrophied myocytes (n = 10) (P < 0.001 vs. baseline), indicating an impaired extent of relaxation. Hypertrophied myocytes, however, showed a greater upward shift in end-diastolic cell position and slowing of relaxation compared with control myocytes (delta 144 +/- 28 vs. 55 +/- 15% of baseline diastolic position, P < 0.02). Exposure to 2-deoxyglucose increased end-diastolic [Ca2+]i in both groups (P < 0.001 vs. baseline), but there was no difference between hypertrophied and control myocytes (218 +/- 38 vs. 183 +/- 29 nM, respectively). The effects of 2-deoxyglucose were corroborated in isolated oxygenated perfused hearts in which glycolytic inhibition which caused severe elevation of isovolumic diastolic pressure and prolongation of relaxation in the hypertrophied hearts compared with controls. In summary, the inhibition of the glycolytic pathway impairs diastolic relaxation to a greater extent in hypertrophied myocytes than in control myocytes even in well-oxygenated conditions. The severe impairment of diastolic relaxation induced by 2-deoxyglucose in hypertrophied myocytes compared with control myocytes cannot be explained by greater diastolic Ca2+ overload, which implicates an increase in myofilament Ca(2+)-responsiveness as a possible mechanism.
Full text
PDF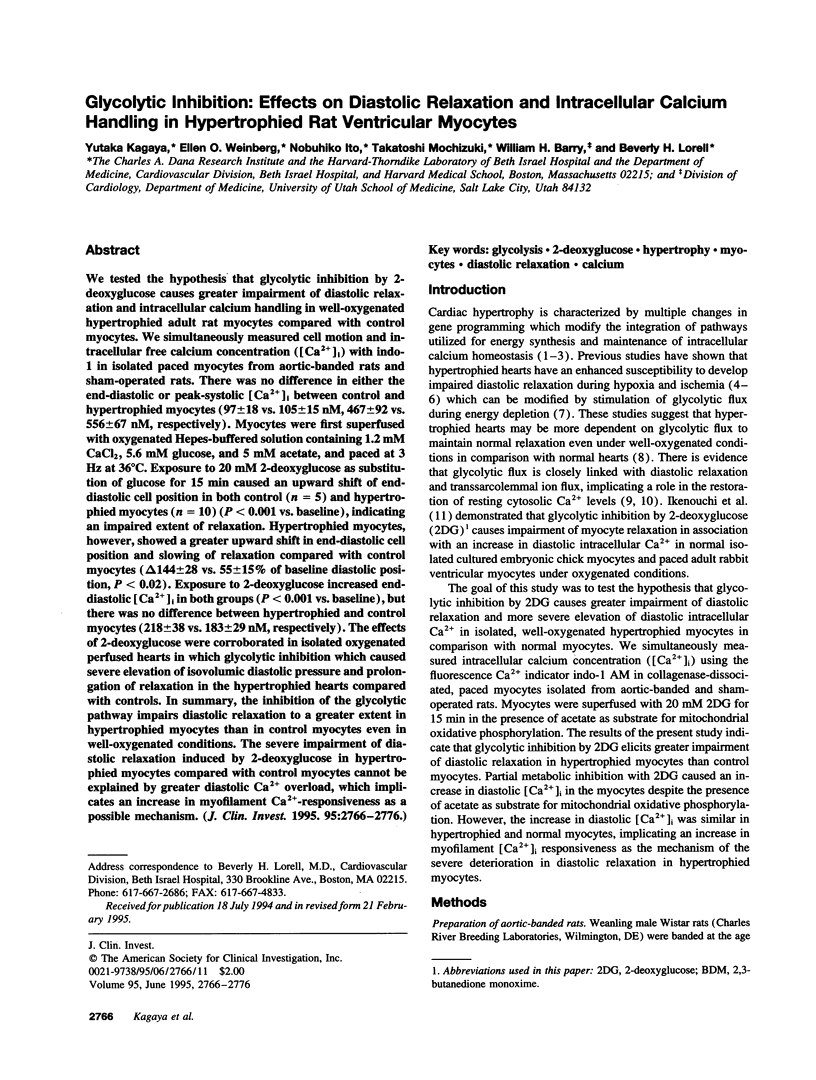
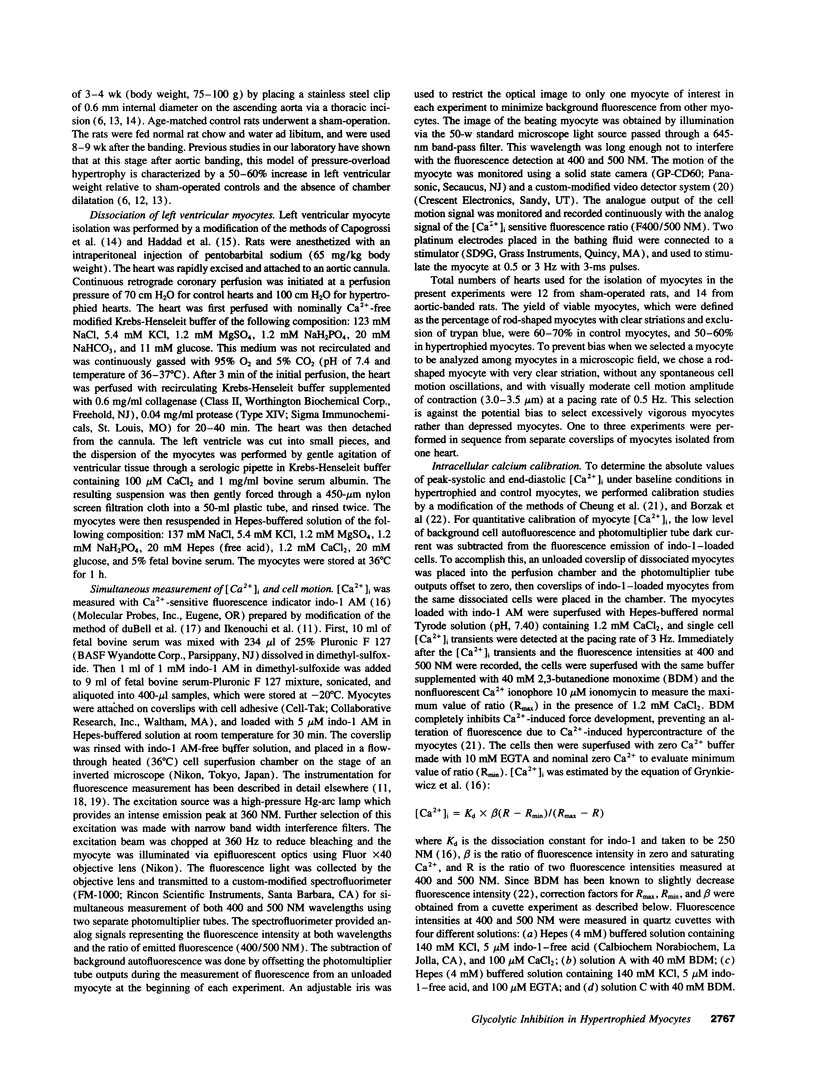
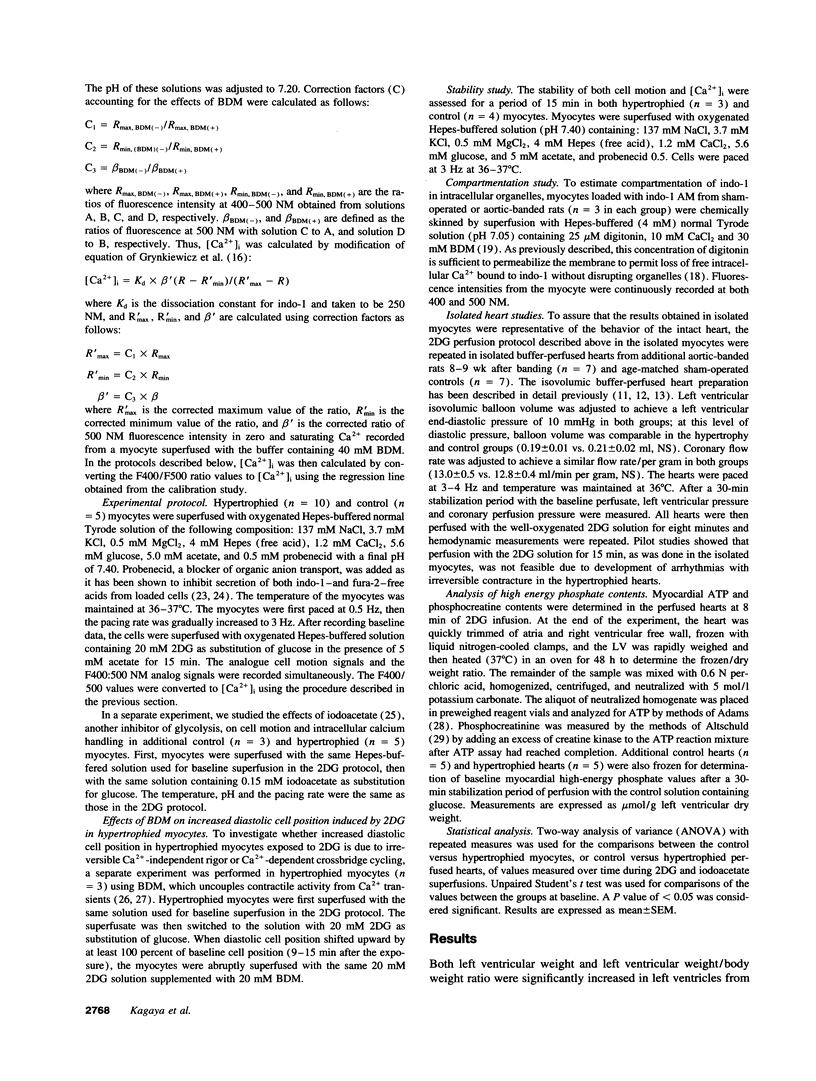
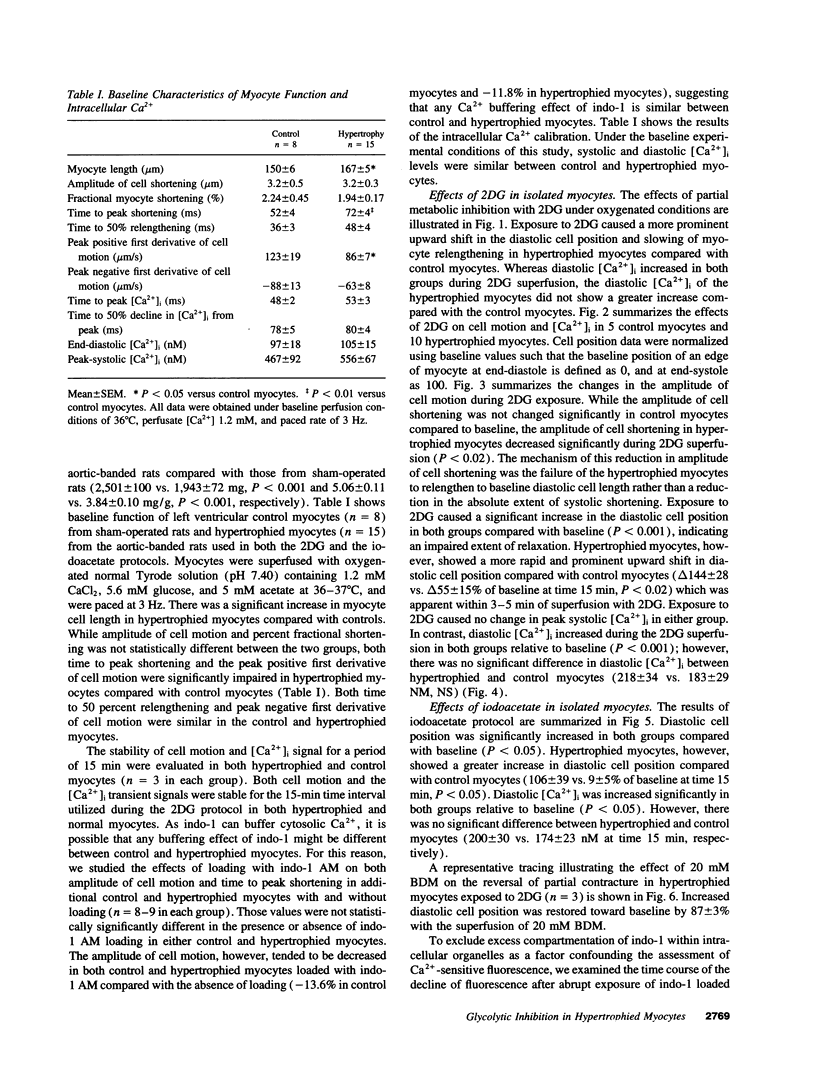
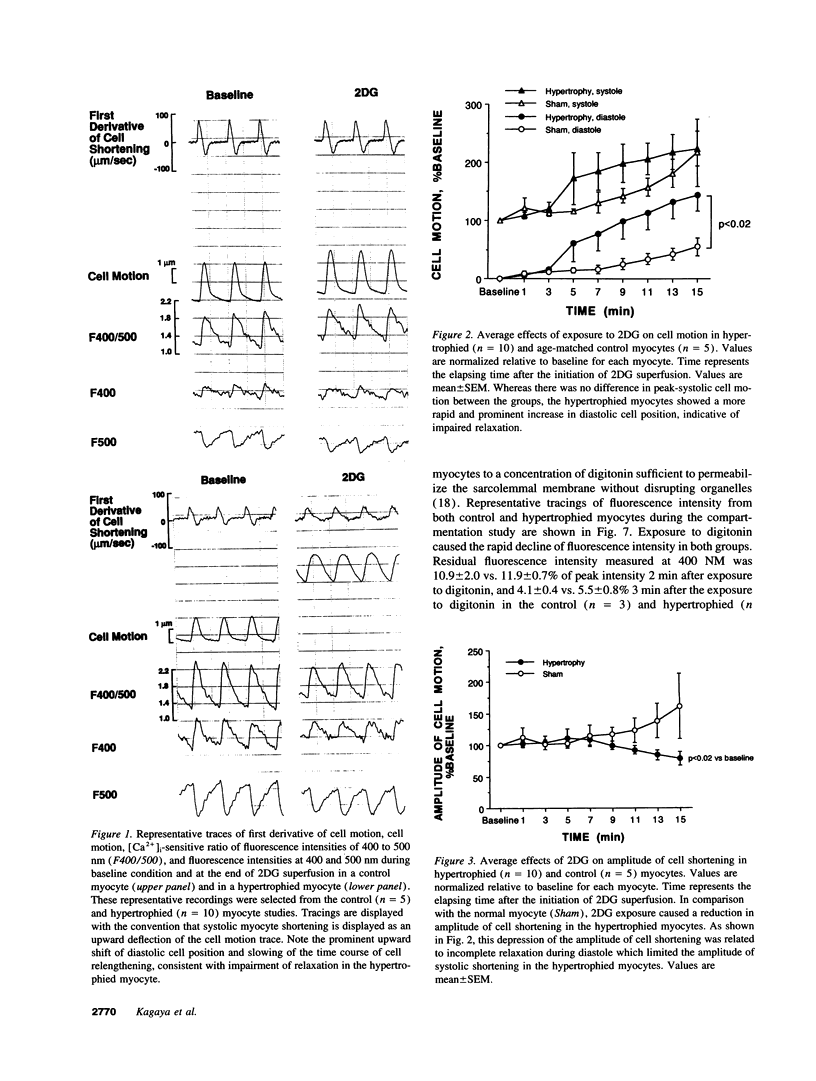
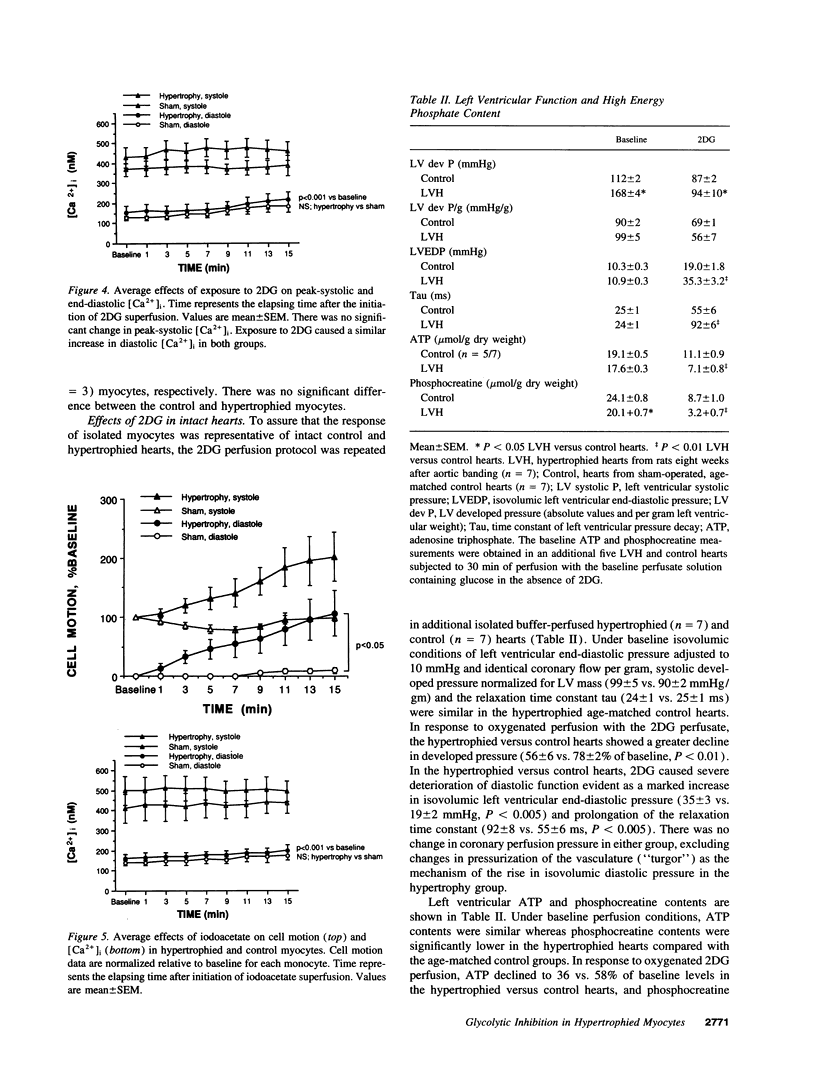
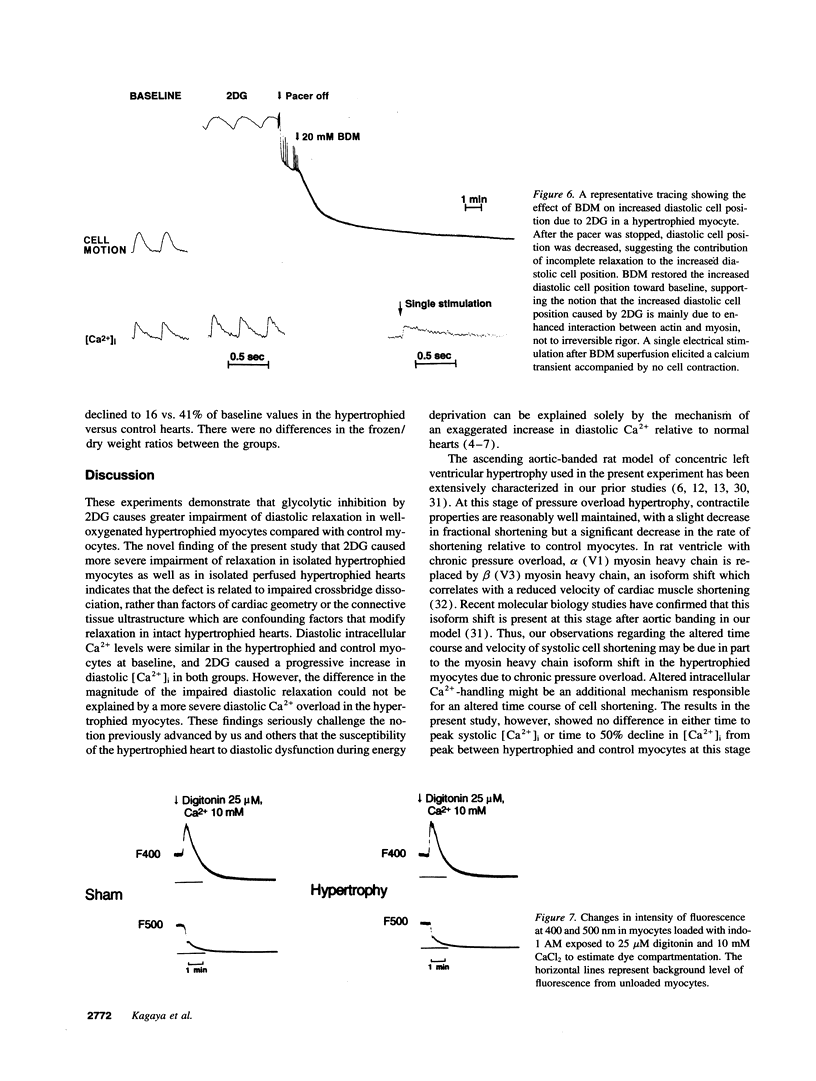
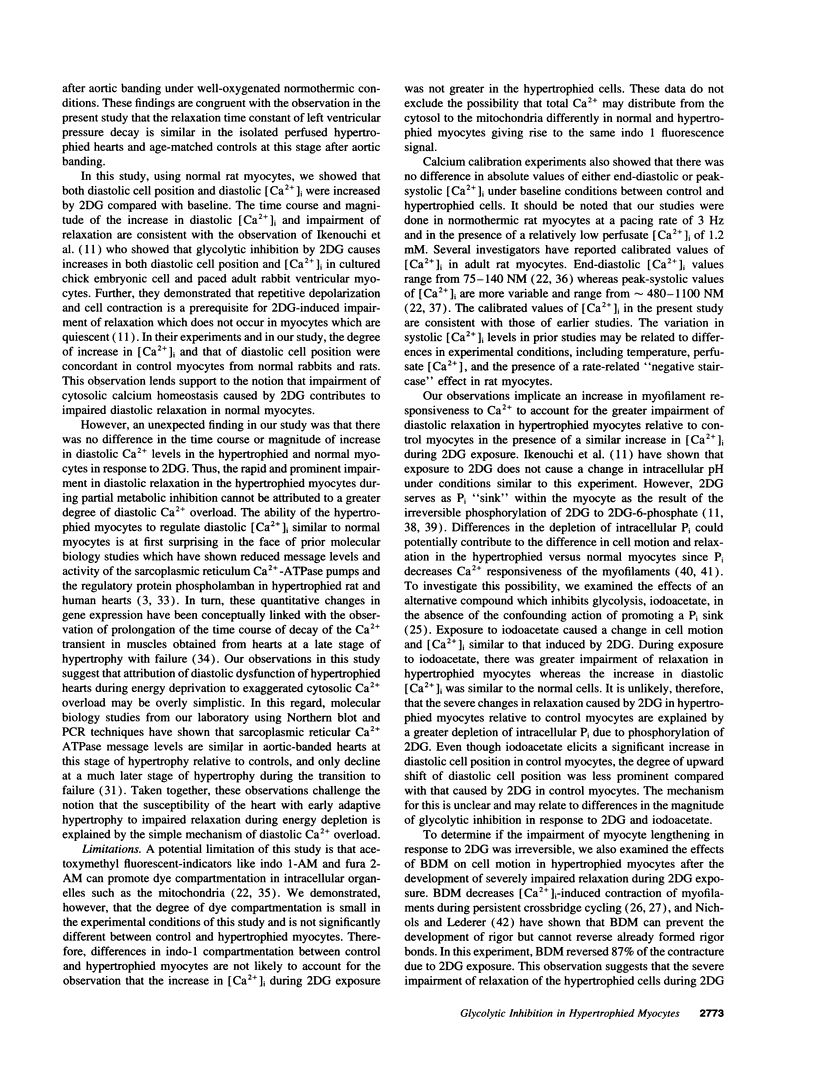
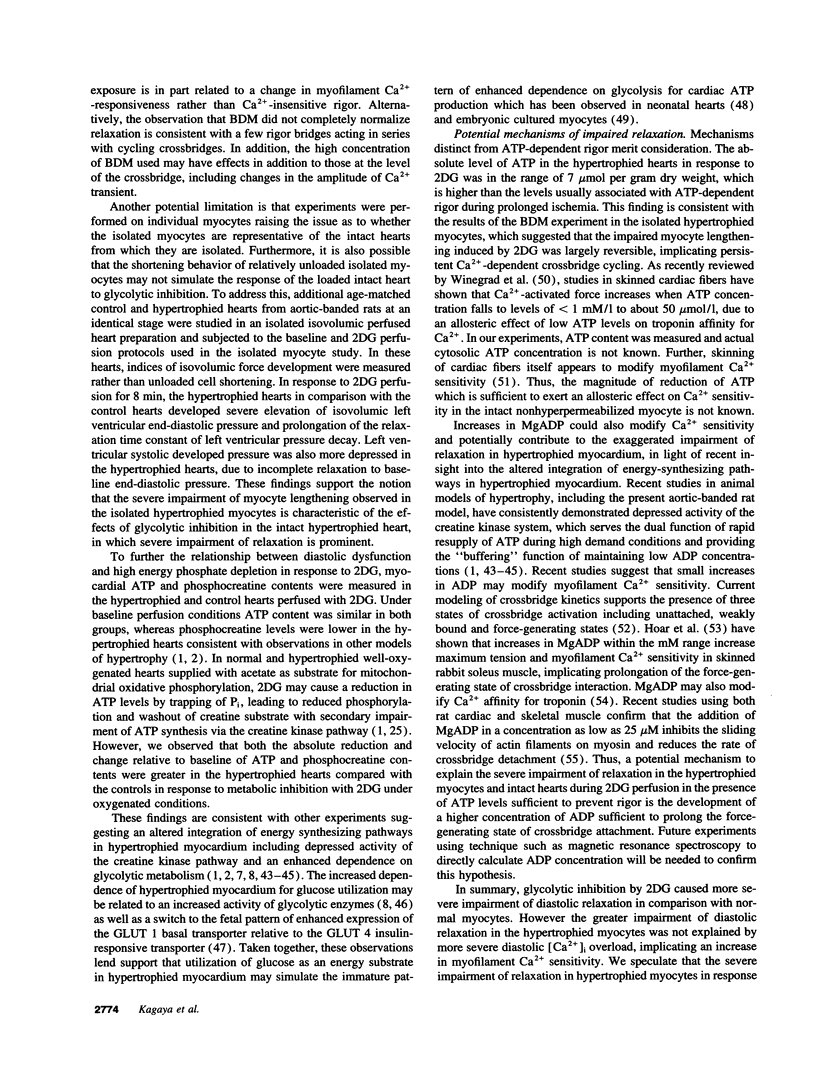
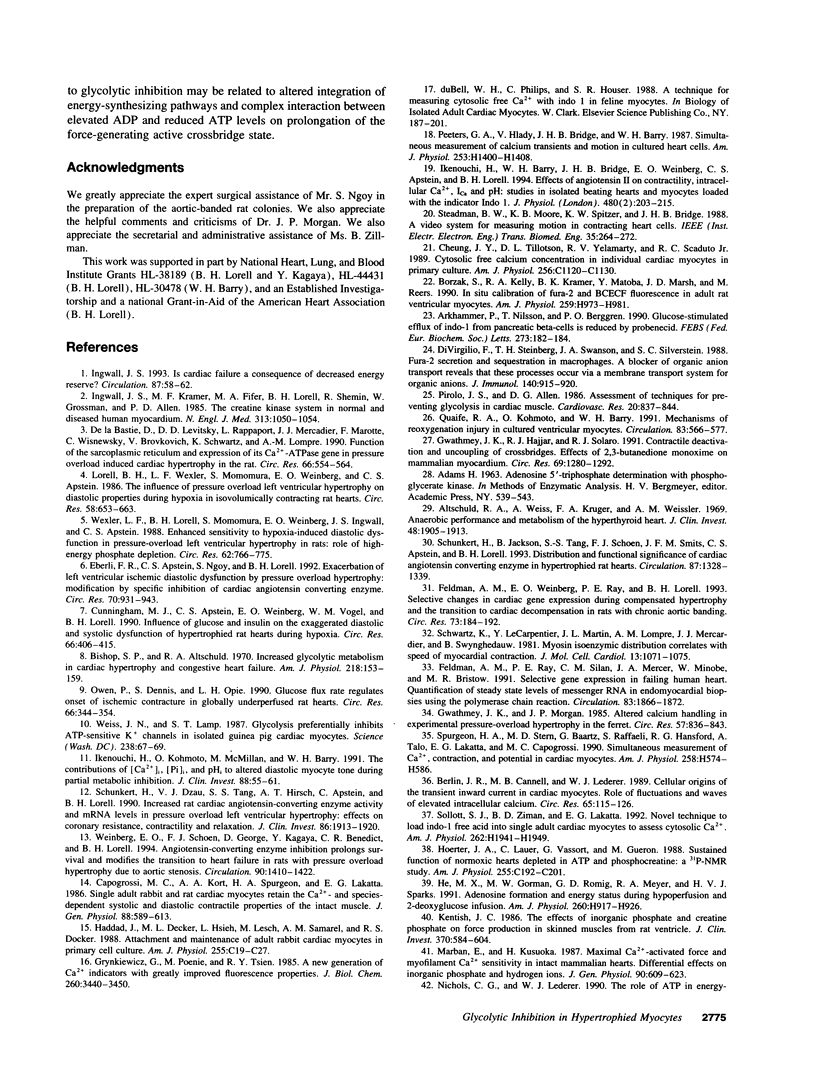
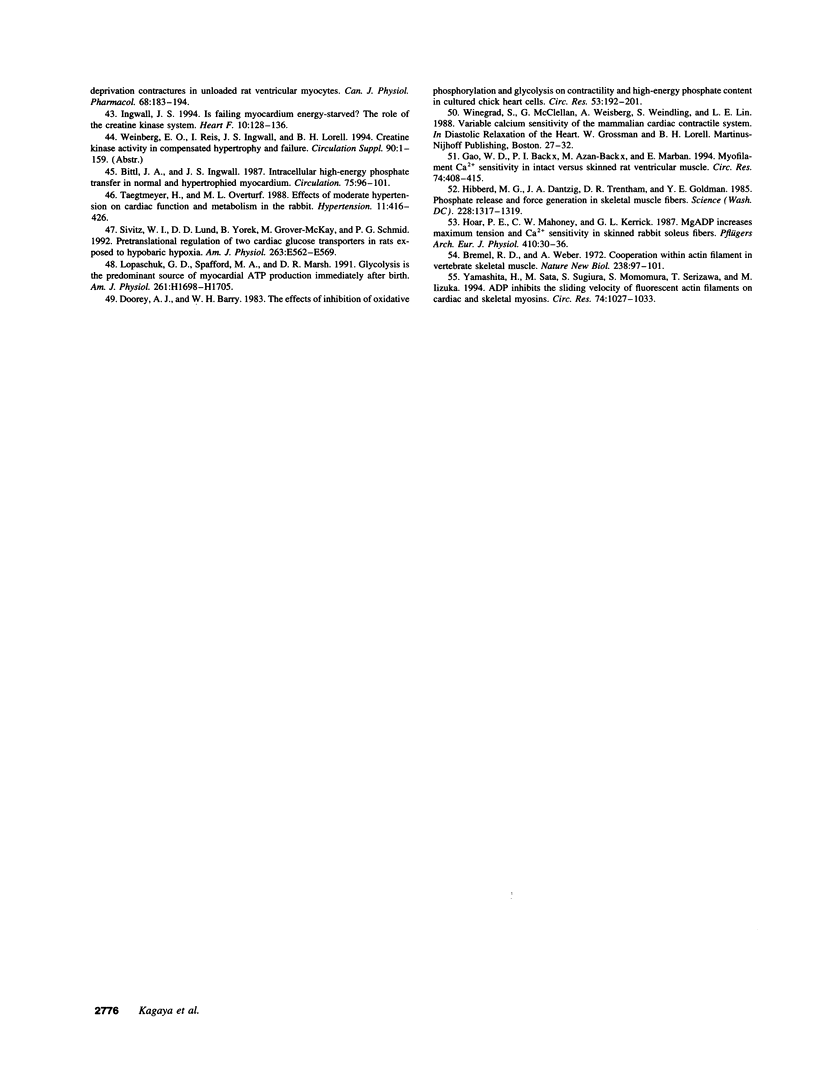
Selected References
These references are in PubMed. This may not be the complete list of references from this article.
