Abstract
Site-directed and monoclonal antibodies recognizing different extracellular regions of the RII sodium channel alpha subunit have been used to determine the sequences that comprise the receptor for alpha-scorpion toxins by evaluating the effect of antibody on voltage-dependent binding of radio-labeled toxin isolated from Leiurus quinquestriatus to both reconstituted rat brain sodium channel and rat brain synaptosomes. Of six antibodies tested, two recognizing amino acid residues 355-371 and 382-400 located on an extracellular loop between transmembrane segments S5 and S6 of domain I and one recognizing residues 1686-1703 of a similar loop of domain IV inhibit binding by 30-55%. Inhibition is concentration-(EC50 = 0.4-2 microM) and time- (t1/2 = 40-80 min) dependent. Five different monoclonal antibodies recognizing the same extracellular loop in domain I inhibit binding completely with similar EC50 values as observed for site-directed antibodies. Kinetic studies of the antibody effect are consistent with a slowly reversible competition for the toxin receptor site. Our results suggest that the extracellular loops between segments S5 and S6 of domains I and IV comprise at least part of the alpha-scorpion toxin receptor site and support the membrane topology models in which domains I and IV are adjacent in the tertiary structure of the channel protein and six transmembrane sequences are contained in each of the four homologous domains.
Full text
PDF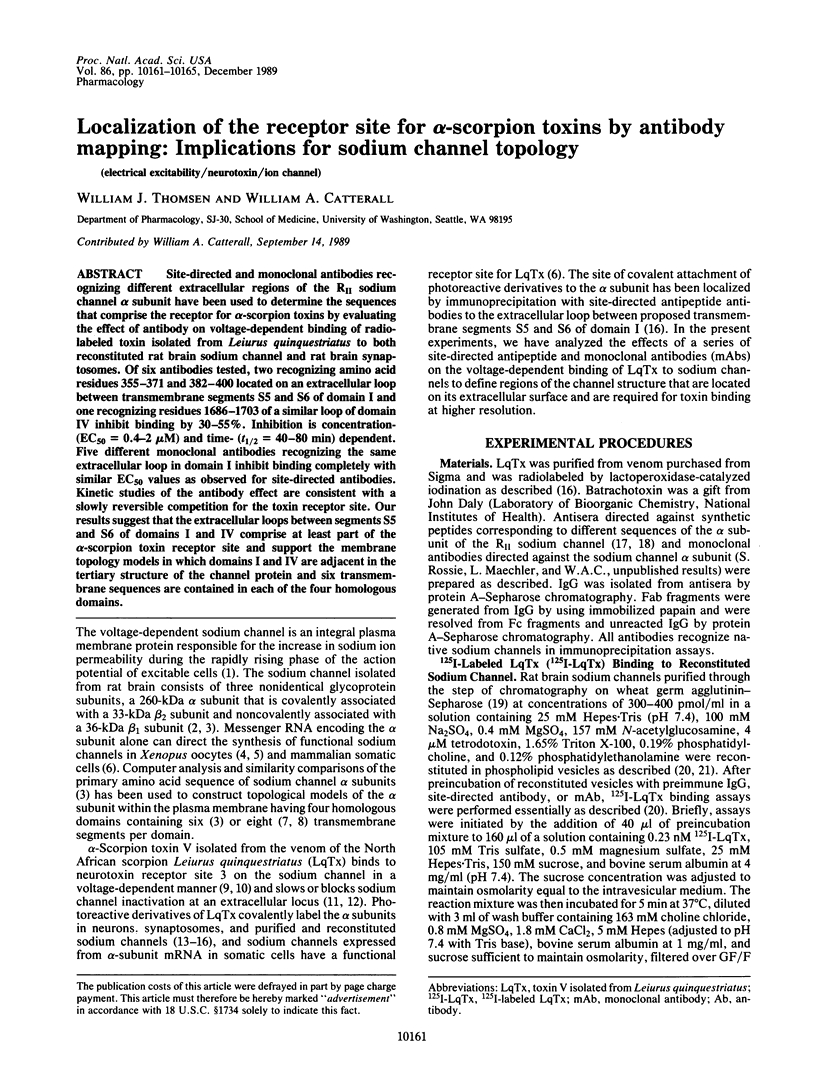
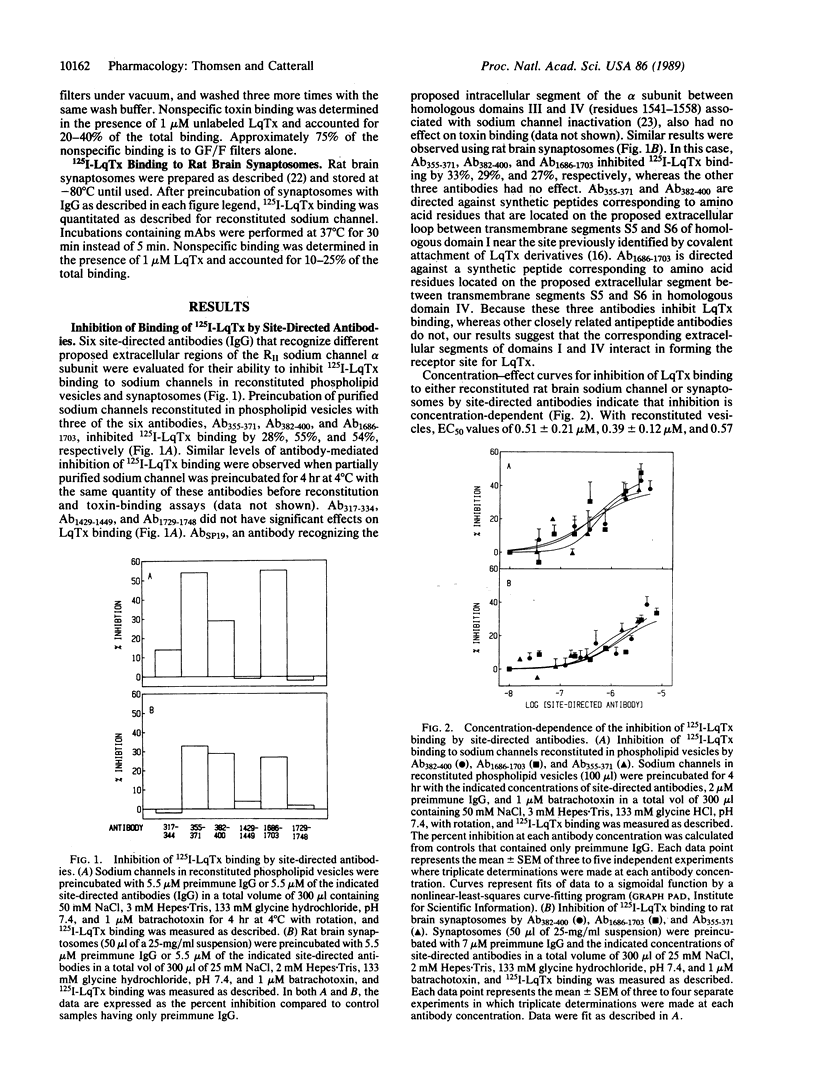
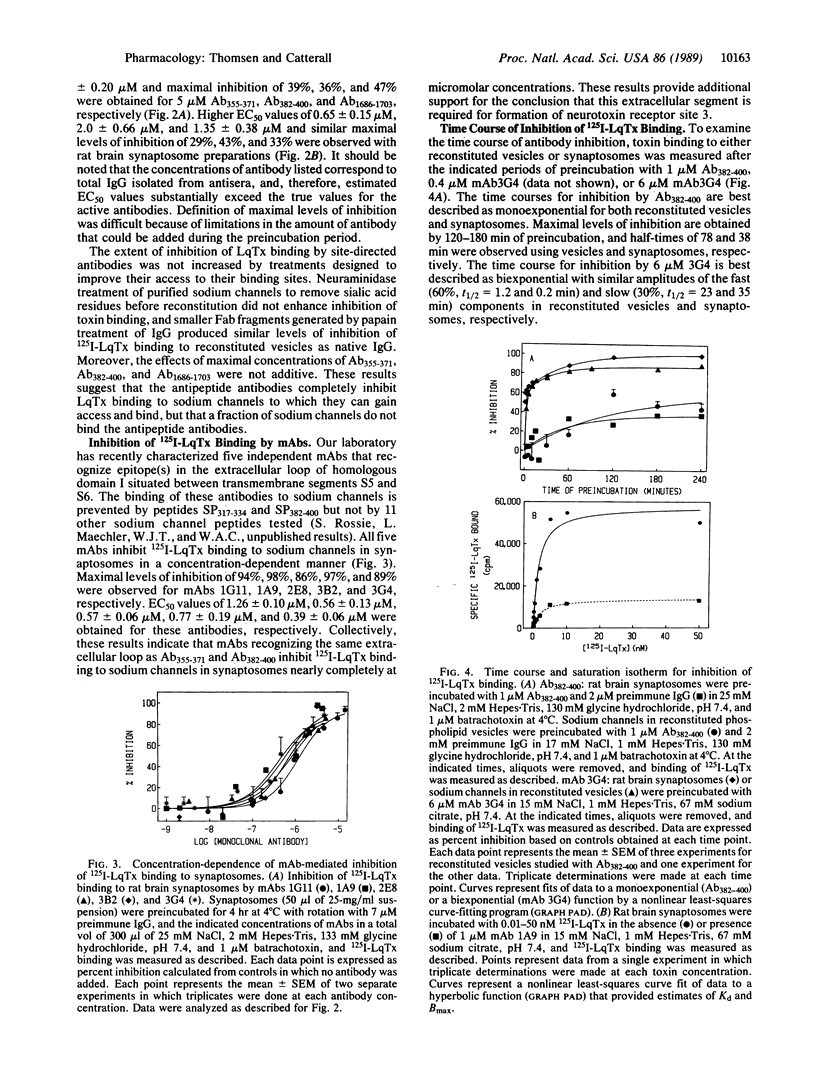
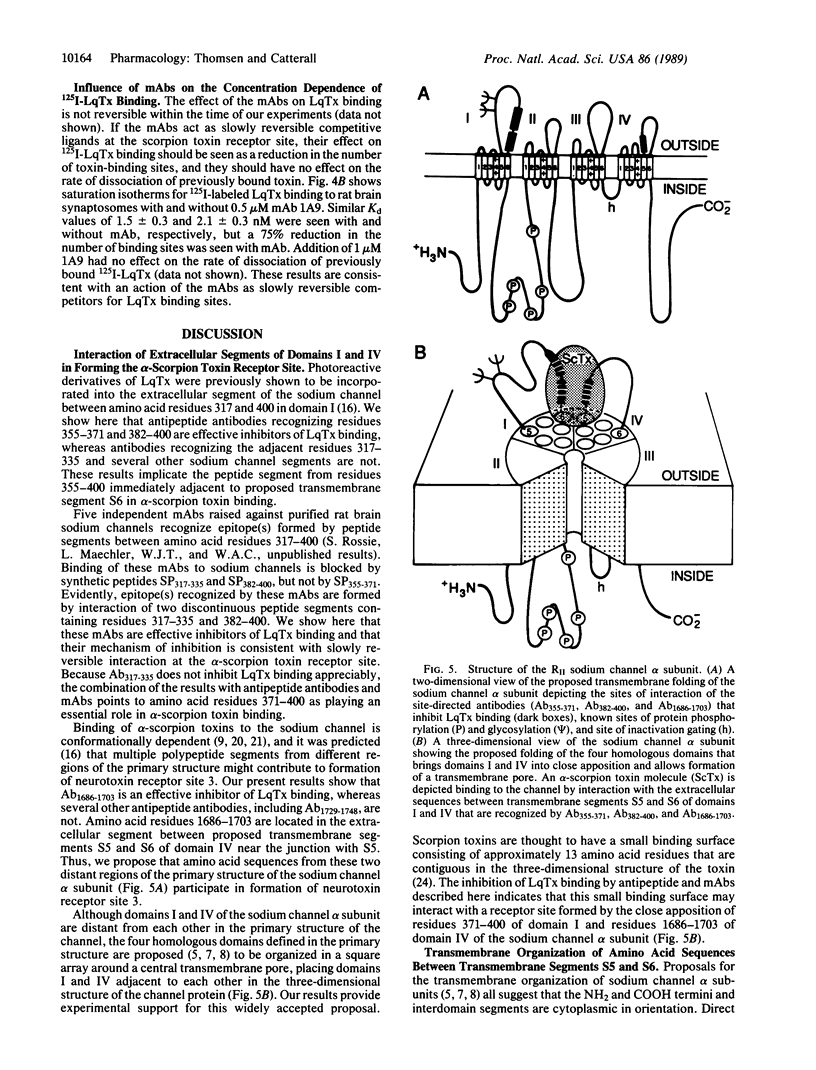
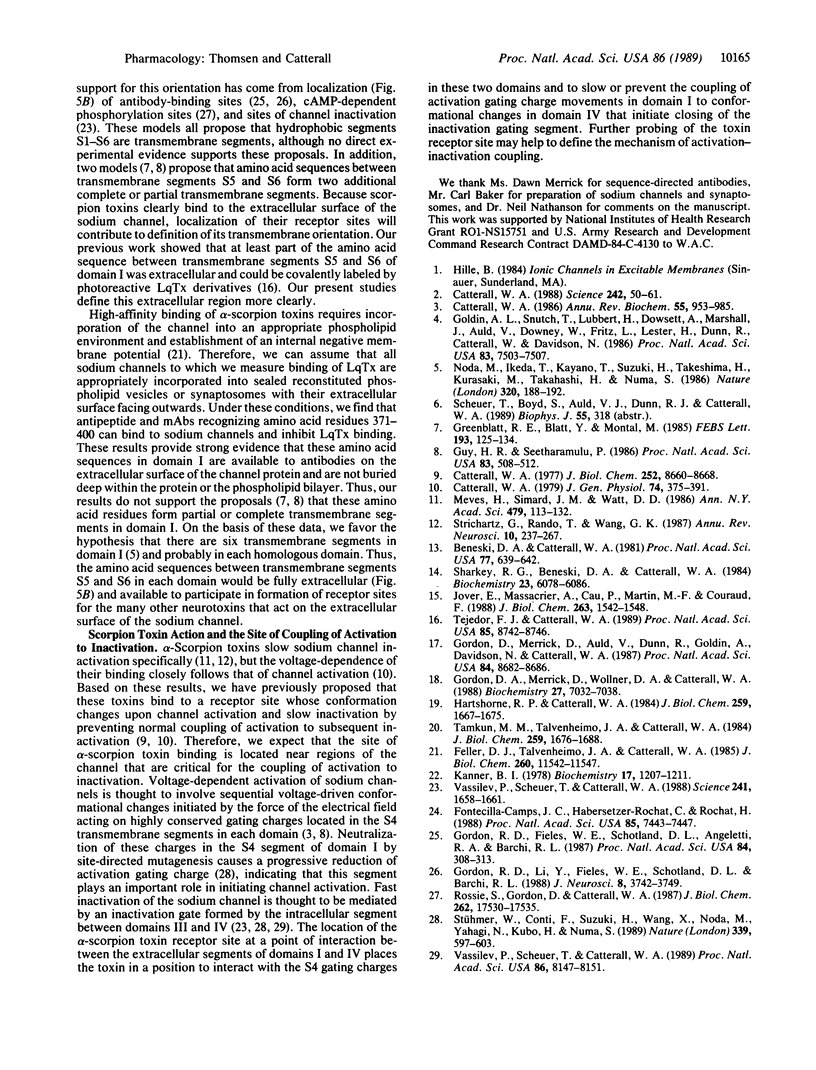
Images in this article
Selected References
These references are in PubMed. This may not be the complete list of references from this article.
