Abstract
Increased tonic contractile activity from exercise or electrical stimulation induces a variety of changes in skeletal muscle, including vascular growth, myoblast proliferation, and fast to slow fiber type conversion. Little is known about the cellular control of such changes, but pleiotropic biochemical modulators such as fibroblast growth factors (FGFs) may be involved in this response and thus may be regulated in response to such stimuli. We examined the regulation of FGF expression in an in vivo model of exercise conditioning previously shown to exhibit vascular growth and fast to slow fiber conversion. FGFs were extracted by heparin-affinity chromatography from extensor digitorum longus muscles of adult rabbits subjected to chronic motor nerve stimulation at 10 Hz. Growth factor activity (expressed in growth factor units [GFUs]) of muscle stimulated for 3 and 21 d was assayed by [3H]thymidine incorporation in 3T3 fibroblasts and compared with that present in the contralateral unstimulated muscle. A small increase in heparin-binding mitogenic activity was observed as early as 3 d of stimulation, and by 21 d mitogenic activity increased significantly when normalized to either wet weight (stimulated, 287 +/- 61 GFU/g; unstimulated, 145 +/- 39 GFU/g) or to protein (stimulated, 5.3 +/- 1.1 GFU/mg; unstimulated, 2.2 +/- 0.6 GFU/mg) (+/- SE, P less than 0.05). Western analysis demonstrated increased amounts of peptides with immunological identity to acidic and basic FGFs in stimulated muscle. The increase in FGF content observed in this study is synchronous with neovascularization, myoblast proliferation, and fast to slow fiber type conversion previously shown in this model. These results demonstrate that increased expression of FGFs