- Altschuld R. A., Weiss A., Kruger F. A., Weissler A. M. Anaerobic performance and metabolism of the hyperthyroid heart. J Clin Invest. 1969 Oct;48(10):1905–1913. doi: 10.1172/JCI106157. [DOI] [PMC free article] [PubMed] [Google Scholar]
- Arkhammar P., Nilsson T., Berggren P. O. Glucose-stimulated efflux of indo-1 from pancreatic beta-cells is reduced by probenecid. FEBS Lett. 1990 Oct 29;273(1-2):182–184. doi: 10.1016/0014-5793(90)81079-4. [DOI] [PubMed] [Google Scholar]
- Berlin J. R., Cannell M. B., Lederer W. J. Cellular origins of the transient inward current in cardiac myocytes. Role of fluctuations and waves of elevated intracellular calcium. Circ Res. 1989 Jul;65(1):115–126. doi: 10.1161/01.res.65.1.115. [DOI] [PubMed] [Google Scholar]
- Bishop S. P., Altschuld R. A. Increased glycolytic metabolism in cardiac hypertrophy and congestive failure. Am J Physiol. 1970 Jan;218(1):153–159. doi: 10.1152/ajplegacy.1970.218.1.153. [DOI] [PubMed] [Google Scholar]
- Bittl J. A., Ingwall J. S. Intracellular high-energy phosphate transfer in normal and hypertrophied myocardium. Circulation. 1987 Jan;75(1 Pt 2):I96–101. [PubMed] [Google Scholar]
- Borzak S., Kelly R. A., Krämer B. K., Matoba Y., Marsh J. D., Reers M. In situ calibration of fura-2 and BCECF fluorescence in adult rat ventricular myocytes. Am J Physiol. 1990 Sep;259(3 Pt 2):H973–H981. doi: 10.1152/ajpheart.1990.259.3.H973. [DOI] [PubMed] [Google Scholar]
- Bremel R. D., Weber A. Cooperation within actin filament in vertebrate skeletal muscle. Nat New Biol. 1972 Jul 26;238(82):97–101. doi: 10.1038/newbio238097a0. [DOI] [PubMed] [Google Scholar]
- Capogrossi M. C., Kort A. A., Spurgeon H. A., Lakatta E. G. Single adult rabbit and rat cardiac myocytes retain the Ca2+- and species-dependent systolic and diastolic contractile properties of intact muscle. J Gen Physiol. 1986 Nov;88(5):589–613. doi: 10.1085/jgp.88.5.589. [DOI] [PMC free article] [PubMed] [Google Scholar]
