Abstract
PEP-19 is a brain-specific polypeptide whose levels increase dramatically during the late maturation of the rodent nervous system. By using immunocytochemistry, PEP-19 is shown to be localized to several regions of the central nervous system, notably cerebellum, thalamus caudate putamen, and olfactory bulb. We have isolated a 0.5-kilobase cDNA clone that encodes the entire PEP-19 protein sequence, making this one of the smallest primary transcripts and translation products ever identified in eukaryotes. The cDNA was used to investigate the developmental expression of PEP-19 in rodent brain. PEP-19 mRNA rises from low levels at embryonic day 17 of gestation in the rat to a plateau value by day 18 postpartum. This mirrored the levels of the protein determined by radioimmunoassay. Since the rise coincided with the formation of synaptic contacts onto Purkinje cells (a major site of PEP-19 expression), the hypothesis was tested that the activity and/or presence of afferent input modulated PEP-19 expression. Parallel fiber innervation was disrupted either by killing granule cells with the cytostatic agent methylazoxymethanol or by examining PEP-19 levels in cerebellar granuloprival mutant mice (reeler and weaver). The influence of climbing fiber input was assessed by either eliminating them with 3-acetylpyridine or stimulating them with harmaline in both neonatal and mature rats. None of the above altered PEP-19 gene expression in cerebellum, leading us to propose that the signals triggering the PEP-19 gene do not emanate from granule cells or neurons in the olivary nucleus. However, preliminary evidence suggests that PEP-19 is under posttranscriptional regulation.
Full text
PDF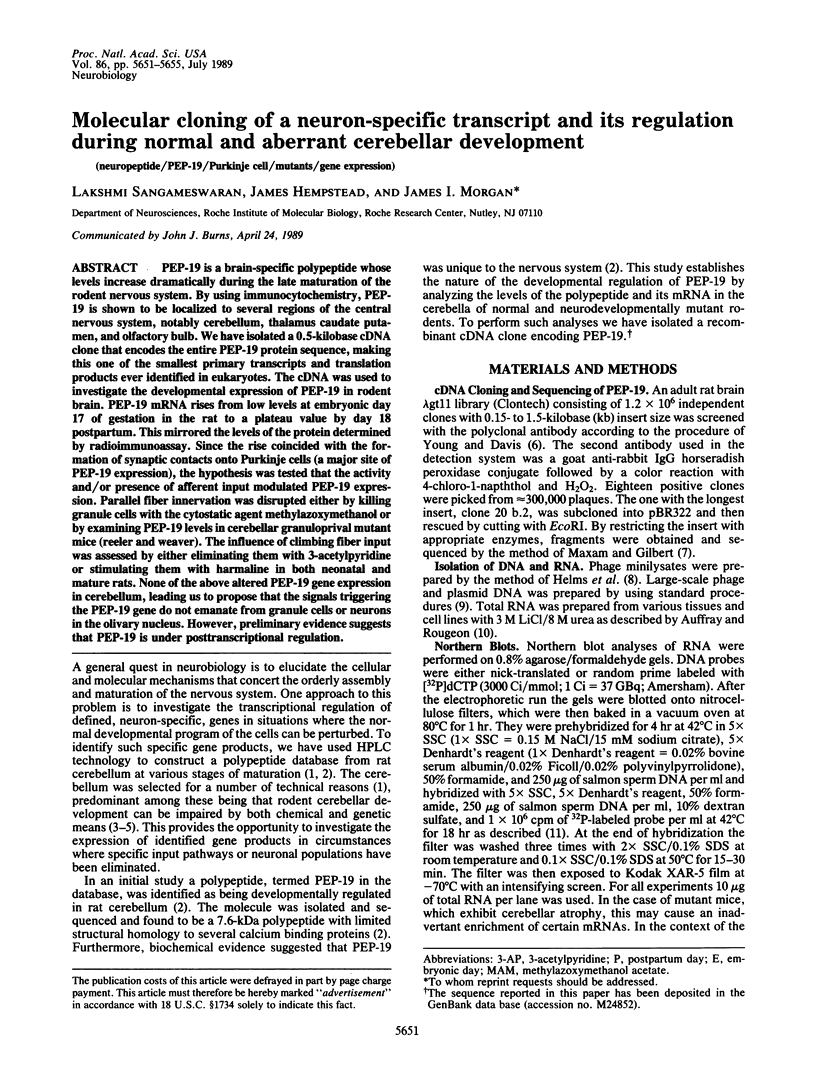
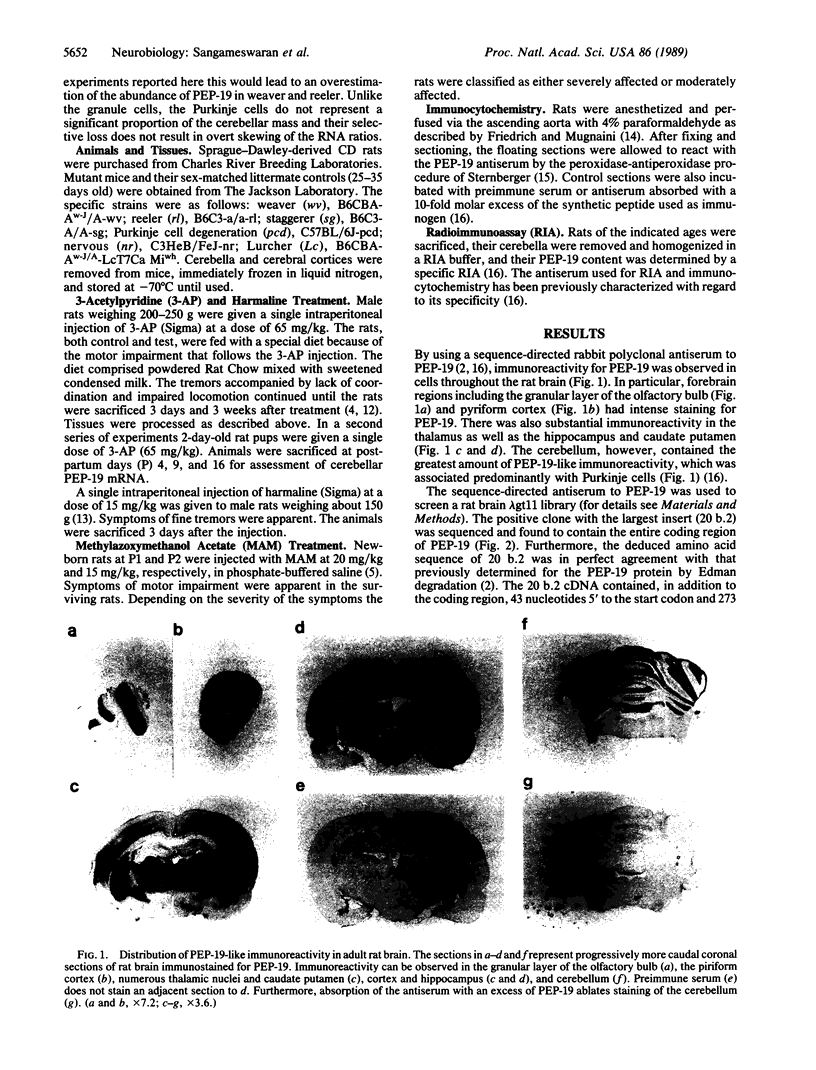
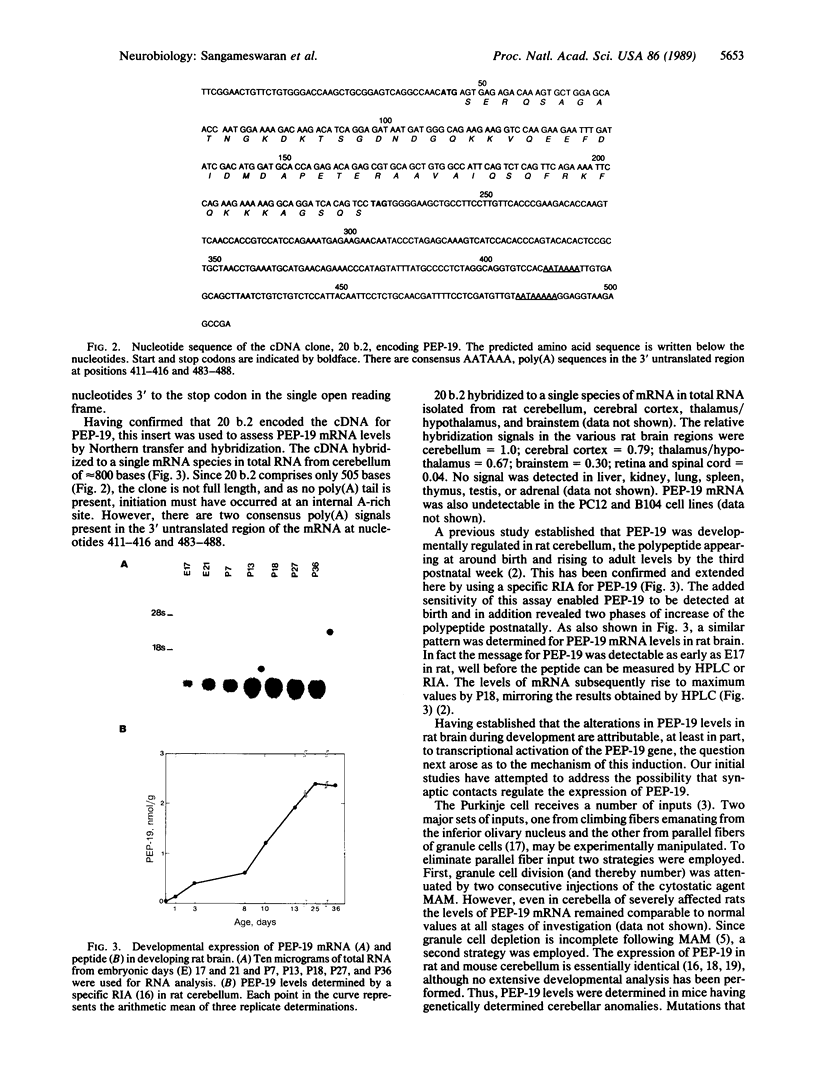
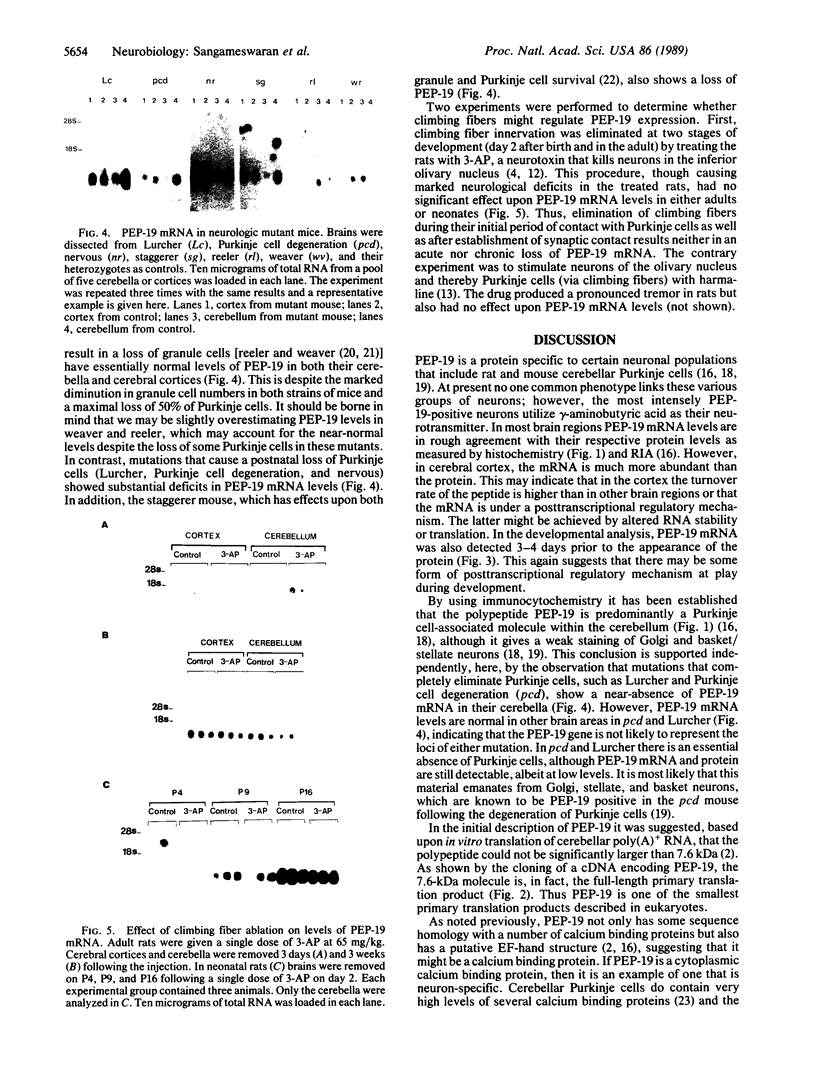
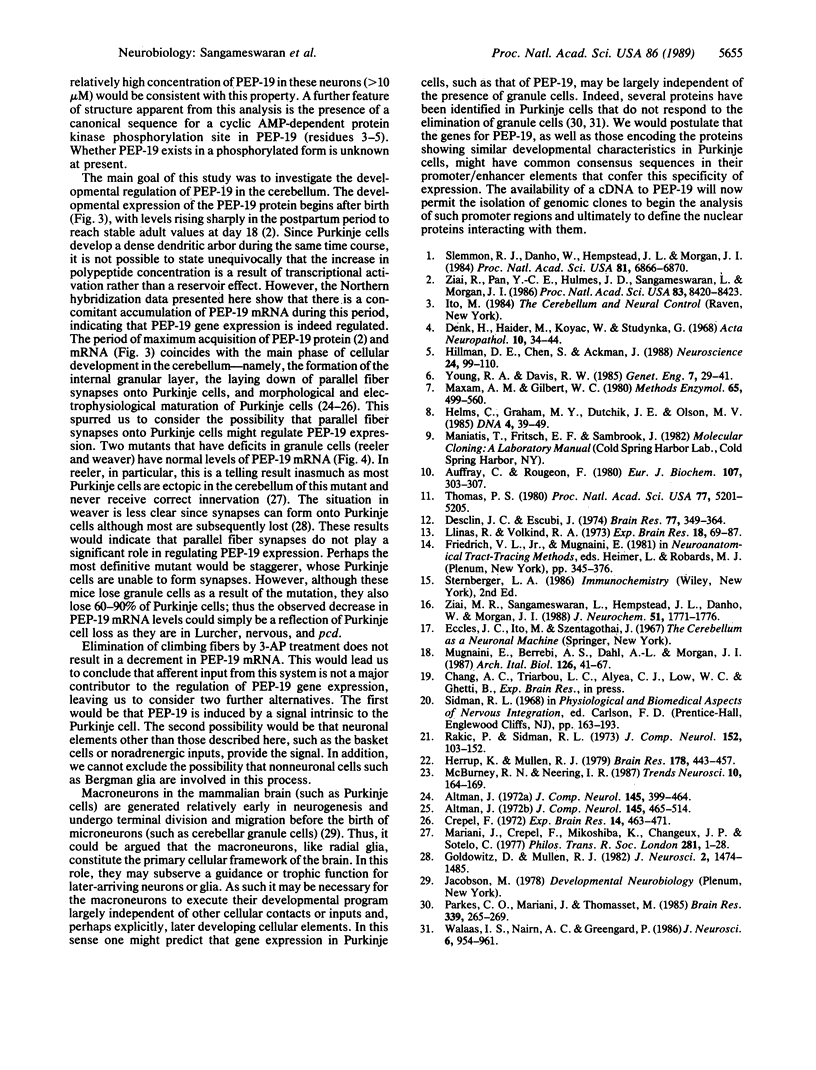
Images in this article
Selected References
These references are in PubMed. This may not be the complete list of references from this article.
- Altman J. Postnatal development of the cerebellar cortex in the rat. 3. Maturation of the components of the granular layer. J Comp Neurol. 1972 Aug;145(4):465–513. doi: 10.1002/cne.901450403. [DOI] [PubMed] [Google Scholar]
- Altman J. Postnatal development of the cerebellar cortex in the rat. II. Phases in the maturation of Purkinje cells and of the molecular layer. J Comp Neurol. 1972 Aug;145(4):399–463. doi: 10.1002/cne.901450402. [DOI] [PubMed] [Google Scholar]
- Auffray C., Rougeon F. Purification of mouse immunoglobulin heavy-chain messenger RNAs from total myeloma tumor RNA. Eur J Biochem. 1980 Jun;107(2):303–314. doi: 10.1111/j.1432-1033.1980.tb06030.x. [DOI] [PubMed] [Google Scholar]
