Abstract
In a previous paper, a model was presented showing how the group of Ca2+/calmodulin-dependent protein kinase II molecules contained within a postsynaptic density could stably store a graded synaptic weight. This paper completes the model by showing how bidirectional control of synaptic weight could be achieved. It is proposed that the quantitative level of the activity-dependent rise in postsynaptic Ca2+ determines whether the synaptic weight will increase or decrease. It is further proposed that reduction of synaptic weight is governed by protein phosphatase 1, an enzyme indirectly controlled by Ca2+ through reactions involving phosphatase inhibitor 1, cAMP-dependent protein kinase, calcineurin, and adenylate cyclase. Modeling of this biochemical system shows that it can function as an analog computer that can store a synaptic weight and modify it in accord with the Hebb and anti-Hebb learning rules.
Full text
PDF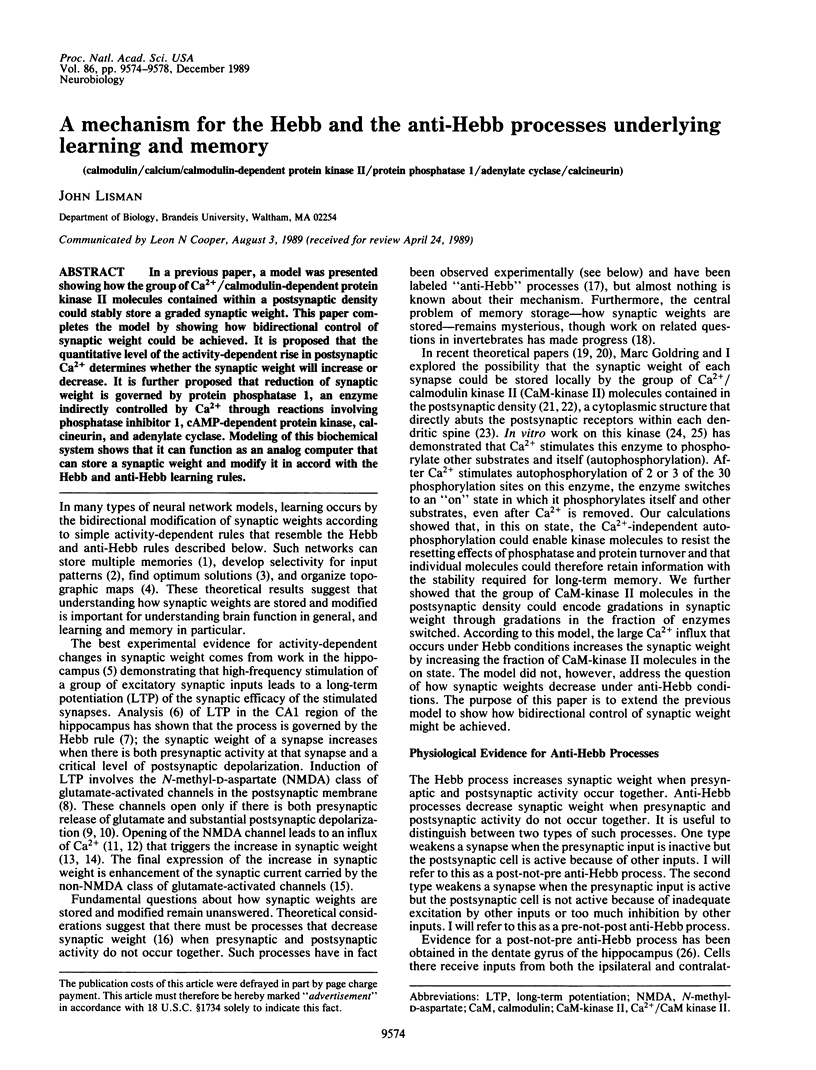
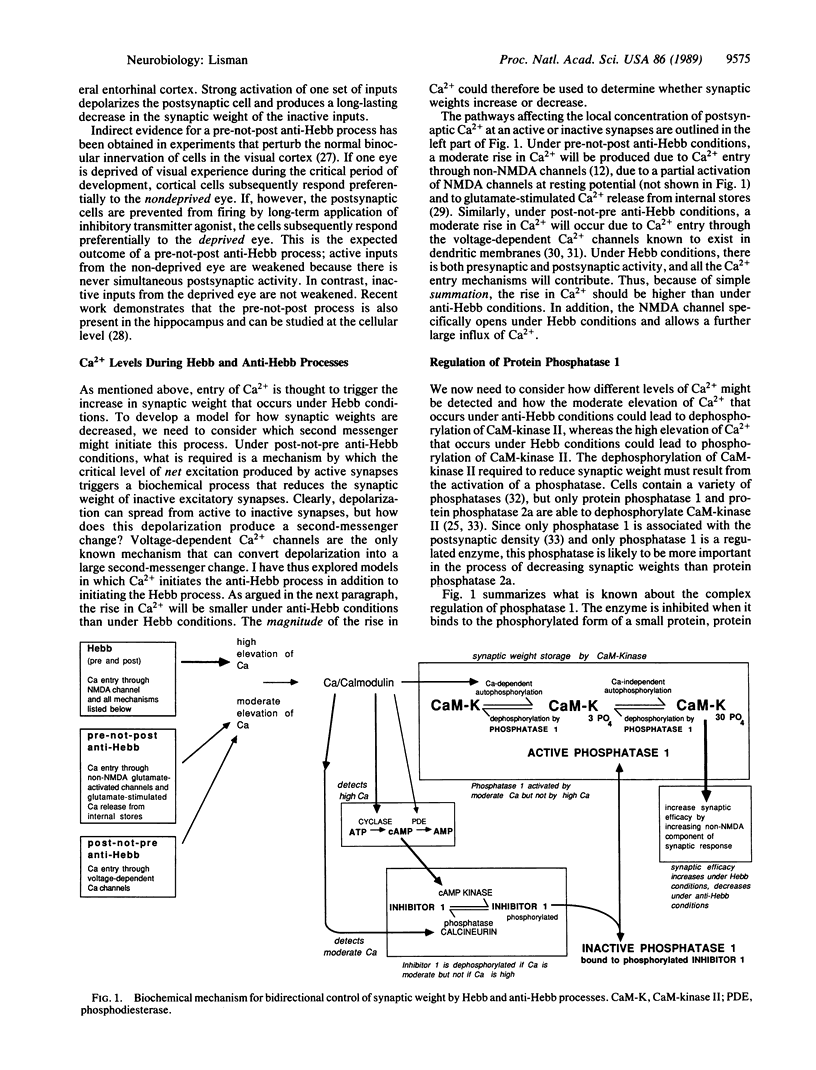
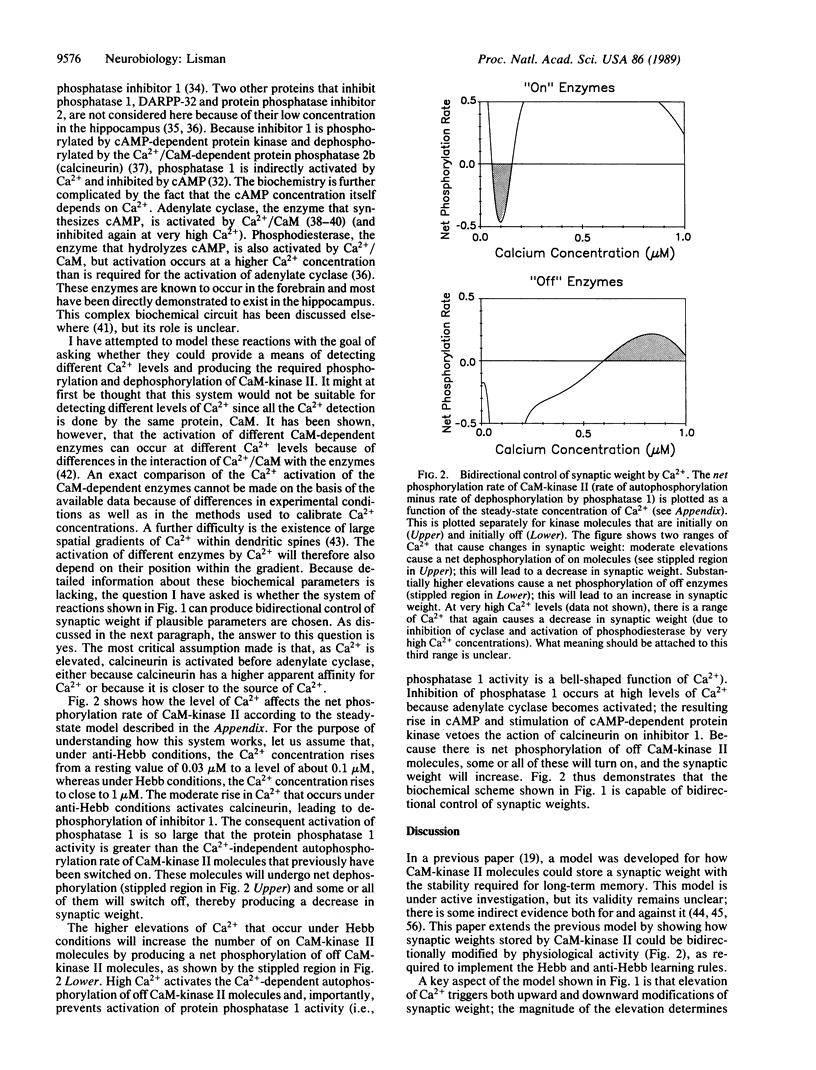
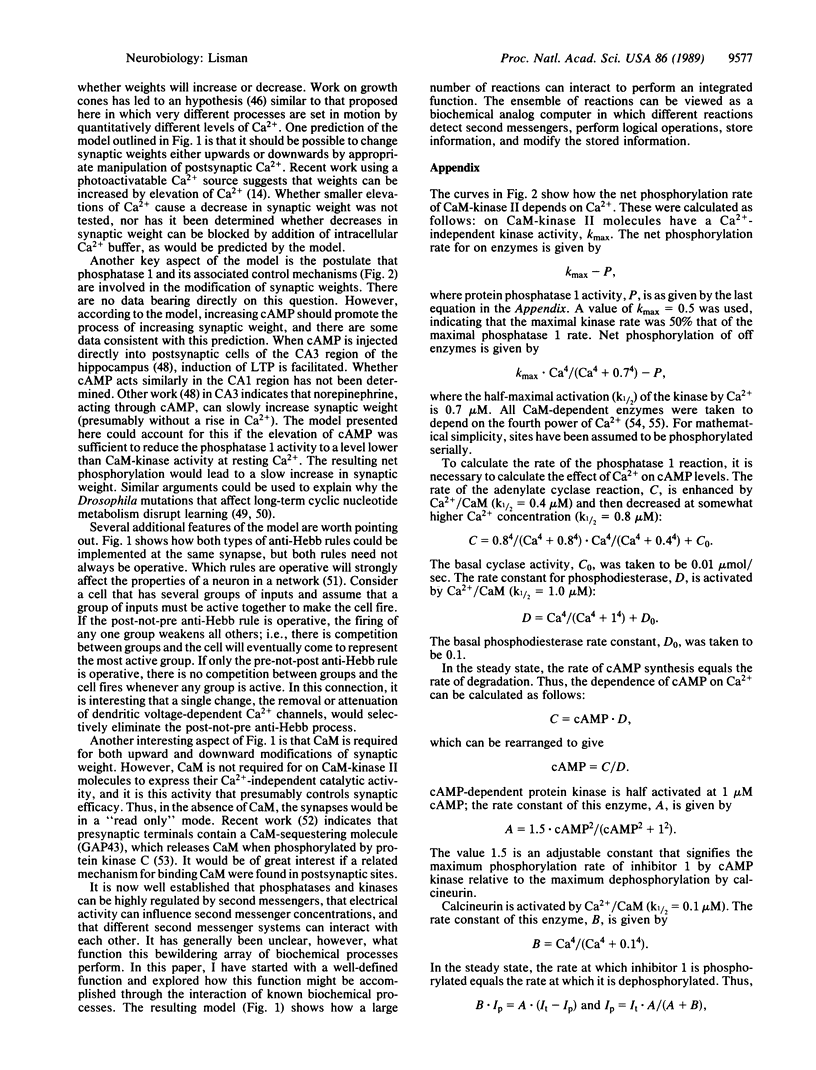
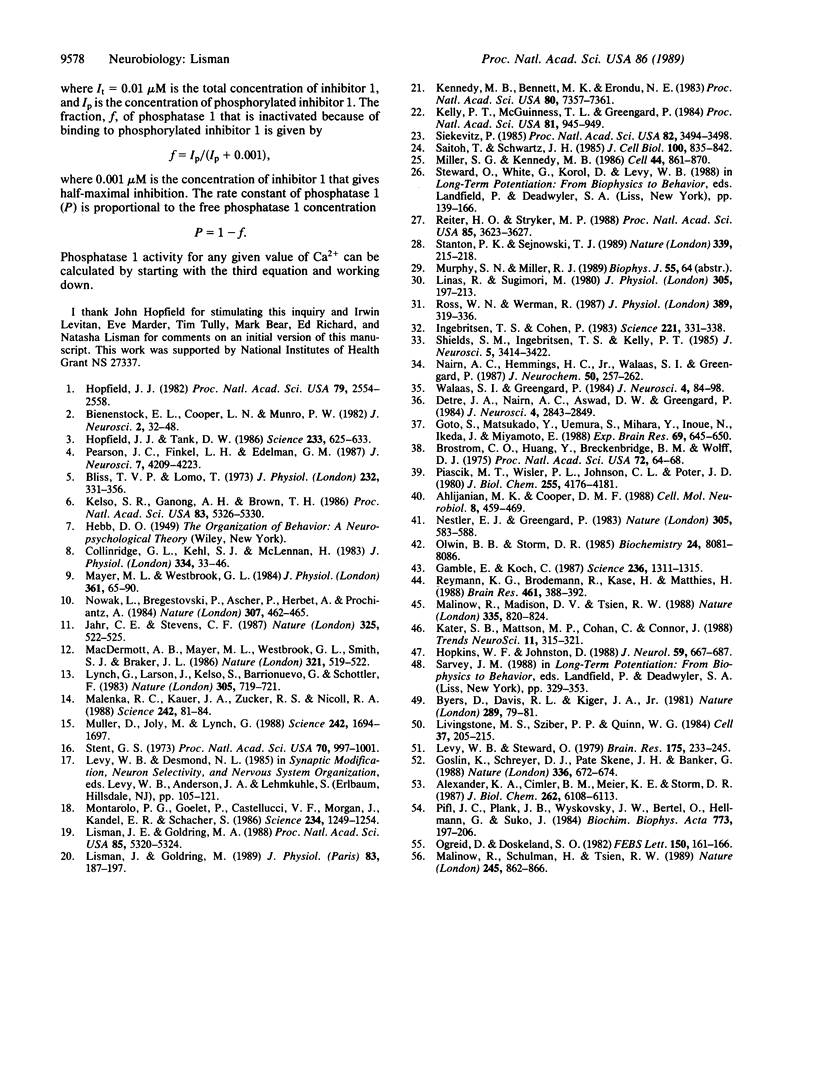
Selected References
These references are in PubMed. This may not be the complete list of references from this article.