- Cunningham M. J., Apstein C. S., Weinberg E. O., Vogel W. M., Lorell B. H. Influence of glucose and insulin on the exaggerated diastolic and systolic dysfunction of hypertrophied rat hearts during hypoxia. Circ Res. 1990 Feb;66(2):406–415. doi: 10.1161/01.res.66.2.406. [DOI] [PubMed] [Google Scholar]
- Di Virgilio F., Steinberg T. H., Swanson J. A., Silverstein S. C. Fura-2 secretion and sequestration in macrophages. A blocker of organic anion transport reveals that these processes occur via a membrane transport system for organic anions. J Immunol. 1988 Feb 1;140(3):915–920. [PubMed] [Google Scholar]
- Doorey A. J., Barry W. H. The effects of inhibition of oxidative phosphorylation and glycolysis on contractility and high-energy phosphate content in cultured chick heart cells. Circ Res. 1983 Aug;53(2):192–201. doi: 10.1161/01.res.53.2.192. [DOI] [PubMed] [Google Scholar]
- Eberli F. R., Apstein C. S., Ngoy S., Lorell B. H. Exacerbation of left ventricular ischemic diastolic dysfunction by pressure-overload hypertrophy. Modification by specific inhibition of cardiac angiotensin converting enzyme. Circ Res. 1992 May;70(5):931–943. doi: 10.1161/01.res.70.5.931. [DOI] [PubMed] [Google Scholar]
- Feldman A. M., Ray P. E., Silan C. M., Mercer J. A., Minobe W., Bristow M. R. Selective gene expression in failing human heart. Quantification of steady-state levels of messenger RNA in endomyocardial biopsies using the polymerase chain reaction. Circulation. 1991 Jun;83(6):1866–1872. doi: 10.1161/01.cir.83.6.1866. [DOI] [PubMed] [Google Scholar]
- Feldman A. M., Weinberg E. O., Ray P. E., Lorell B. H. Selective changes in cardiac gene expression during compensated hypertrophy and the transition to cardiac decompensation in rats with chronic aortic banding. Circ Res. 1993 Jul;73(1):184–192. doi: 10.1161/01.res.73.1.184. [DOI] [PubMed] [Google Scholar]
