Abstract
We examined the energy requirement for maltose transport in right-side-out membrane vesicles derived from Escherichia coli. When membrane vesicles were made from strains producing tethered maltose-binding proteins by dilution of spheroplasts into phosphate buffer, those from an F0F1 ATPase-containing (unc+) strain transported maltose in the presence of an exogenous electron donor, such as ascorbate/phenazine methosulfate, at a rate of 1-5 nmol/min per mg of protein, whereas those from an isogenic unc- strain failed to transport maltose. Transport in vesicles obtained from the latter strain could be restored in the presence of electron donors if the vesicles were made to contain NAD+ and either ATP or an ATP-regenerating system. ATP hydrolysis was apparently required for transport, since nonhydrolyzable ATP analogues did not sustain transport. Maltose transport significantly increased ATP hydrolysis in ATP-containing vesicles from unc- cells. Finally, ATP-containing vesicles from unc- strains producing normal maltose-binding proteins could accumulate maltose in the absence of electron donors. These results provide convincing evidence that it is the hydrolysis of ATP that drives maltose transport, and probably also other periplasmic-binding-protein-dependent transport systems.
Full text
PDF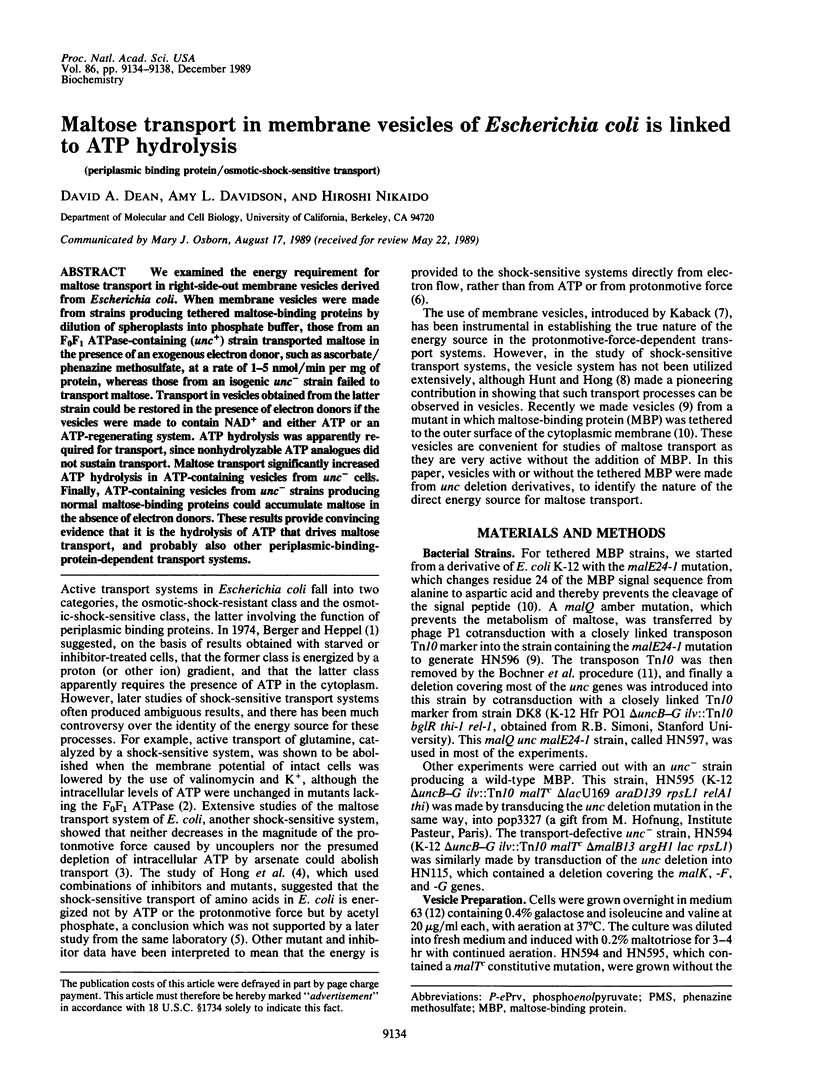
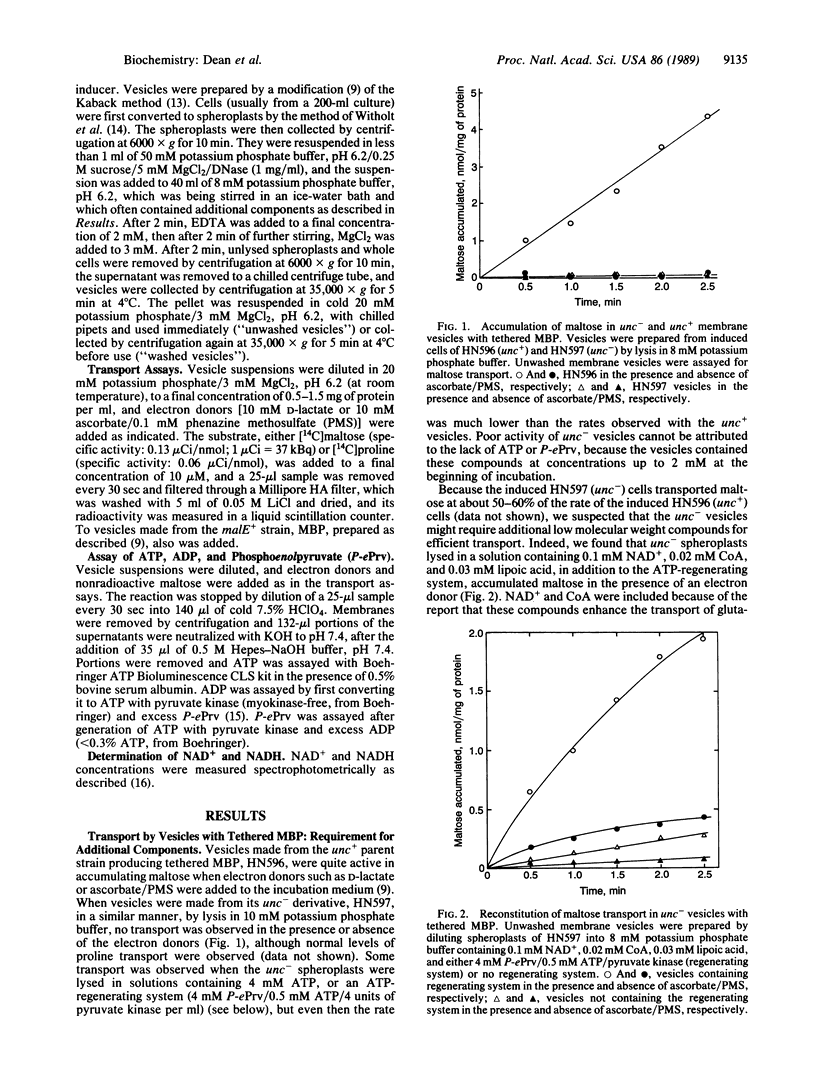
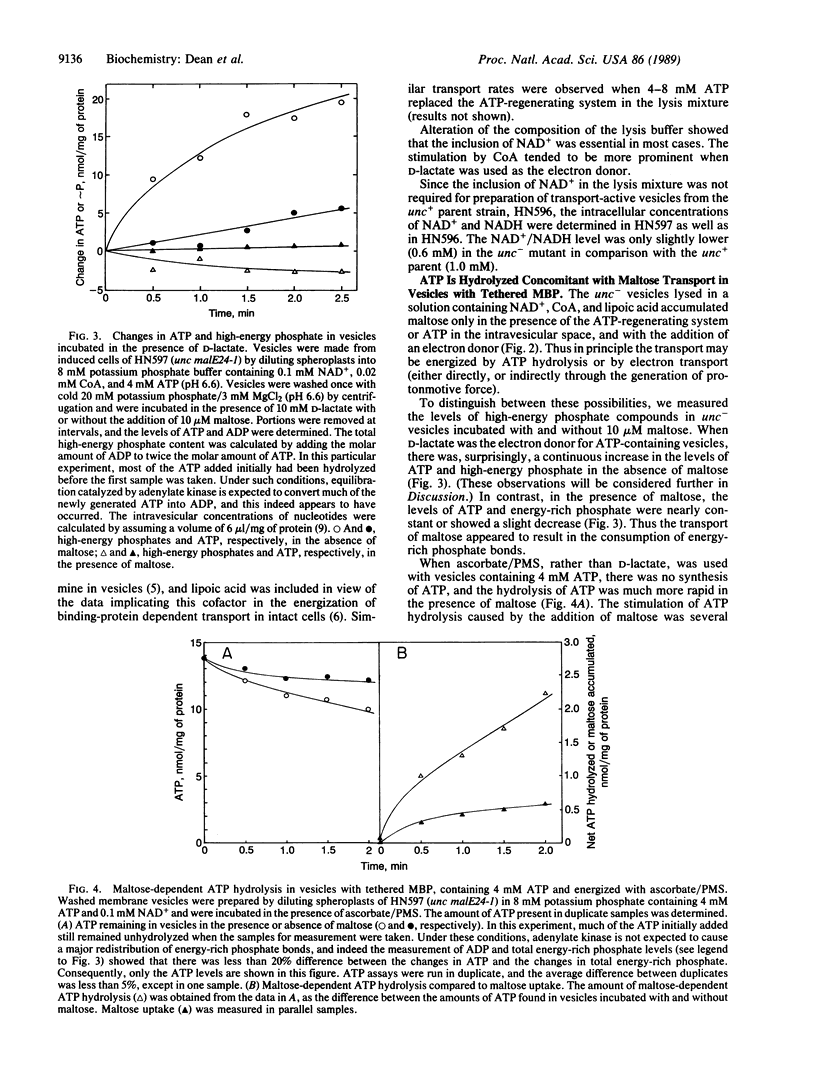
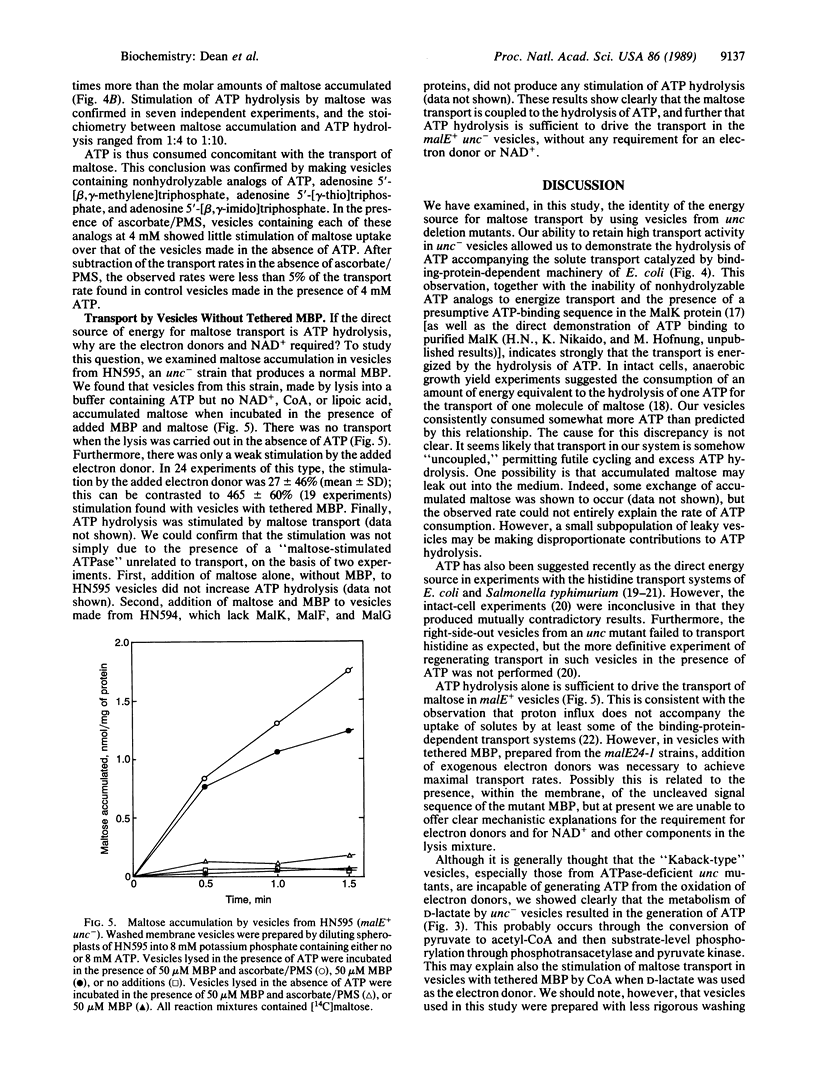
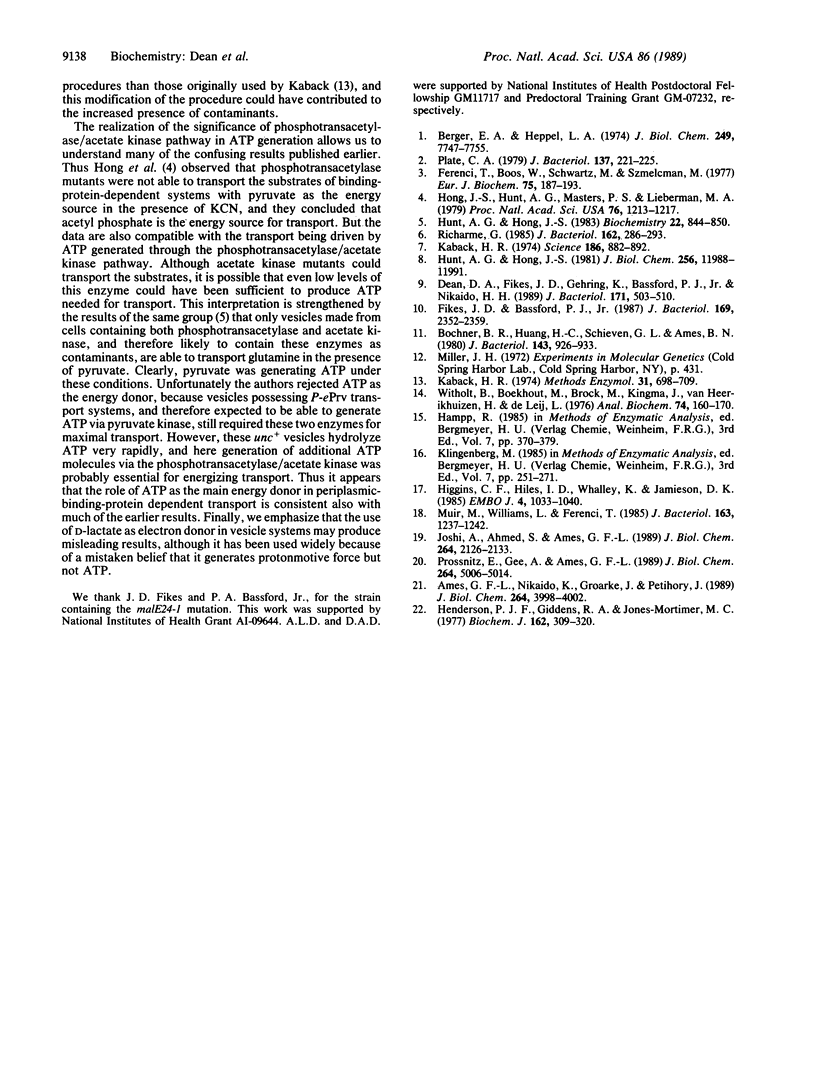
Selected References
These references are in PubMed. This may not be the complete list of references from this article.
- Ames G. F., Nikaido K., Groarke J., Petithory J. Reconstitution of periplasmic transport in inside-out membrane vesicles. Energization by ATP. J Biol Chem. 1989 Mar 5;264(7):3998–4002. [PubMed] [Google Scholar]
- Berger E. A., Heppel L. A. Different mechanisms of energy coupling for the shock-sensitive and shock-resistant amino acid permeases of Escherichia coli. J Biol Chem. 1974 Dec 25;249(24):7747–7755. [PubMed] [Google Scholar]
- Bochner B. R., Huang H. C., Schieven G. L., Ames B. N. Positive selection for loss of tetracycline resistance. J Bacteriol. 1980 Aug;143(2):926–933. doi: 10.1128/jb.143.2.926-933.1980. [DOI] [PMC free article] [PubMed] [Google Scholar]
- Dean D. A., Fikes J. D., Gehring K., Bassford P. J., Jr, Nikaido H. Active transport of maltose in membrane vesicles obtained from Escherichia coli cells producing tethered maltose-binding protein. J Bacteriol. 1989 Jan;171(1):503–510. doi: 10.1128/jb.171.1.503-510.1989. [DOI] [PMC free article] [PubMed] [Google Scholar]
