Abstract
The question as to whether synaptic vesicles prepared from vertebrate brain can be transported to the active zones of the squid giant synapse was studied by using a combined optical and electrophysiological approach. In order to visualize the behavior of the vertebrate synaptic vesicles in situ, synaptic vesicles isolated from rat brain were labeled with a fluorescent dye (Texas red) and injected into the presynaptic terminal of the squid giant synapse. The pattern of fluorescence that would result from passive diffusion was determined by coinjection of an unconjugated fluorescent dye (fluorescein). The patterns obtained with fluorescent synaptic vesicles were strikingly different from that obtained by simple diffusion of fluorescein. Although the fluorescein diffused freely in both directions, the vesicles moved preferentially into the terminal--i.e., toward the release sites--at a rate of 0.5 microns/sec. The final distribution of the injected fluorescent synaptic vesicles displayed a discrete localization that suggested a distribution coincident with the active zones of the presynaptic terminal. Like fast axonal transport, but unlike fluorescein movements in the terminal, the vesicle movement was energy dependent, since the addition of 2,4-dinitrophenol blocked the redistribution of vesicles completely. In addition, reduction of extracellular calcium concentration reversibly blocked vesicular movement as well. In conclusion, mammalian synaptic vesicles retain the cytoplasmic surface components necessary for translocation, sorting, and targeting to the proper locations by the native machinery of the squid giant synapse.
Full text
PDF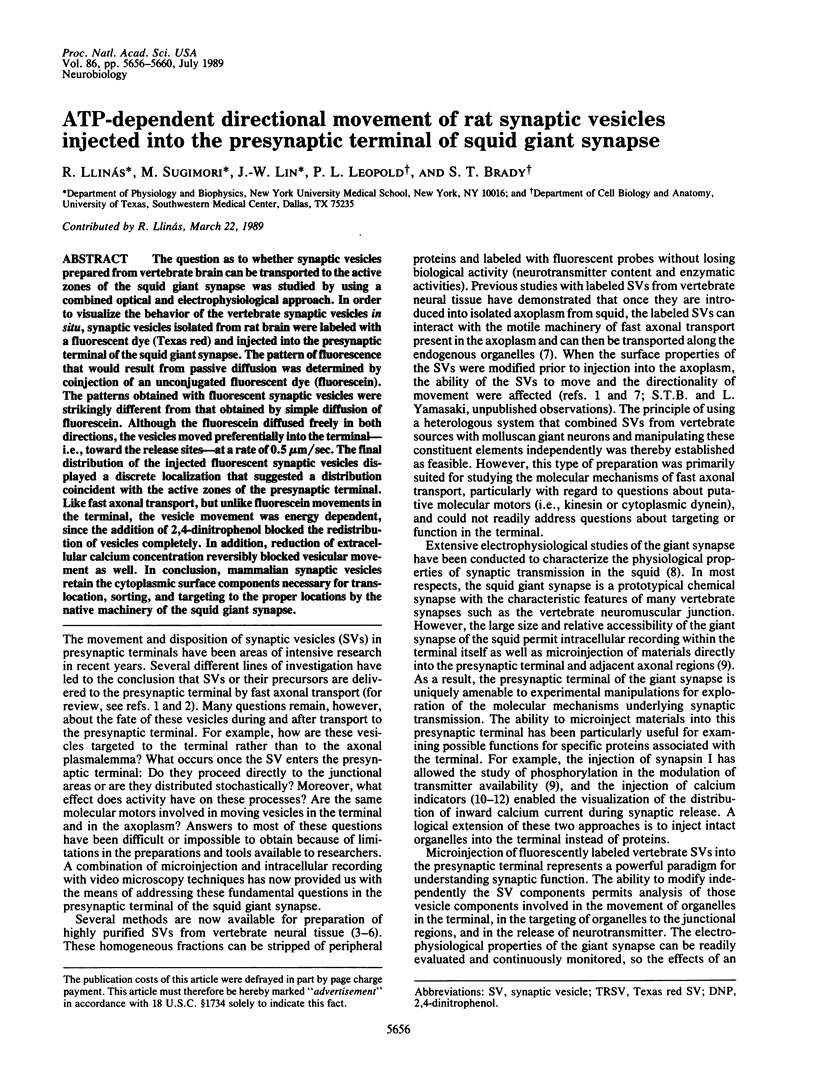
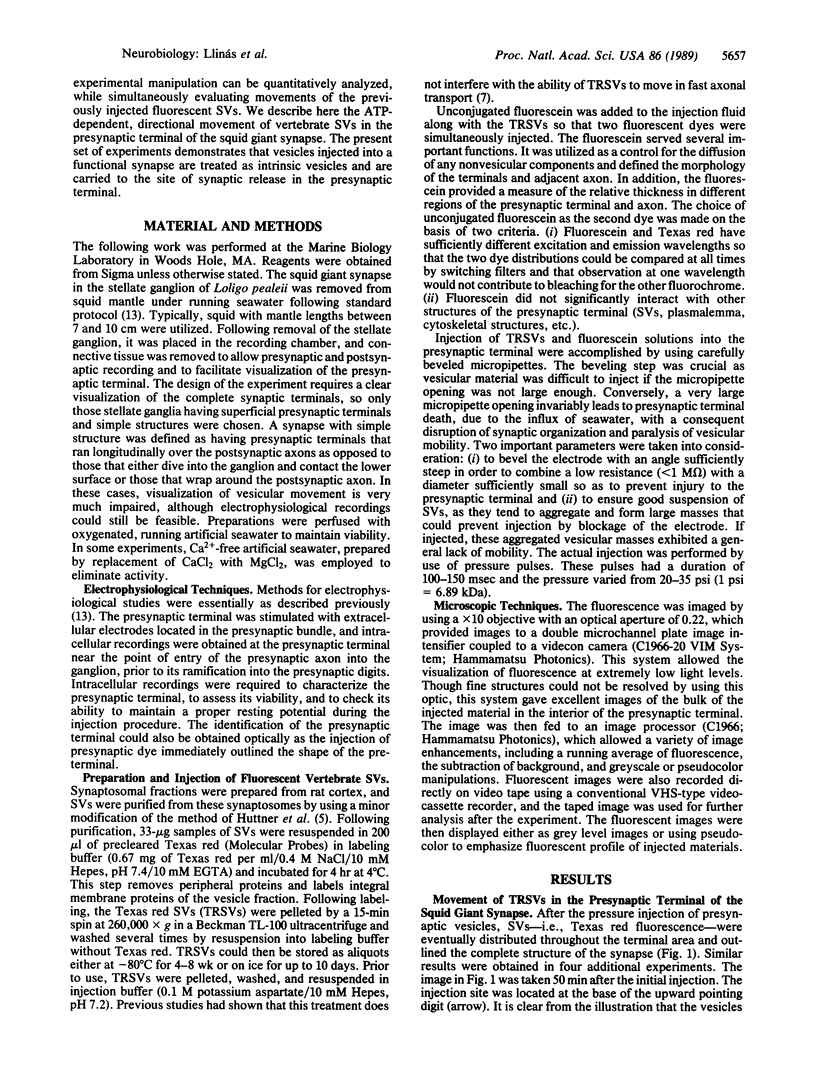
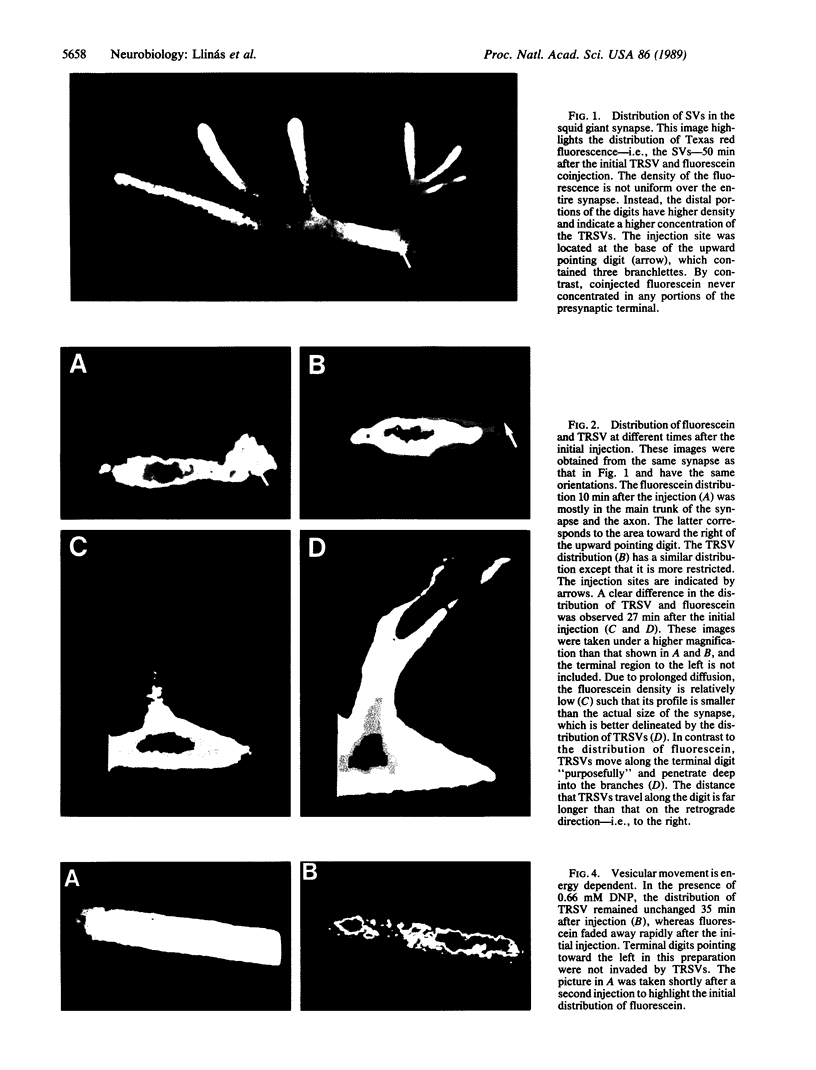
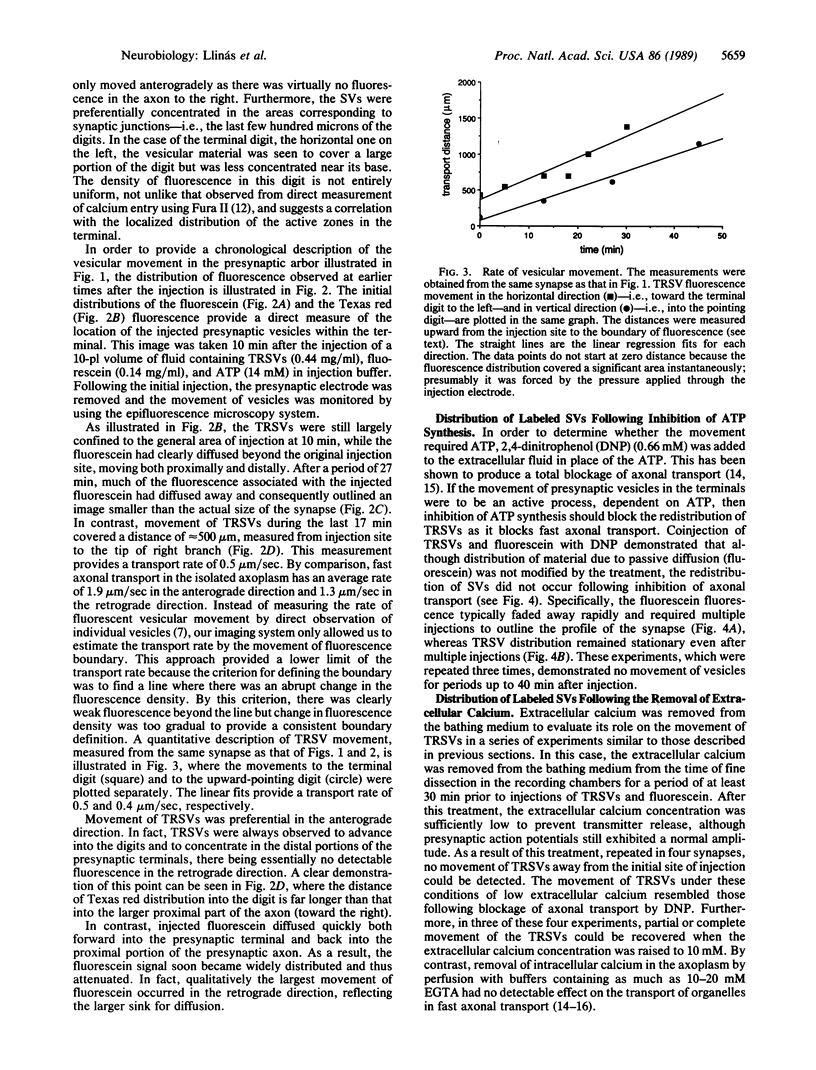
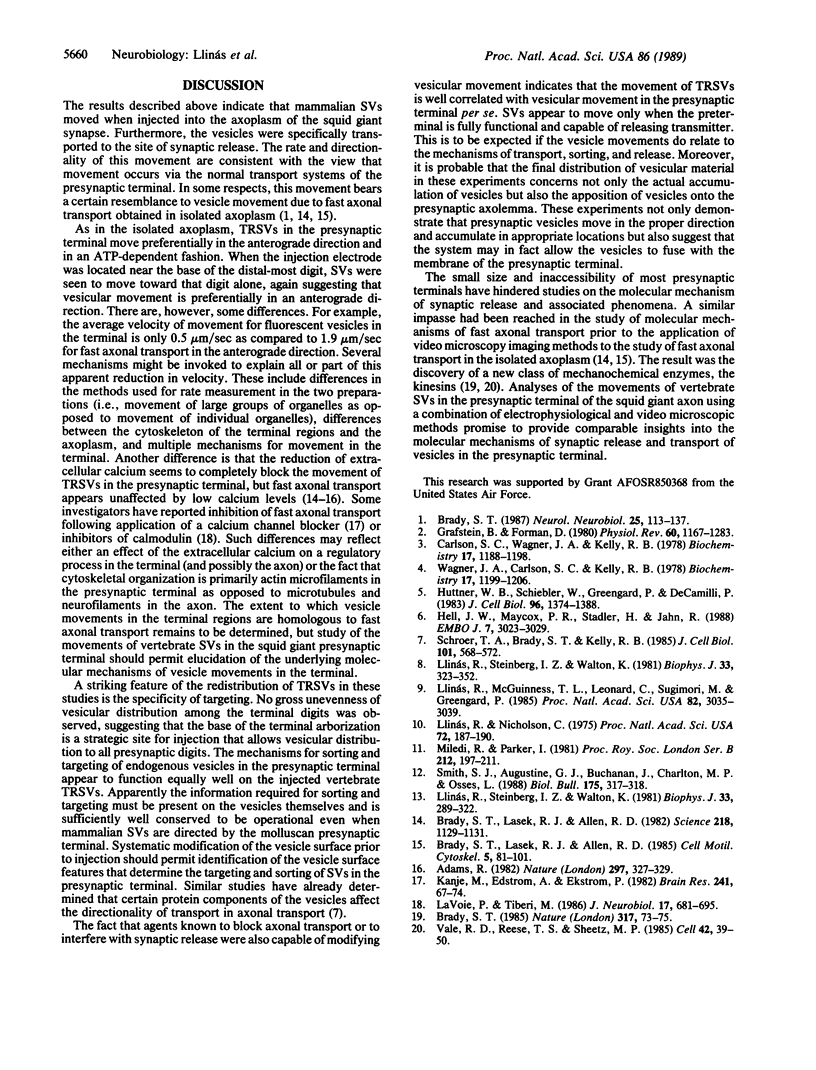
Images in this article
Selected References
These references are in PubMed. This may not be the complete list of references from this article.