- Gao W. D., Backx P. H., Azan-Backx M., Marban E. Myofilament Ca2+ sensitivity in intact versus skinned rat ventricular muscle. Circ Res. 1994 Mar;74(3):408–415. doi: 10.1161/01.res.74.3.408. [DOI] [PubMed] [Google Scholar]
- Grynkiewicz G., Poenie M., Tsien R. Y. A new generation of Ca2+ indicators with greatly improved fluorescence properties. J Biol Chem. 1985 Mar 25;260(6):3440–3450. [PubMed] [Google Scholar]
- Gwathmey J. K., Hajjar R. J., Solaro R. J. Contractile deactivation and uncoupling of crossbridges. Effects of 2,3-butanedione monoxime on mammalian myocardium. Circ Res. 1991 Nov;69(5):1280–1292. doi: 10.1161/01.res.69.5.1280. [DOI] [PubMed] [Google Scholar]
- Gwathmey J. K., Morgan J. P. Altered calcium handling in experimental pressure-overload hypertrophy in the ferret. Circ Res. 1985 Dec;57(6):836–843. doi: 10.1161/01.res.57.6.836. [DOI] [PubMed] [Google Scholar]
- Haddad J., Decker M. L., Hsieh L. C., Lesch M., Samarel A. M., Decker R. S. Attachment and maintenance of adult rabbit cardiac myocytes in primary cell culture. Am J Physiol. 1988 Jul;255(1 Pt 1):C19–C27. doi: 10.1152/ajpcell.1988.255.1.C19. [DOI] [PubMed] [Google Scholar]
- He M. X., Gorman M. W., Romig G. D., Meyer R. A., Sparks H. V., Jr Adenosine formation and energy status during hypoperfusion and 2-deoxyglucose infusion. Am J Physiol. 1991 Mar;260(3 Pt 2):H917–H926. doi: 10.1152/ajpheart.1991.260.3.H917. [DOI] [PubMed] [Google Scholar]
- Hibberd M. G., Dantzig J. A., Trentham D. R., Goldman Y. E. Phosphate release and force generation in skeletal muscle fibers. Science. 1985 Jun 14;228(4705):1317–1319. doi: 10.1126/science.3159090. [DOI] [PubMed] [Google Scholar]
