Abstract
The postsynaptic actions of acetylcholine, adenosine, gamma-aminobutyric acid, histamine, norepinephrine, and serotonin were analyzed in human cortical pyramidal cells maintained in vitro. The actions of these six putative neurotransmitters converged onto three distinct potassium currents. Application of acetylcholine, histamine, norepinephrine, or serotonin all increased spiking by reducing spike-frequency adaptation, in part by reducing the current that underlies the slow after hyperpolarization. In addition, application of muscarinic receptor agonists to all neurons or of serotonin to middle-layer cells substantially reduced or blocked the M-current (a K+ current that is voltage and time dependent). Inhibition of neuronal firing was elicited by adenosine, baclofen (a gamma-aminobutyric acid type B receptor agonist), or serotonin and appeared to be due to an increase in the same potassium current by all three agents. These data reveal that individual neuronal currents in the human cerebral cortex are under the control of several putative neurotransmitters and that each neurotransmitter may exhibit more than one postsynaptic action. The specific anatomical connections of these various neurotransmitter systems, as well as their heterogeneous distribution of postsynaptic receptors and responses, allows each to make a specific contribution to the modulation of cortical activity.
Full text
PDF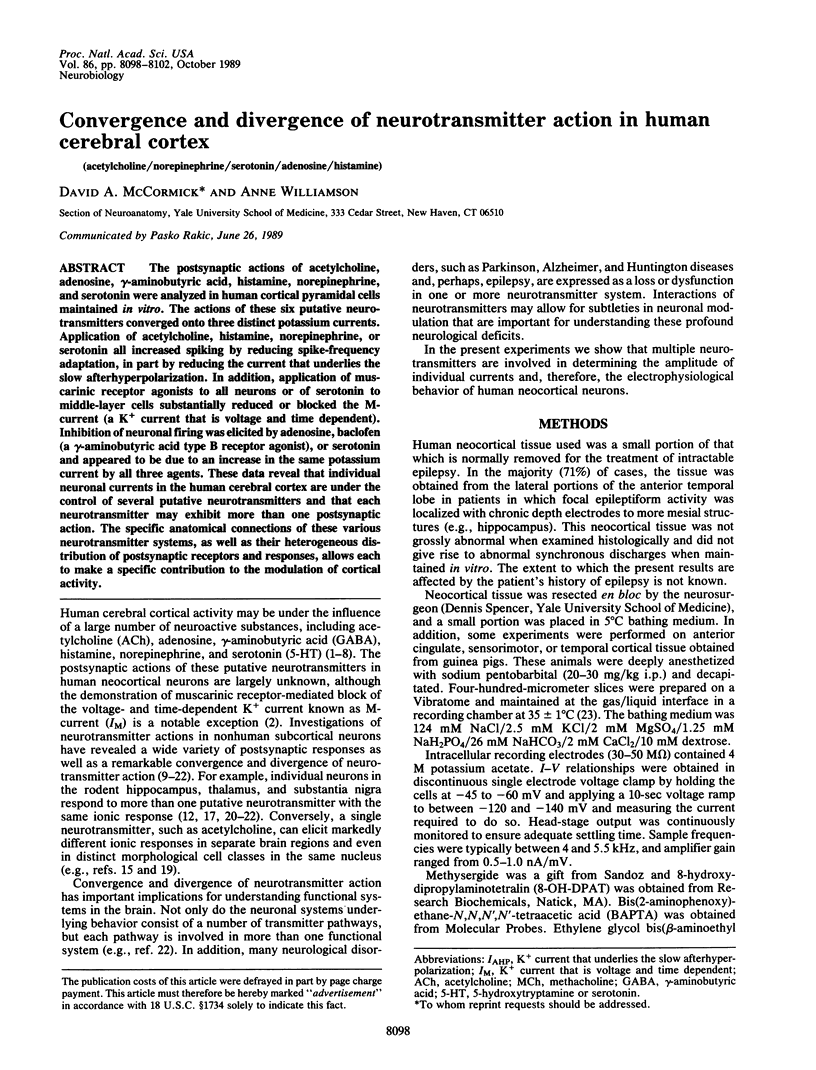
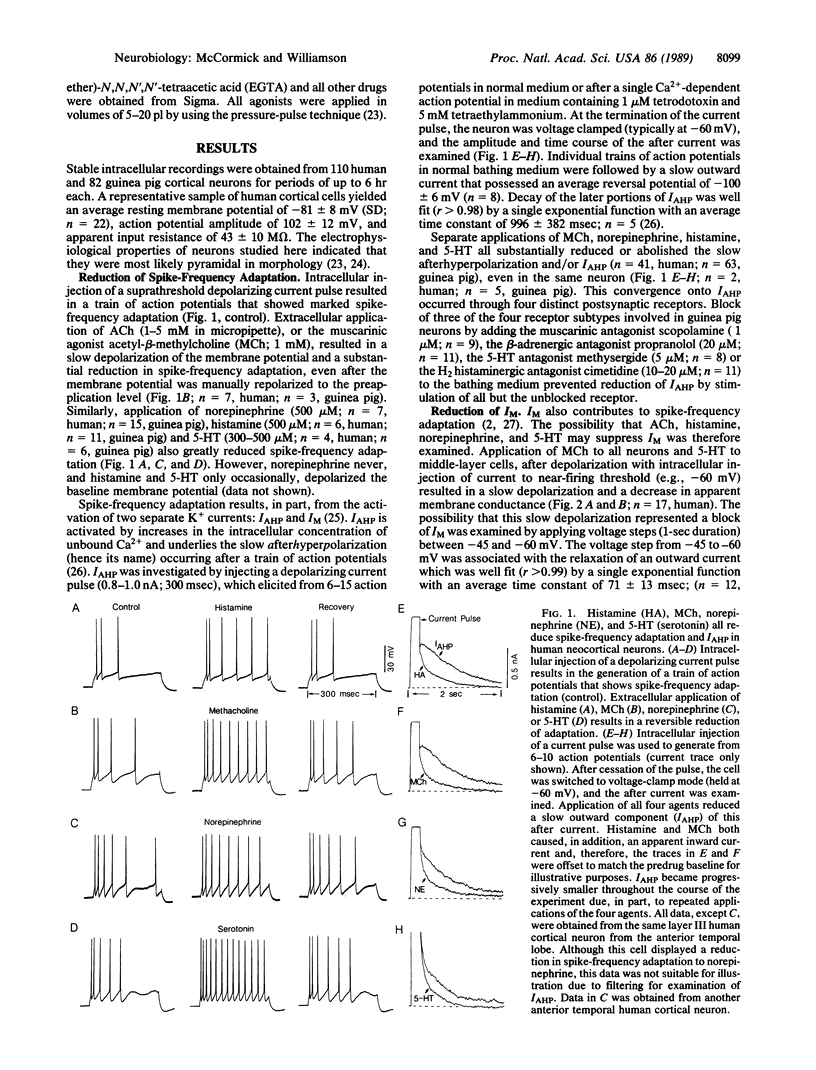
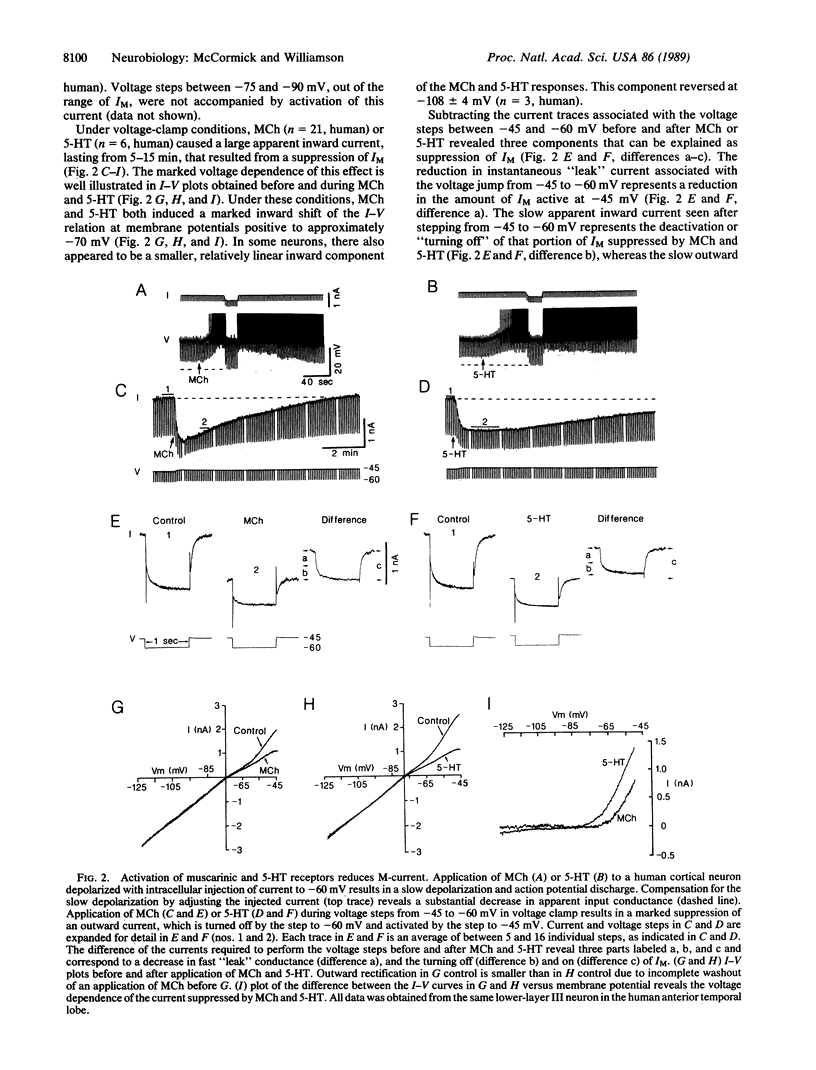
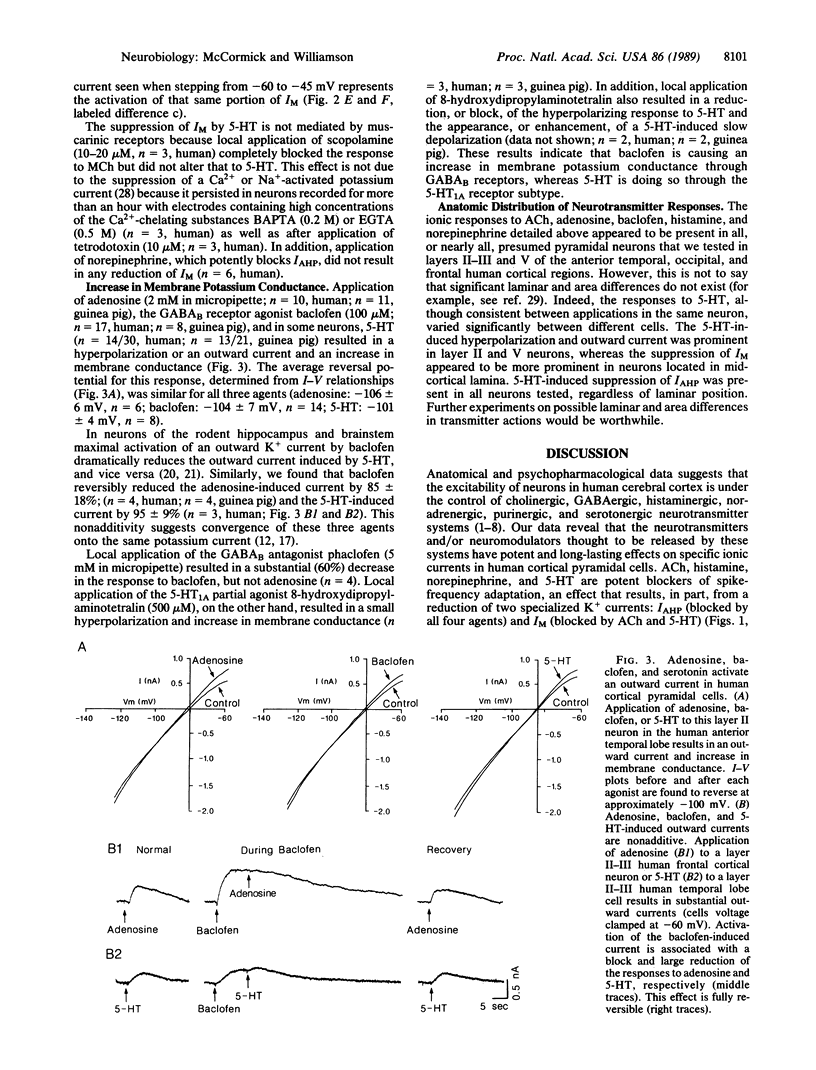
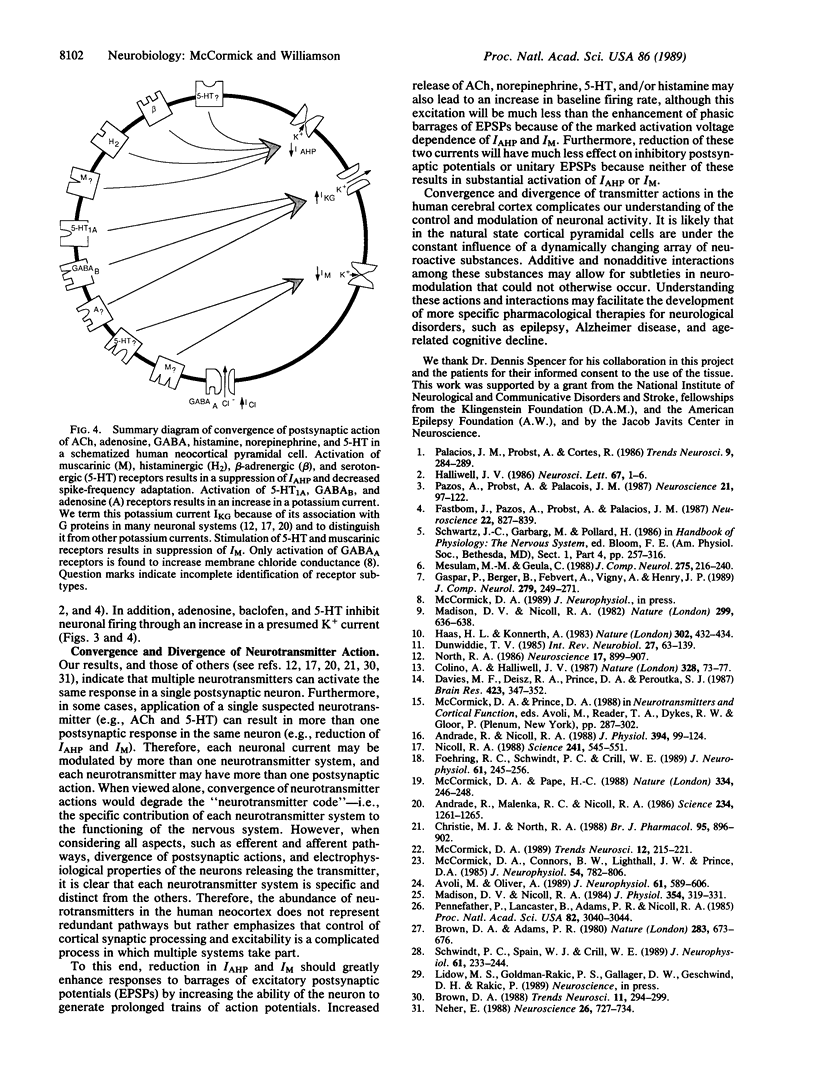
Images in this article
Selected References
These references are in PubMed. This may not be the complete list of references from this article.