- Ferenci T., Boos W., Schwartz M., Szmelcman S. Energy-coupling of the transport system of Escherichia coli dependent on maltose-binding protein. Eur J Biochem. 1977 May 2;75(1):187–193. doi: 10.1111/j.1432-1033.1977.tb11516.x. [DOI] [PubMed] [Google Scholar]
- Fikes J. D., Bassford P. J., Jr Export of unprocessed precursor maltose-binding protein to the periplasm of Escherichia coli cells. J Bacteriol. 1987 Jun;169(6):2352–2359. doi: 10.1128/jb.169.6.2352-2359.1987. [DOI] [PMC free article] [PubMed] [Google Scholar]
- Henderson P. J., Giddens R. A., Jones-Mortimer M. C. Transport of galactose, glucose and their molecular analogues by Escherichia coli K12. Biochem J. 1977 Feb 15;162(2):309–320. doi: 10.1042/bj1620309. [DOI] [PMC free article] [PubMed] [Google Scholar]
- Higgins C. F., Hiles I. D., Whalley K., Jamieson D. J. Nucleotide binding by membrane components of bacterial periplasmic binding protein-dependent transport systems. EMBO J. 1985 Apr;4(4):1033–1039. doi: 10.1002/j.1460-2075.1985.tb03735.x. [DOI] [PMC free article] [PubMed] [Google Scholar]