- Adams R. J. Organelle movement in axons depends on ATP. Nature. 1982 May 27;297(5864):327–329. doi: 10.1038/297327a0. [DOI] [PubMed] [Google Scholar]
- Brady S. T. A novel brain ATPase with properties expected for the fast axonal transport motor. Nature. 1985 Sep 5;317(6032):73–75. doi: 10.1038/317073a0. [DOI] [PubMed] [Google Scholar]
- Brady S. T., Lasek R. J., Allen R. D. Fast axonal transport in extruded axoplasm from squid giant axon. Science. 1982 Dec 10;218(4577):1129–1131. doi: 10.1126/science.6183745. [DOI] [PubMed] [Google Scholar]
- Brady S. T., Lasek R. J., Allen R. D. Video microscopy of fast axonal transport in extruded axoplasm: a new model for study of molecular mechanisms. Cell Motil. 1985;5(2):81–101. doi: 10.1002/cm.970050203. [DOI] [PubMed] [Google Scholar]
- Carlson S. S., Wagner J. A., Kelly R. B. Purification of synaptic vesicles from elasmobranch electric organ and the use of biophysical criteria to demonstrate purity. Biochemistry. 1978 Apr 4;17(7):1188–1199. doi: 10.1021/bi00600a009. [DOI] [PubMed] [Google Scholar]
- Grafstein B., Forman D. S. Intracellular transport in neurons. Physiol Rev. 1980 Oct;60(4):1167–1283. doi: 10.1152/physrev.1980.60.4.1167. [DOI] [PubMed] [Google Scholar]
- Hell J. W., Maycox P. R., Stadler H., Jahn R. Uptake of GABA by rat brain synaptic vesicles isolated by a new procedure. EMBO J. 1988 Oct;7(10):3023–3029. doi: 10.1002/j.1460-2075.1988.tb03166.x. [DOI] [PMC free article] [PubMed] [Google Scholar]
- Huttner W. B., Schiebler W., Greengard P., De Camilli P. Synapsin I (protein I), a nerve terminal-specific phosphoprotein. III. Its association with synaptic vesicles studied in a highly purified synaptic vesicle preparation. J Cell Biol. 1983 May;96(5):1374–1388. doi: 10.1083/jcb.96.5.1374. [DOI] [PMC free article] [PubMed] [Google Scholar]
- Kanje M., Edström A., Ekström P. Divalent cations and fast axonal transport in chemically desheathed (Triton X-treated) frog sciatic nerve. Brain Res. 1982 Jun 3;241(1):67–74. doi: 10.1016/0006-8993(82)91229-x. [DOI] [PubMed] [Google Scholar]
- Lavoie P. A., Tiberi M. Inhibition of fast axonal transport in bullfrog nerves by dibenzazepine and dibenzocycloheptadiene calmodulin inhibitors. J Neurobiol. 1986 Nov;17(6):681–695. doi: 10.1002/neu.480170611. [DOI] [PubMed] [Google Scholar]
- Llinás R., McGuinness T. L., Leonard C. S., Sugimori M., Greengard P. Intraterminal injection of synapsin I or calcium/calmodulin-dependent protein kinase II alters neurotransmitter release at the squid giant synapse. Proc Natl Acad Sci U S A. 1985 May;82(9):3035–3039. doi: 10.1073/pnas.82.9.3035. [DOI] [PMC free article] [PubMed] [Google Scholar]
- Llinás R., Nicholson C. Calcium role in depolarization-secretion coupling: an aequorin study in squid giant synapse. Proc Natl Acad Sci U S A. 1975 Jan;72(1):187–190. doi: 10.1073/pnas.72.1.187. [DOI] [PMC free article] [PubMed] [Google Scholar]
- Llinás R., Steinberg I. Z., Walton K. Presynaptic calcium currents in squid giant synapse. Biophys J. 1981 Mar;33(3):289–321. doi: 10.1016/S0006-3495(81)84898-9. [DOI] [PMC free article] [PubMed] [Google Scholar]
- Llinás R., Steinberg I. Z., Walton K. Relationship between presynaptic calcium current and postsynaptic potential in squid giant synapse. Biophys J. 1981 Mar;33(3):323–351. doi: 10.1016/S0006-3495(81)84899-0. [DOI] [PMC free article] [PubMed] [Google Scholar]
- Miledi R., Parker I. Calcium transients recorded with arsenazo III in the presynaptic terminal of the squid giant synapse. Proc R Soc Lond B Biol Sci. 1981 May 22;212(1187):197–211. doi: 10.1098/rspb.1981.0034. [DOI] [PubMed] [Google Scholar]
- Schroer T. A., Brady S. T., Kelly R. B. Fast axonal transport of foreign synaptic vesicles in squid axoplasm. J Cell Biol. 1985 Aug;101(2):568–572. doi: 10.1083/jcb.101.2.568. [DOI] [PMC free article] [PubMed] [Google Scholar]
- Vale R. D., Reese T. S., Sheetz M. P. Identification of a novel force-generating protein, kinesin, involved in microtubule-based motility. Cell. 1985 Aug;42(1):39–50. doi: 10.1016/s0092-8674(85)80099-4. [DOI] [PMC free article] [PubMed] [Google Scholar]
- Wagner J. A., Carlson S. S., Kelly R. B. Chemical and physical characterization of cholinergic synaptic vesicles. Biochemistry. 1978 Apr 4;17(7):1199–1206. doi: 10.1021/bi00600a010. [DOI] [PubMed] [Google Scholar]