- Andrade R., Malenka R. C., Nicoll R. A. A G protein couples serotonin and GABAB receptors to the same channels in hippocampus. Science. 1986 Dec 5;234(4781):1261–1265. doi: 10.1126/science.2430334. [DOI] [PubMed] [Google Scholar]
- Andrade R., Nicoll R. A. Pharmacologically distinct actions of serotonin on single pyramidal neurones of the rat hippocampus recorded in vitro. J Physiol. 1987 Dec;394:99–124. doi: 10.1113/jphysiol.1987.sp016862. [DOI] [PMC free article] [PubMed] [Google Scholar]
- Avoli M., Olivier A. Electrophysiological properties and synaptic responses in the deep layers of the human epileptogenic neocortex in vitro. J Neurophysiol. 1989 Mar;61(3):589–606. doi: 10.1152/jn.1989.61.3.589. [DOI] [PubMed] [Google Scholar]
- Brown D. A., Adams P. R. Muscarinic suppression of a novel voltage-sensitive K+ current in a vertebrate neurone. Nature. 1980 Feb 14;283(5748):673–676. doi: 10.1038/283673a0. [DOI] [PubMed] [Google Scholar]
- Brown D. M-currents: an update. Trends Neurosci. 1988 Jul;11(7):294–299. doi: 10.1016/0166-2236(88)90089-6. [DOI] [PubMed] [Google Scholar]
- Christie M. J., North R. A. Agonists at mu-opioid, M2-muscarinic and GABAB-receptors increase the same potassium conductance in rat lateral parabrachial neurones. Br J Pharmacol. 1988 Nov;95(3):896–902. doi: 10.1111/j.1476-5381.1988.tb11719.x. [DOI] [PMC free article] [PubMed] [Google Scholar]
- Colino A., Halliwell J. V. Differential modulation of three separate K-conductances in hippocampal CA1 neurons by serotonin. Nature. 1987 Jul 2;328(6125):73–77. doi: 10.1038/328073a0. [DOI] [PubMed] [Google Scholar]
- Davies M. F., Deisz R. A., Prince D. A., Peroutka S. J. Two distinct effects of 5-hydroxytryptamine on single cortical neurons. Brain Res. 1987 Oct 13;423(1-2):347–352. doi: 10.1016/0006-8993(87)90861-4. [DOI] [PubMed] [Google Scholar]
- Dunwiddie T. V. The physiological role of adenosine in the central nervous system. Int Rev Neurobiol. 1985;27:63–139. doi: 10.1016/s0074-7742(08)60556-5. [DOI] [PubMed] [Google Scholar]
- Fastbom J., Pazos A., Probst A., Palacios J. M. Adenosine A1 receptors in the human brain: a quantitative autoradiographic study. Neuroscience. 1987 Sep;22(3):827–839. doi: 10.1016/0306-4522(87)92962-9. [DOI] [PubMed] [Google Scholar]
- Foehring R. C., Schwindt P. C., Crill W. E. Norepinephrine selectively reduces slow Ca2+- and Na+-mediated K+ currents in cat neocortical neurons. J Neurophysiol. 1989 Feb;61(2):245–256. doi: 10.1152/jn.1989.61.2.245. [DOI] [PubMed] [Google Scholar]
- Gaspar P., Berger B., Febvret A., Vigny A., Henry J. P. Catecholamine innervation of the human cerebral cortex as revealed by comparative immunohistochemistry of tyrosine hydroxylase and dopamine-beta-hydroxylase. J Comp Neurol. 1989 Jan 8;279(2):249–271. doi: 10.1002/cne.902790208. [DOI] [PubMed] [Google Scholar]
- Haas H. L., Konnerth A. Histamine and noradrenaline decrease calcium-activated potassium conductance in hippocampal pyramidal cells. 1983 Mar 31-Apr 6Nature. 302(5907):432–434. doi: 10.1038/302432a0. [DOI] [PubMed] [Google Scholar]