- Hong J. S., Hunt A. G., Masters P. S., Lieberman M. A. Requirements of acetyl phosphate for the binding protein-dependent transport systems in Escherichia coli. Proc Natl Acad Sci U S A. 1979 Mar;76(3):1213–1217. doi: 10.1073/pnas.76.3.1213. [DOI] [PMC free article] [PubMed] [Google Scholar]
- Hunt A. G., Hong J. Properties and characterization of binding protein dependent active transport of glutamine in isolated membrane vesicles of Escherichia coli. Biochemistry. 1983 Feb 15;22(4):844–850. doi: 10.1021/bi00273a021. [DOI] [PubMed] [Google Scholar]
- Hunt A. G., Hong J. The reconstitution of binding protein-dependent active transport of glutamine in isolated membrane vesicles from Escherichia coli. J Biol Chem. 1981 Dec 10;256(23):11988–11991. [PubMed] [Google Scholar]
- Joshi A. K., Ahmed S., Ferro-Luzzi Ames G. Energy coupling in bacterial periplasmic transport systems. Studies in intact Escherichia coli cells. J Biol Chem. 1989 Feb 5;264(4):2126–2133. [PubMed] [Google Scholar]
- Kaback H. R. Transport in isolated bacterial membrane vesicles. Methods Enzymol. 1974;31:698–709. doi: 10.1016/0076-6879(74)31075-0. [DOI] [PubMed] [Google Scholar]
- Kaback H. R. Transport studies in bacterial membrane vesicles. Science. 1974 Dec 6;186(4167):882–892. doi: 10.1126/science.186.4167.882. [DOI] [PubMed] [Google Scholar]