- Ahlijanian M. K., Cooper D. M. Distinct interactions between Ca2+/calmodulin and neurotransmitter stimulation of adenylate cyclase in striatum and hippocampus. Cell Mol Neurobiol. 1988 Dec;8(4):459–469. doi: 10.1007/BF00711229. [DOI] [PubMed] [Google Scholar]
- Alexander K. A., Cimler B. M., Meier K. E., Storm D. R. Regulation of calmodulin binding to P-57. A neurospecific calmodulin binding protein. J Biol Chem. 1987 May 5;262(13):6108–6113. [PubMed] [Google Scholar]
- Bienenstock E. L., Cooper L. N., Munro P. W. Theory for the development of neuron selectivity: orientation specificity and binocular interaction in visual cortex. J Neurosci. 1982 Jan;2(1):32–48. doi: 10.1523/JNEUROSCI.02-01-00032.1982. [DOI] [PMC free article] [PubMed] [Google Scholar]
- Bliss T. V., Lomo T. Long-lasting potentiation of synaptic transmission in the dentate area of the anaesthetized rabbit following stimulation of the perforant path. J Physiol. 1973 Jul;232(2):331–356. doi: 10.1113/jphysiol.1973.sp010273. [DOI] [PMC free article] [PubMed] [Google Scholar]
- Brostrom C. O., Huang Y. C., Breckenridge B. M., Wolff D. J. Identification of a calcium-binding protein as a calcium-dependent regulator of brain adenylate cyclase. Proc Natl Acad Sci U S A. 1975 Jan;72(1):64–68. doi: 10.1073/pnas.72.1.64. [DOI] [PMC free article] [PubMed] [Google Scholar]
- Byers D., Davis R. L., Kiger J. A., Jr Defect in cyclic AMP phosphodiesterase due to the dunce mutation of learning in Drosophila melanogaster. Nature. 1981 Jan 1;289(5793):79–81. doi: 10.1038/289079a0. [DOI] [PubMed] [Google Scholar]
- Collingridge G. L., Kehl S. J., McLennan H. Excitatory amino acids in synaptic transmission in the Schaffer collateral-commissural pathway of the rat hippocampus. J Physiol. 1983 Jan;334:33–46. doi: 10.1113/jphysiol.1983.sp014478. [DOI] [PMC free article] [PubMed] [Google Scholar]
- Detre J. A., Nairn A. C., Aswad D. W., Greengard P. Localization in mammalian brain of G-substrate, a specific substrate for guanosine 3',5'-cyclic monophosphate-dependent protein kinase. J Neurosci. 1984 Nov;4(11):2843–2849. doi: 10.1523/JNEUROSCI.04-11-02843.1984. [DOI] [PMC free article] [PubMed] [Google Scholar]
- Gamble E., Koch C. The dynamics of free calcium in dendritic spines in response to repetitive synaptic input. Science. 1987 Jun 5;236(4806):1311–1315. doi: 10.1126/science.3495885. [DOI] [PubMed] [Google Scholar]
- Goslin K., Schreyer D. J., Skene J. H., Banker G. Development of neuronal polarity: GAP-43 distinguishes axonal from dendritic growth cones. Nature. 1988 Dec 15;336(6200):672–674. doi: 10.1038/336672a0. [DOI] [PubMed] [Google Scholar]
- Goto S., Matsukado Y., Uemura S., Mihara Y., Inoue N., Ikeda J., Miyamoto E. A comparative immunohistochemical study of calcineurin and S-100 protein in mammalian and avian brains. Exp Brain Res. 1988;69(3):645–650. doi: 10.1007/BF00247316. [DOI] [PubMed] [Google Scholar]
- Hopfield J. J. Neural networks and physical systems with emergent collective computational abilities. Proc Natl Acad Sci U S A. 1982 Apr;79(8):2554–2558. doi: 10.1073/pnas.79.8.2554. [DOI] [PMC free article] [PubMed] [Google Scholar]
- Hopfield J. J., Tank D. W. Computing with neural circuits: a model. Science. 1986 Aug 8;233(4764):625–633. doi: 10.1126/science.3755256. [DOI] [PubMed] [Google Scholar]