- Hoar P. E., Mahoney C. W., Kerrick W. G. MgADP- increases maximum tension and Ca2+ sensitivity in skinned rabbit soleus fibers. Pflugers Arch. 1987 Sep;410(1-2):30–36. doi: 10.1007/BF00581892. [DOI] [PubMed] [Google Scholar]
- Hoerter J. A., Lauer C., Vassort G., Guéron M. Sustained function of normoxic hearts depleted in ATP and phosphocreatine: a 31P-NMR study. Am J Physiol. 1988 Aug;255(2 Pt 1):C192–C201. doi: 10.1152/ajpcell.1988.255.2.C192. [DOI] [PubMed] [Google Scholar]
- Ikenouchi H., Barry W. H., Bridge J. H., Weinberg E. O., Apstein C. S., Lorell B. H. Effects of angiotensin II on intracellular Ca2+ and pH in isolated beating rabbit hearts and myocytes loaded with the indicator indo-1. J Physiol. 1994 Oct 15;480(Pt 2):203–215. doi: 10.1113/jphysiol.1994.sp020353. [DOI] [PMC free article] [PubMed] [Google Scholar]
- Ikenouchi H., Kohmoto O., McMillan M., Barry W. H. Contributions of [Ca2+]i, [Pi]i, and pHi to altered diastolic myocyte tone during partial metabolic inhibition. J Clin Invest. 1991 Jul;88(1):55–61. doi: 10.1172/JCI115304. [DOI] [PMC free article] [PubMed] [Google Scholar]
- Ingwall J. S., Kramer M. F., Fifer M. A., Lorell B. H., Shemin R., Grossman W., Allen P. D. The creatine kinase system in normal and diseased human myocardium. N Engl J Med. 1985 Oct 24;313(17):1050–1054. doi: 10.1056/NEJM198510243131704. [DOI] [PubMed] [Google Scholar]
- Kentish J. C. The effects of inorganic phosphate and creatine phosphate on force production in skinned muscles from rat ventricle. J Physiol. 1986 Jan;370:585–604. doi: 10.1113/jphysiol.1986.sp015952. [DOI] [PMC free article] [PubMed] [Google Scholar]
- Lopaschuk G. D., Spafford M. A., Marsh D. R. Glycolysis is predominant source of myocardial ATP production immediately after birth. Am J Physiol. 1991 Dec;261(6 Pt 2):H1698–H1705. doi: 10.1152/ajpheart.1991.261.6.H1698. [DOI] [PubMed] [Google Scholar]