is associated with motor nerve stimulation and increased tonic contractile activity of skeletal muscle, and suggests that these proteins may play a regulatory role in the cellular changes that occur during exercise conditioning.
Full text
PDF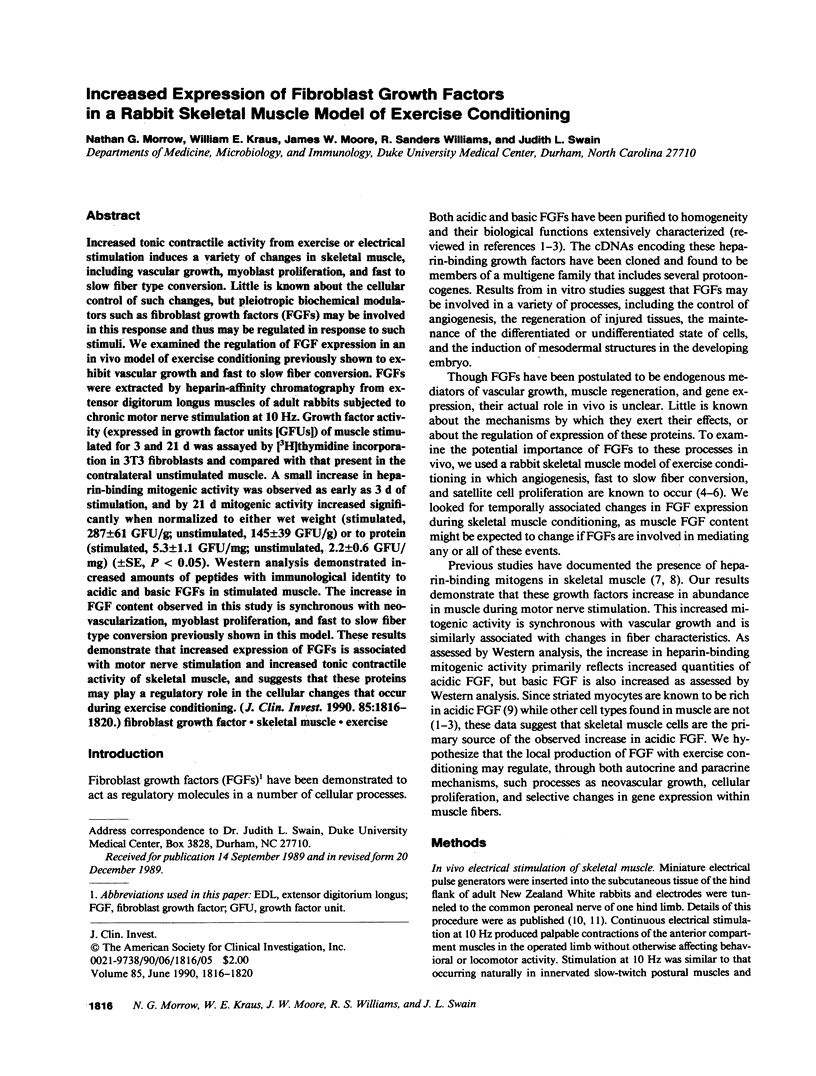
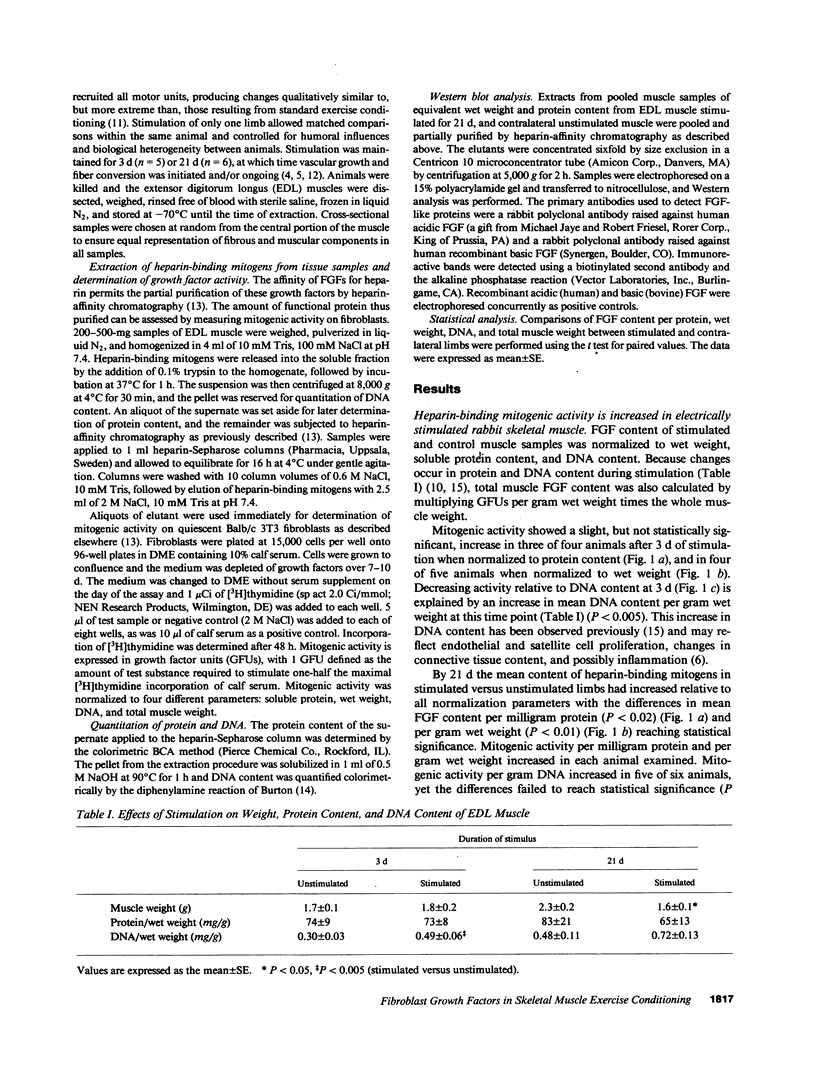
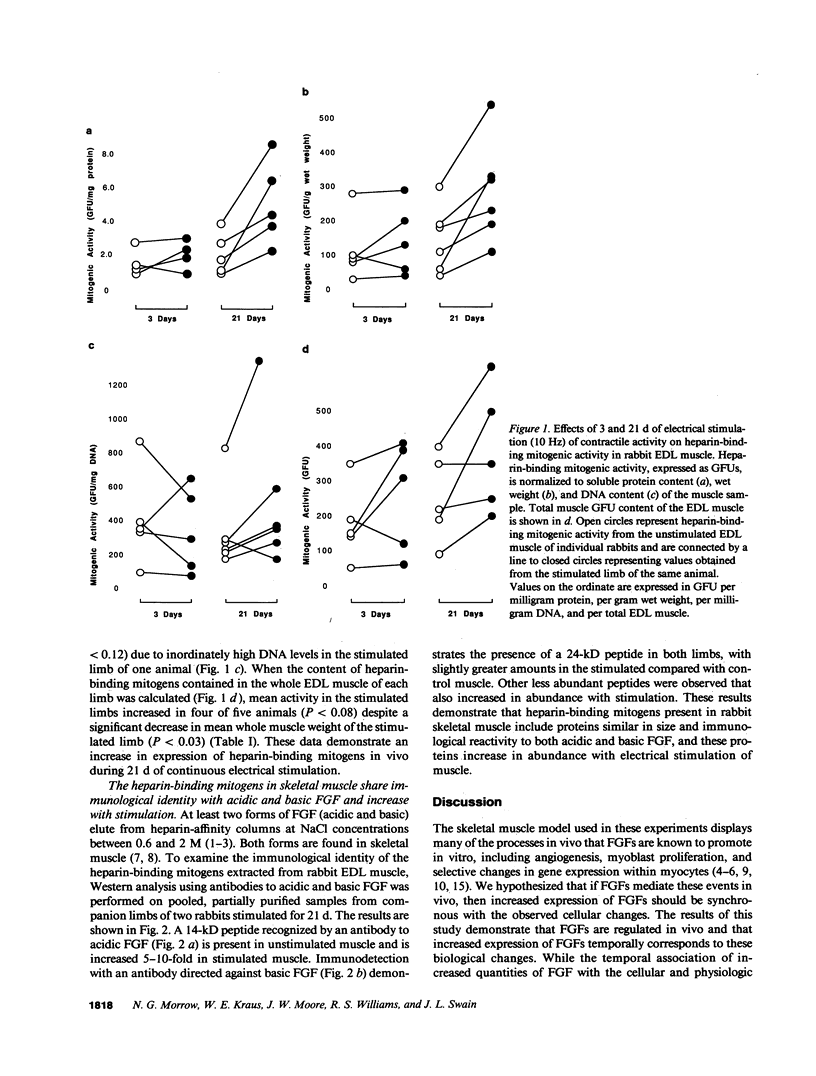
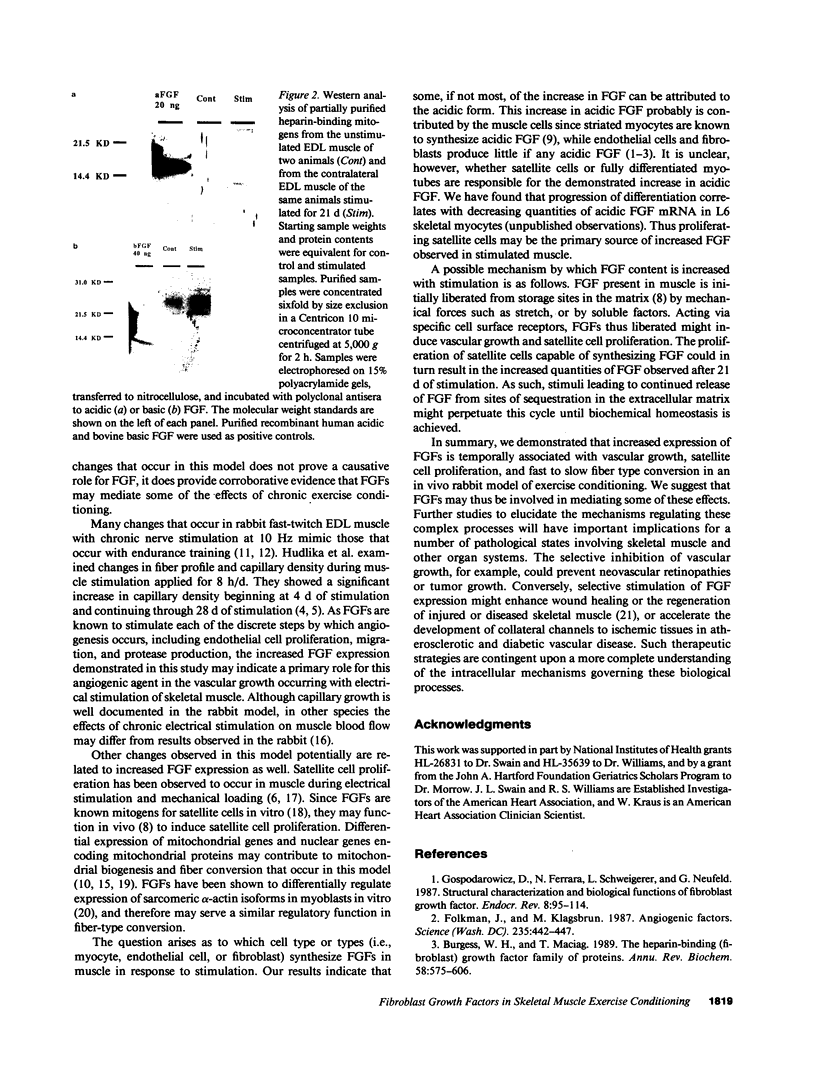
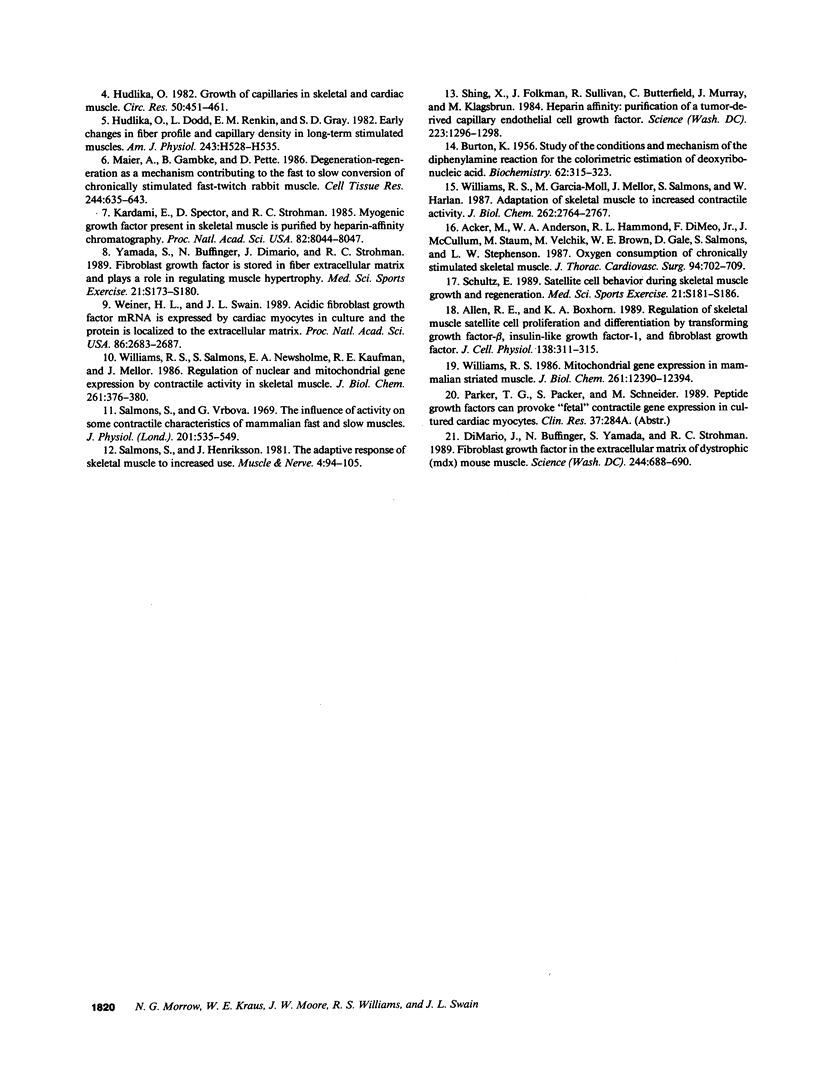
Images in this article
Selected References
These references are in PubMed. This may not be the complete list of references from this article.