- Hopkins W. F., Johnston D. Noradrenergic enhancement of long-term potentiation at mossy fiber synapses in the hippocampus. J Neurophysiol. 1988 Feb;59(2):667–687. doi: 10.1152/jn.1988.59.2.667. [DOI] [PubMed] [Google Scholar]
- Ingebritsen T. S., Cohen P. Protein phosphatases: properties and role in cellular regulation. Science. 1983 Jul 22;221(4608):331–338. doi: 10.1126/science.6306765. [DOI] [PubMed] [Google Scholar]
- Jahr C. E., Stevens C. F. Glutamate activates multiple single channel conductances in hippocampal neurons. Nature. 1987 Feb 5;325(6104):522–525. doi: 10.1038/325522a0. [DOI] [PubMed] [Google Scholar]
- Kater S. B., Mattson M. P., Cohan C., Connor J. Calcium regulation of the neuronal growth cone. Trends Neurosci. 1988 Jul;11(7):315–321. doi: 10.1016/0166-2236(88)90094-x. [DOI] [PubMed] [Google Scholar]
- Kelly P. T., McGuinness T. L., Greengard P. Evidence that the major postsynaptic density protein is a component of a Ca2+/calmodulin-dependent protein kinase. Proc Natl Acad Sci U S A. 1984 Feb;81(3):945–949. doi: 10.1073/pnas.81.3.945. [DOI] [PMC free article] [PubMed] [Google Scholar]
- Kelso S. R., Ganong A. H., Brown T. H. Hebbian synapses in hippocampus. Proc Natl Acad Sci U S A. 1986 Jul;83(14):5326–5330. doi: 10.1073/pnas.83.14.5326. [DOI] [PMC free article] [PubMed] [Google Scholar]
- Kennedy M. B., Bennett M. K., Erondu N. E. Biochemical and immunochemical evidence that the "major postsynaptic density protein" is a subunit of a calmodulin-dependent protein kinase. Proc Natl Acad Sci U S A. 1983 Dec;80(23):7357–7361. doi: 10.1073/pnas.80.23.7357. [DOI] [PMC free article] [PubMed] [Google Scholar]
- Levy W. B., Steward O. Synapses as associative memory elements in the hippocampal formation. Brain Res. 1979 Oct 19;175(2):233–245. doi: 10.1016/0006-8993(79)91003-5. [DOI] [PubMed] [Google Scholar]
- Lisman J. E., Goldring M. A. Feasibility of long-term storage of graded information by the Ca2+/calmodulin-dependent protein kinase molecules of the postsynaptic density. Proc Natl Acad Sci U S A. 1988 Jul;85(14):5320–5324. doi: 10.1073/pnas.85.14.5320. [DOI] [PMC free article] [PubMed] [Google Scholar]
- Lisman J., Goldring M. Evaluation of a model of long-term memory based on the properties of the Ca2+/calmodulin-dependent protein kinase. J Physiol (Paris) 1988;83(3):187–197. [PubMed] [Google Scholar]
- Livingstone M. S., Sziber P. P., Quinn W. G. Loss of calcium/calmodulin responsiveness in adenylate cyclase of rutabaga, a Drosophila learning mutant. Cell. 1984 May;37(1):205–215. doi: 10.1016/0092-8674(84)90316-7. [DOI] [PubMed] [Google Scholar]
- Llinás R., Sugimori M. Electrophysiological properties of in vitro Purkinje cell dendrites in mammalian cerebellar slices. J Physiol. 1980 Aug;305:197–213. doi: 10.1113/jphysiol.1980.sp013358. [DOI] [PMC free article] [PubMed] [Google Scholar]
- Lynch G., Larson J., Kelso S., Barrionuevo G., Schottler F. Intracellular injections of EGTA block induction of hippocampal long-term potentiation. Nature. 1983 Oct 20;305(5936):719–721. doi: 10.1038/305719a0. [DOI] [PubMed] [Google Scholar]