- Lorell B. H., Wexler L. F., Momomura S., Weinberg E., Apstein C. S. The influence of pressure overload left ventricular hypertrophy on diastolic properties during hypoxia in isovolumically contracting rat hearts. Circ Res. 1986 May;58(5):653–663. doi: 10.1161/01.res.58.5.653. [DOI] [PubMed] [Google Scholar]
- Marban E., Kusuoka H. Maximal Ca2+-activated force and myofilament Ca2+ sensitivity in intact mammalian hearts. Differential effects of inorganic phosphate and hydrogen ions. J Gen Physiol. 1987 Nov;90(5):609–623. doi: 10.1085/jgp.90.5.609. [DOI] [PMC free article] [PubMed] [Google Scholar]
- Nichols C. G., Lederer W. J. The role of ATP in energy-deprivation contractures in unloaded rat ventricular myocytes. Can J Physiol Pharmacol. 1990 Feb;68(2):183–194. doi: 10.1139/y90-029. [DOI] [PubMed] [Google Scholar]
- Owen P., Dennis S., Opie L. H. Glucose flux rate regulates onset of ischemic contracture in globally underperfused rat hearts. Circ Res. 1990 Feb;66(2):344–354. doi: 10.1161/01.res.66.2.344. [DOI] [PubMed] [Google Scholar]
- Peeters G. A., Hlady V., Bridge J. H., Barry W. H. Simultaneous measurement of calcium transients and motion in cultured heart cells. Am J Physiol. 1987 Dec;253(6 Pt 2):H1400–H1408. doi: 10.1152/ajpheart.1987.253.6.H1400. [DOI] [PubMed] [Google Scholar]
- Pirolo J. S., Allen D. G. Assessment of techniques for preventing glycolysis in cardiac muscle. Cardiovasc Res. 1986 Nov;20(11):837–844. doi: 10.1093/cvr/20.11.837. [DOI] [PubMed] [Google Scholar]
- Quaife R. A., Kohmoto O., Barry W. H. Mechanisms of reoxygenation injury in cultured ventricular myocytes. Circulation. 1991 Feb;83(2):566–577. doi: 10.1161/01.cir.83.2.566. [DOI] [PubMed] [Google Scholar]