- Muir M., Williams L., Ferenci T. Influence of transport energization on the growth yield of Escherichia coli. J Bacteriol. 1985 Sep;163(3):1237–1242. doi: 10.1128/jb.163.3.1237-1242.1985. [DOI] [PMC free article] [PubMed] [Google Scholar]
- Plate C. A. Requirement for membrane potential in active transport of glutamine by Escherichia coli. J Bacteriol. 1979 Jan;137(1):221–225. doi: 10.1128/jb.137.1.221-225.1979. [DOI] [PMC free article] [PubMed] [Google Scholar]
- Prossnitz E., Gee A., Ames G. F. Reconstitution of the histidine periplasmic transport system in membrane vesicles. Energy coupling and interaction between the binding protein and the membrane complex. J Biol Chem. 1989 Mar 25;264(9):5006–5014. [PubMed] [Google Scholar]
- Richarme G. Possible involvement of lipoic acid in binding protein-dependent transport systems in Escherichia coli. J Bacteriol. 1985 Apr;162(1):286–293. doi: 10.1128/jb.162.1.286-293.1985. [DOI] [PMC free article] [PubMed] [Google Scholar]
- Witholt B., Boekhout M., Brock M., Kingma J., Heerikhuizen H. V., Leij L. D. An efficient and reproducible procedure for the formation of spheroplasts from variously grown Escherichia coli. Anal Biochem. 1976 Jul;74(1):160–170. doi: 10.1016/0003-2697(76)90320-1. [DOI] [PubMed] [Google Scholar]