- MacDermott A. B., Mayer M. L., Westbrook G. L., Smith S. J., Barker J. L. NMDA-receptor activation increases cytoplasmic calcium concentration in cultured spinal cord neurones. 1986 May 29-Jun 4Nature. 321(6069):519–522. doi: 10.1038/321519a0. [DOI] [PubMed] [Google Scholar]
- Malenka R. C., Kauer J. A., Zucker R. S., Nicoll R. A. Postsynaptic calcium is sufficient for potentiation of hippocampal synaptic transmission. Science. 1988 Oct 7;242(4875):81–84. doi: 10.1126/science.2845577. [DOI] [PubMed] [Google Scholar]
- Malinow R., Madison D. V., Tsien R. W. Persistent protein kinase activity underlying long-term potentiation. Nature. 1988 Oct 27;335(6193):820–824. doi: 10.1038/335820a0. [DOI] [PubMed] [Google Scholar]
- Malinow R., Schulman H., Tsien R. W. Inhibition of postsynaptic PKC or CaMKII blocks induction but not expression of LTP. Science. 1989 Aug 25;245(4920):862–866. doi: 10.1126/science.2549638. [DOI] [PubMed] [Google Scholar]
- Mayer M. L., Westbrook G. L. The action of N-methyl-D-aspartic acid on mouse spinal neurones in culture. J Physiol. 1985 Apr;361:65–90. doi: 10.1113/jphysiol.1985.sp015633. [DOI] [PMC free article] [PubMed] [Google Scholar]
- Miller S. G., Kennedy M. B. Regulation of brain type II Ca2+/calmodulin-dependent protein kinase by autophosphorylation: a Ca2+-triggered molecular switch. Cell. 1986 Mar 28;44(6):861–870. doi: 10.1016/0092-8674(86)90008-5. [DOI] [PubMed] [Google Scholar]
- Montarolo P. G., Goelet P., Castellucci V. F., Morgan J., Kandel E. R., Schacher S. A critical period for macromolecular synthesis in long-term heterosynaptic facilitation in Aplysia. Science. 1986 Dec 5;234(4781):1249–1254. doi: 10.1126/science.3775383. [DOI] [PubMed] [Google Scholar]
- Muller D., Joly M., Lynch G. Contributions of quisqualate and NMDA receptors to the induction and expression of LTP. Science. 1988 Dec 23;242(4886):1694–1697. doi: 10.1126/science.2904701. [DOI] [PubMed] [Google Scholar]
- Nairn A. C., Hemmings H. C., Jr, Walaas S. I., Greengard P. DARPP-32 and phosphatase inhibitor-1, two structurally related inhibitors of protein phosphatase-1, are both present in striatonigral neurons. J Neurochem. 1988 Jan;50(1):257–262. doi: 10.1111/j.1471-4159.1988.tb13258.x. [DOI] [PubMed] [Google Scholar]
- Nestler E. J., Greengard P. Protein phosphorylation in the brain. Nature. 1983 Oct 13;305(5935):583–588. doi: 10.1038/305583a0. [DOI] [PubMed] [Google Scholar]
- Nowak L., Bregestovski P., Ascher P., Herbet A., Prochiantz A. Magnesium gates glutamate-activated channels in mouse central neurones. Nature. 1984 Feb 2;307(5950):462–465. doi: 10.1038/307462a0. [DOI] [PubMed] [Google Scholar]
- Ogreid D., Døskeland S. O. Activation of protein kinase isoenzymes under near physiological conditions. Evidence that both types (A and B) of cAMP binding sites are involved in the activation of protein kinase by cAMP and 8-N3-cAMP. FEBS Lett. 1982 Dec 13;150(1):161–166. doi: 10.1016/0014-5793(82)81326-4. [DOI] [PubMed] [Google Scholar]
- Olwin B. B., Storm D. R. Calcium binding to complexes of calmodulin and calmodulin binding proteins. Biochemistry. 1985 Dec 31;24(27):8081–8086. doi: 10.1021/bi00348a037. [DOI] [PubMed] [Google Scholar]