- Denk H., Haider M., Kovac W., Studynka G. Verhaltensänderung und Neuropathologie bei der 3-Acetylpyridinvergiftung der Ratte. Acta Neuropathol. 1968 Jan 2;10(1):34–44. doi: 10.1007/BF00690508. [DOI] [PubMed] [Google Scholar]
- Desclin J. C., Escubi J. Effects of 3-acetylpyridine on the central nervous system of the rat, as demonstrated by silver methods. Brain Res. 1974 Sep 13;77(3):349–364. doi: 10.1016/0006-8993(74)90627-1. [DOI] [PubMed] [Google Scholar]
- Goldowitz D., Mullen R. J. Granule cell as a site of gene action in the weaver mouse cerebellum: evidence from heterozygous mutant chimeras. J Neurosci. 1982 Oct;2(10):1474–1485. doi: 10.1523/JNEUROSCI.02-10-01474.1982. [DOI] [PMC free article] [PubMed] [Google Scholar]
- Helms C., Graham M. Y., Dutchik J. E., Olson M. V. A new method for purifying lambda DNA from phage lysates. DNA. 1985 Feb;4(1):39–49. doi: 10.1089/dna.1985.4.39. [DOI] [PubMed] [Google Scholar]
- Herrup K., Mullen R. J. Staggerer chimeras: intrinsic nature of Purkinje cell defects and implications for normal cerebellar development. Brain Res. 1979 Dec 14;178(2-3):443–457. doi: 10.1016/0006-8993(79)90705-4. [DOI] [PubMed] [Google Scholar]
- Hillman D. E., Chen S., Ackman J. Perinatal methylazoxymethanol acetate uncouples coincidence of orientation of cerebellar folia and parallel fibers. Neuroscience. 1988 Jan;24(1):99–110. doi: 10.1016/0306-4522(88)90315-6. [DOI] [PubMed] [Google Scholar]