- Schunkert H., Dzau V. J., Tang S. S., Hirsch A. T., Apstein C. S., Lorell B. H. Increased rat cardiac angiotensin converting enzyme activity and mRNA expression in pressure overload left ventricular hypertrophy. Effects on coronary resistance, contractility, and relaxation. J Clin Invest. 1990 Dec;86(6):1913–1920. doi: 10.1172/JCI114924. [DOI] [PMC free article] [PubMed] [Google Scholar]
- Schunkert H., Jackson B., Tang S. S., Schoen F. J., Smits J. F., Apstein C. S., Lorell B. H. Distribution and functional significance of cardiac angiotensin converting enzyme in hypertrophied rat hearts. Circulation. 1993 Apr;87(4):1328–1339. doi: 10.1161/01.cir.87.4.1328. [DOI] [PubMed] [Google Scholar]
- Schwartz K., Lecarpentier Y., Martin J. L., Lompré A. M., Mercadier J. J., Swynghedauw B. Myosin isoenzymic distribution correlates with speed of myocardial contraction. J Mol Cell Cardiol. 1981 Dec;13(12):1071–1075. doi: 10.1016/0022-2828(81)90297-2. [DOI] [PubMed] [Google Scholar]
- Sivitz W. I., Lund D. D., Yorek B., Grover-McKay M., Schmid P. G. Pretranslational regulation of two cardiac glucose transporters in rats exposed to hypobaric hypoxia. Am J Physiol. 1992 Sep;263(3 Pt 1):E562–E569. doi: 10.1152/ajpendo.1992.263.3.E562. [DOI] [PubMed] [Google Scholar]
- Sollott S. J., Ziman B. D., Lakatta E. G. Novel technique to load indo-1 free acid into single adult cardiac myocytes to assess cytosolic Ca2+. Am J Physiol. 1992 Jun;262(6 Pt 2):H1941–H1949. doi: 10.1152/ajpheart.1992.262.6.H1941. [DOI] [PubMed] [Google Scholar]
- Spurgeon H. A., Stern M. D., Baartz G., Raffaeli S., Hansford R. G., Talo A., Lakatta E. G., Capogrossi M. C. Simultaneous measurement of Ca2+, contraction, and potential in cardiac myocytes. Am J Physiol. 1990 Feb;258(2 Pt 2):H574–H586. doi: 10.1152/ajpheart.1990.258.2.H574. [DOI] [PubMed] [Google Scholar]
- Steadman B. W., Moore K. B., Spitzer K. W., Bridge J. H. A video system for measuring motion in contracting heart cells. IEEE Trans Biomed Eng. 1988 Apr;35(4):264–272. doi: 10.1109/10.1375. [DOI] [PubMed] [Google Scholar]