- Halliwell J. V. M-current in human neocortical neurones. Neurosci Lett. 1986 Jun 6;67(1):1–6. doi: 10.1016/0304-3940(86)90198-9. [DOI] [PubMed] [Google Scholar]
- Madison D. V., Nicoll R. A. Control of the repetitive discharge of rat CA 1 pyramidal neurones in vitro. J Physiol. 1984 Sep;354:319–331. doi: 10.1113/jphysiol.1984.sp015378. [DOI] [PMC free article] [PubMed] [Google Scholar]
- Madison D. V., Nicoll R. A. Noradrenaline blocks accommodation of pyramidal cell discharge in the hippocampus. Nature. 1982 Oct 14;299(5884):636–638. doi: 10.1038/299636a0. [DOI] [PubMed] [Google Scholar]
- McCormick D. A. Cholinergic and noradrenergic modulation of thalamocortical processing. Trends Neurosci. 1989 Jun;12(6):215–221. doi: 10.1016/0166-2236(89)90125-2. [DOI] [PubMed] [Google Scholar]
- McCormick D. A., Connors B. W., Lighthall J. W., Prince D. A. Comparative electrophysiology of pyramidal and sparsely spiny stellate neurons of the neocortex. J Neurophysiol. 1985 Oct;54(4):782–806. doi: 10.1152/jn.1985.54.4.782. [DOI] [PubMed] [Google Scholar]
- McCormick D. A., Pape H. C. Acetylcholine inhibits identified interneurons in the cat lateral geniculate nucleus. Nature. 1988 Jul 21;334(6179):246–248. doi: 10.1038/334246a0. [DOI] [PubMed] [Google Scholar]
- Mesulam M. M., Geula C. Nucleus basalis (Ch4) and cortical cholinergic innervation in the human brain: observations based on the distribution of acetylcholinesterase and choline acetyltransferase. J Comp Neurol. 1988 Sep 8;275(2):216–240. doi: 10.1002/cne.902750205. [DOI] [PubMed] [Google Scholar]
- Neher E. The use of the patch clamp technique to study second messenger-mediated cellular events. Neuroscience. 1988 Sep;26(3):727–734. doi: 10.1016/0306-4522(88)90094-2. [DOI] [PubMed] [Google Scholar]
- Nicoll R. A. The coupling of neurotransmitter receptors to ion channels in the brain. Science. 1988 Jul 29;241(4865):545–551. doi: 10.1126/science.2456612. [DOI] [PubMed] [Google Scholar]
- North R. A. Receptors on individual neurones. Neuroscience. 1986 Apr;17(4):899–907. doi: 10.1016/0306-4522(86)90070-9. [DOI] [PubMed] [Google Scholar]
- Pazos A., Probst A., Palacios J. M. Serotonin receptors in the human brain--III. Autoradiographic mapping of serotonin-1 receptors. Neuroscience. 1987 Apr;21(1):97–122. doi: 10.1016/0306-4522(87)90326-5. [DOI] [PubMed] [Google Scholar]
- Pennefather P., Lancaster B., Adams P. R., Nicoll R. A. Two distinct Ca-dependent K currents in bullfrog sympathetic ganglion cells. Proc Natl Acad Sci U S A. 1985 May;82(9):3040–3044. doi: 10.1073/pnas.82.9.3040. [DOI] [PMC free article] [PubMed] [Google Scholar]
- Schwindt P. C., Spain W. J., Crill W. E. Long-lasting reduction of excitability by a sodium-dependent potassium current in cat neocortical neurons. J Neurophysiol. 1989 Feb;61(2):233–244. doi: 10.1152/jn.1989.61.2.233. [DOI] [PubMed] [Google Scholar]