- Llinás R., Volkind R. A. The olivo-cerebellar system: functional properties as revealed by harmaline-induced tremor. Exp Brain Res. 1973 Aug 31;18(1):69–87. doi: 10.1007/BF00236557. [DOI] [PubMed] [Google Scholar]
- Mariani J., Crepel F., Mikoshiba K., Changeux J. P., Sotelo C. Anatomical, physiological and biochemical studies of the cerebellum from Reeler mutant mouse. Philos Trans R Soc Lond B Biol Sci. 1977 Nov 2;281(978):1–28. doi: 10.1098/rstb.1977.0121. [DOI] [PubMed] [Google Scholar]
- Maxam A. M., Gilbert W. Sequencing end-labeled DNA with base-specific chemical cleavages. Methods Enzymol. 1980;65(1):499–560. doi: 10.1016/s0076-6879(80)65059-9. [DOI] [PubMed] [Google Scholar]
- Mugnaini E., Berrebi A. S., Dahl A. L., Morgan J. I. The polypeptide PEP-19 is a marker for Purkinje neurons in cerebellar cortex and cartwheel neurons in the dorsal cochlear nucleus. Arch Ital Biol. 1987 Dec;126(1):41–67. [PubMed] [Google Scholar]
- Parkes C. O., Mariani J., Thomasset M. 28 K cholecalcin (CaBP) levels in abnormal cerebella: studies on mutant mice and harmaline- and 3-acetylpyridine-treated rats. Brain Res. 1985 Jul 29;339(2):265–269. doi: 10.1016/0006-8993(85)90091-5. [DOI] [PubMed] [Google Scholar]
- Rakic P., Sidman R. L. Sequence of developmental abnormalities leading to granule cell deficit in cerebellar cortex of weaver mutant mice. J Comp Neurol. 1973 Nov 15;152(2):103–132. doi: 10.1002/cne.901520202. [DOI] [PubMed] [Google Scholar]