- Taegtmeyer H., Overturf M. L. Effects of moderate hypertension on cardiac function and metabolism in the rabbit. Hypertension. 1988 May;11(5):416–426. doi: 10.1161/01.hyp.11.5.416. [DOI] [PubMed] [Google Scholar]
- Weinberg E. O., Schoen F. J., George D., Kagaya Y., Douglas P. S., Litwin S. E., Schunkert H., Benedict C. R., Lorell B. H. Angiotensin-converting enzyme inhibition prolongs survival and modifies the transition to heart failure in rats with pressure overload hypertrophy due to ascending aortic stenosis. Circulation. 1994 Sep;90(3):1410–1422. doi: 10.1161/01.cir.90.3.1410. [DOI] [PubMed] [Google Scholar]
- Weiss J. N., Lamp S. T. Glycolysis preferentially inhibits ATP-sensitive K+ channels in isolated guinea pig cardiac myocytes. Science. 1987 Oct 2;238(4823):67–69. doi: 10.1126/science.2443972. [DOI] [PubMed] [Google Scholar]
- Wexler L. F., Lorell B. H., Momomura S., Weinberg E. O., Ingwall J. S., Apstein C. S. Enhanced sensitivity to hypoxia-induced diastolic dysfunction in pressure-overload left ventricular hypertrophy in the rat: role of high-energy phosphate depletion. Circ Res. 1988 Apr;62(4):766–775. doi: 10.1161/01.res.62.4.766. [DOI] [PubMed] [Google Scholar]
- Yamashita H., Sata M., Sugiura S., Momomura S., Serizawa T., Iizuka M. ADP inhibits the sliding velocity of fluorescent actin filaments on cardiac and skeletal myosins. Circ Res. 1994 Jun;74(6):1027–1033. doi: 10.1161/01.res.74.6.1027. [DOI] [PubMed] [Google Scholar]
- de la Bastie D., Levitsky D., Rappaport L., Mercadier J. J., Marotte F., Wisnewsky C., Brovkovich V., Schwartz K., Lompré A. M. Function of the sarcoplasmic reticulum and expression of its Ca2(+)-ATPase gene in pressure overload-induced cardiac hypertrophy in the rat. Circ Res. 1990 Feb;66(2):554–564. doi: 10.1161/01.res.66.2.554. [DOI] [PubMed] [Google Scholar]