- Acker M., Anderson W. A., Hammond R. L., DiMeo F., Jr, McCullum J., Staum M., Velchik M., Brown W. E., Gale D., Salmons S. Oxygen consumption of chronically stimulated skeletal muscle. J Thorac Cardiovasc Surg. 1987 Nov;94(5):702–709. [PubMed] [Google Scholar]
- Allen R. E., Boxhorn L. K. Regulation of skeletal muscle satellite cell proliferation and differentiation by transforming growth factor-beta, insulin-like growth factor I, and fibroblast growth factor. J Cell Physiol. 1989 Feb;138(2):311–315. doi: 10.1002/jcp.1041380213. [DOI] [PubMed] [Google Scholar]
- BURTON K. A study of the conditions and mechanism of the diphenylamine reaction for the colorimetric estimation of deoxyribonucleic acid. Biochem J. 1956 Feb;62(2):315–323. doi: 10.1042/bj0620315. [DOI] [PMC free article] [PubMed] [Google Scholar]
- Burgess W. H., Maciag T. The heparin-binding (fibroblast) growth factor family of proteins. Annu Rev Biochem. 1989;58:575–606. doi: 10.1146/annurev.bi.58.070189.003043. [DOI] [PubMed] [Google Scholar]
- DiMario J., Buffinger N., Yamada S., Strohman R. C. Fibroblast growth factor in the extracellular matrix of dystrophic (mdx) mouse muscle. Science. 1989 May 12;244(4905):688–690. doi: 10.1126/science.2717945. [DOI] [PubMed] [Google Scholar]
- Folkman J., Klagsbrun M. Angiogenic factors. Science. 1987 Jan 23;235(4787):442–447. doi: 10.1126/science.2432664. [DOI] [PubMed] [Google Scholar]
- Gospodarowicz D., Ferrara N., Schweigerer L., Neufeld G. Structural characterization and biological functions of fibroblast growth factor. Endocr Rev. 1987 May;8(2):95–114. doi: 10.1210/edrv-8-2-95. [DOI] [PubMed] [Google Scholar]
- Hudlická O., Dodd L., Renkin E. M., Gray S. D. Early changes in fiber profile and capillary density in long-term stimulated muscles. Am J Physiol. 1982 Oct;243(4):H528–H535. doi: 10.1152/ajpheart.1982.243.4.H528. [DOI] [PubMed] [Google Scholar]
- Hudlická O. Growth of capillaries in skeletal and cardiac muscle. Circ Res. 1982 Apr;50(4):451–461. doi: 10.1161/01.res.50.4.451. [DOI] [PubMed] [Google Scholar]
- Kardami E., Spector D., Strohman R. C. Myogenic growth factor present in skeletal muscle is purified by heparin-affinity chromatography. Proc Natl Acad Sci U S A. 1985 Dec;82(23):8044–8047. doi: 10.1073/pnas.82.23.8044. [DOI] [PMC free article] [PubMed] [Google Scholar]
- Maier A., Gambke B., Pette D. Degeneration-regeneration as a mechanism contributing to the fast to slow conversion of chronically stimulated fast-twitch rabbit muscle. Cell Tissue Res. 1986;244(3):635–643. doi: 10.1007/BF00212544. [DOI] [PubMed] [Google Scholar]
- Salmons S., Henriksson J. The adaptive response of skeletal muscle to increased use. Muscle Nerve. 1981 Mar-Apr;4(2):94–105. doi: 10.1002/mus.880040204. [DOI] [PubMed] [Google Scholar]
- Salmons S., Vrbová G. The influence of activity on some contractile characteristics of mammalian fast and slow muscles. J Physiol. 1969 May;201(3):535–549. doi: 10.1113/jphysiol.1969.sp008771. [DOI] [PMC free article] [PubMed] [Google Scholar]
- Schultz E. Satellite cell behavior during skeletal muscle growth and regeneration. Med Sci Sports Exerc. 1989 Oct;21(5 Suppl):S181–S186. [PubMed] [Google Scholar]
- Shing Y., Folkman J., Sullivan R., Butterfield C., Murray J., Klagsbrun M. Heparin affinity: purification of a tumor-derived capillary endothelial cell growth factor. Science. 1984 Mar 23;223(4642):1296–1299. doi: 10.1126/science.6199844. [DOI] [PubMed] [Google Scholar]
- Weiner H. L., Swain J. L. Acidic fibroblast growth factor mRNA is expressed by cardiac myocytes in culture and the protein is localized to the extracellular matrix. Proc Natl Acad Sci U S A. 1989 Apr;86(8):2683–2687. doi: 10.1073/pnas.86.8.2683. [DOI] [PMC free article] [PubMed] [Google Scholar]
- Williams R. S., Garcia-Moll M., Mellor J., Salmons S., Harlan W. Adaptation of skeletal muscle to increased contractile activity. Expression nuclear genes encoding mitochondrial proteins. J Biol Chem. 1987 Feb 25;262(6):2764–2767. [PubMed] [Google Scholar]
- Williams R. S. Mitochondrial gene expression in mammalian striated muscle. Evidence that variation in gene dosage is the major regulatory event. J Biol Chem. 1986 Sep 15;261(26):12390–12394. [PubMed] [Google Scholar]
- Williams R. S., Salmons S., Newsholme E. A., Kaufman R. E., Mellor J. Regulation of nuclear and mitochondrial gene expression by contractile activity in skeletal muscle. J Biol Chem. 1986 Jan 5;261(1):376–380. [PubMed] [Google Scholar]