- Slemmon J. R., Blacher R., Danho W., Hempstead J. L., Morgan J. I. Isolation and sequencing of two cerebellum-specific peptides. Proc Natl Acad Sci U S A. 1984 Nov;81(21):6866–6870. doi: 10.1073/pnas.81.21.6866. [DOI] [PMC free article] [PubMed] [Google Scholar]
- Thomas P. S. Hybridization of denatured RNA and small DNA fragments transferred to nitrocellulose. Proc Natl Acad Sci U S A. 1980 Sep;77(9):5201–5205. doi: 10.1073/pnas.77.9.5201. [DOI] [PMC free article] [PubMed] [Google Scholar]
- Walaas S. I., Nairn A. C., Greengard P. PCPP-260, a Purkinje cell-specific cyclic AMP-regulated membrane phosphoprotein of Mr 260,000. J Neurosci. 1986 Apr;6(4):954–961. doi: 10.1523/JNEUROSCI.06-04-00954.1986. [DOI] [PMC free article] [PubMed] [Google Scholar]
- Ziai M. R., Sangameswaran L., Hempstead J. L., Danho W., Morgan J. I. An immunochemical analysis of the distribution of a brain-specific polypeptide, PEP-19. J Neurochem. 1988 Dec;51(6):1771–1776. doi: 10.1111/j.1471-4159.1988.tb01158.x. [DOI] [PubMed] [Google Scholar]
- Ziai R., Pan Y. C., Hulmes J. D., Sangameswaran L., Morgan J. I. Isolation, sequence, and developmental profile of a brain-specific polypeptide, PEP-19. Proc Natl Acad Sci U S A. 1986 Nov;83(21):8420–8423. doi: 10.1073/pnas.83.21.8420. [DOI] [PMC free article] [PubMed] [Google Scholar]