- Pearson J. C., Finkel L. H., Edelman G. M. Plasticity in the organization of adult cerebral cortical maps: a computer simulation based on neuronal group selection. J Neurosci. 1987 Dec;7(12):4209–4223. doi: 10.1523/JNEUROSCI.07-12-04209.1987. [DOI] [PMC free article] [PubMed] [Google Scholar]
- Piascik M. T., Wisler P. L., Johnson C. L., Potter J. D. Ca2+-dependent regulation of guinea pig brain adenylate cyclase. J Biol Chem. 1980 May 10;255(9):4176–4181. [PubMed] [Google Scholar]
- Pifl C., Plank B., Wyskovsky W., Bertel O., Hellmann G., Suko J. Calmodulin X (Ca2+)4 is the active calmodulin-calcium species activating the calcium-, calmodulin-dependent protein kinase of cardiac sarcoplasmic reticulum in the regulation of the calcium pump. Biochim Biophys Acta. 1984 Jun 27;773(2):197–206. doi: 10.1016/0005-2736(84)90083-x. [DOI] [PubMed] [Google Scholar]
- Reiter H. O., Stryker M. P. Neural plasticity without postsynaptic action potentials: less-active inputs become dominant when kitten visual cortical cells are pharmacologically inhibited. Proc Natl Acad Sci U S A. 1988 May;85(10):3623–3627. doi: 10.1073/pnas.85.10.3623. [DOI] [PMC free article] [PubMed] [Google Scholar]
- Reymann K. G., Brödemann R., Kase H., Matthies H. Inhibitors of calmodulin and protein kinase C block different phases of hippocampal long-term potentiation. Brain Res. 1988 Oct 4;461(2):388–392. doi: 10.1016/0006-8993(88)90274-0. [DOI] [PubMed] [Google Scholar]
- Ross W. N., Werman R. Mapping calcium transients in the dendrites of Purkinje cells from the guinea-pig cerebellum in vitro. J Physiol. 1987 Aug;389:319–336. doi: 10.1113/jphysiol.1987.sp016659. [DOI] [PMC free article] [PubMed] [Google Scholar]
- Saitoh T., Schwartz J. H. Phosphorylation-dependent subcellular translocation of a Ca2+/calmodulin-dependent protein kinase produces an autonomous enzyme in Aplysia neurons. J Cell Biol. 1985 Mar;100(3):835–842. doi: 10.1083/jcb.100.3.835. [DOI] [PMC free article] [PubMed] [Google Scholar]
- Shields S. M., Ingebritsen T. S., Kelly P. T. Identification of protein phosphatase 1 in synaptic junctions: dephosphorylation of endogenous calmodulin-dependent kinase II and synapse-enriched phosphoproteins. J Neurosci. 1985 Dec;5(12):3414–3422. doi: 10.1523/JNEUROSCI.05-12-03414.1985. [DOI] [PMC free article] [PubMed] [Google Scholar]
- Siekevitz P. The postsynaptic density: a possible role in long-lasting effects in the central nervous system. Proc Natl Acad Sci U S A. 1985 May;82(10):3494–3498. doi: 10.1073/pnas.82.10.3494. [DOI] [PMC free article] [PubMed] [Google Scholar]
- Stanton P. K., Sejnowski T. J. Associative long-term depression in the hippocampus induced by hebbian covariance. Nature. 1989 May 18;339(6221):215–218. doi: 10.1038/339215a0. [DOI] [PubMed] [Google Scholar]
- Stent G. S. A physiological mechanism for Hebb's postulate of learning. Proc Natl Acad Sci U S A. 1973 Apr;70(4):997–1001. doi: 10.1073/pnas.70.4.997. [DOI] [PMC free article] [PubMed] [Google Scholar]
- Walaas S. I., Greengard P. DARPP-32, a dopamine- and adenosine 3':5'-monophosphate-regulated phosphoprotein enriched in dopamine-innervated brain regions. I. Regional and cellular distribution in the rat brain. J Neurosci. 1984 Jan;4(1):84–98. doi: 10.1523/JNEUROSCI.04-01-00084.1984. [DOI] [PMC free article] [PubMed] [Google Scholar]