- Beneski D. A., Catterall W. A. Covalent labeling of protein components of the sodium channel with a photoactivable derivative of scorpion toxin. Proc Natl Acad Sci U S A. 1980 Jan;77(1):639–643. doi: 10.1073/pnas.77.1.639. [DOI] [PMC free article] [PubMed] [Google Scholar]
- Catterall W. A. Binding of scorpion toxin to receptor sites associated with sodium channels in frog muscle. Correlation of voltage-dependent binding with activation. J Gen Physiol. 1979 Sep;74(3):375–391. doi: 10.1085/jgp.74.3.375. [DOI] [PMC free article] [PubMed] [Google Scholar]
- Catterall W. A. Membrane potential-dependent binding of scorpion toxin to the action potential Na+ ionophore. Studies with a toxin derivative prepared by lactoperoxidase-catalyzed iodination. J Biol Chem. 1977 Dec 10;252(23):8660–8668. [PubMed] [Google Scholar]
- Catterall W. A. Molecular properties of voltage-sensitive sodium channels. Annu Rev Biochem. 1986;55:953–985. doi: 10.1146/annurev.bi.55.070186.004513. [DOI] [PubMed] [Google Scholar]
- Catterall W. A. Structure and function of voltage-sensitive ion channels. Science. 1988 Oct 7;242(4875):50–61. doi: 10.1126/science.2459775. [DOI] [PubMed] [Google Scholar]
- Feller D. J., Talvenheimo J. A., Catterall W. A. The sodium channel from rat brain. Reconstitution of voltage-dependent scorpion toxin binding in vesicles of defined lipid composition. J Biol Chem. 1985 Sep 25;260(21):11542–11547. [PubMed] [Google Scholar]
- Fontecilla-Camps J. C., Habersetzer-Rochat C., Rochat H. Orthorhombic crystals and three-dimensional structure of the potent toxin II from the scorpion Androctonus australis Hector. Proc Natl Acad Sci U S A. 1988 Oct;85(20):7443–7447. doi: 10.1073/pnas.85.20.7443. [DOI] [PMC free article] [PubMed] [Google Scholar]
- Goldin A. L., Snutch T., Lübbert H., Dowsett A., Marshall J., Auld V., Downey W., Fritz L. C., Lester H. A., Dunn R. Messenger RNA coding for only the alpha subunit of the rat brain Na channel is sufficient for expression of functional channels in Xenopus oocytes. Proc Natl Acad Sci U S A. 1986 Oct;83(19):7503–7507. doi: 10.1073/pnas.83.19.7503. [DOI] [PMC free article] [PubMed] [Google Scholar]
- Gordon D., Merrick D., Auld V., Dunn R., Goldin A. L., Davidson N., Catterall W. A. Tissue-specific expression of the RI and RII sodium channel subtypes. Proc Natl Acad Sci U S A. 1987 Dec;84(23):8682–8686. doi: 10.1073/pnas.84.23.8682. [DOI] [PMC free article] [PubMed] [Google Scholar]
- Gordon D., Merrick D., Wollner D. A., Catterall W. A. Biochemical properties of sodium channels in a wide range of excitable tissues studied with site-directed antibodies. Biochemistry. 1988 Sep 6;27(18):7032–7038. doi: 10.1021/bi00418a054. [DOI] [PubMed] [Google Scholar]
- Gordon R. D., Fieles W. E., Schotland D. L., Hogue-Angeletti R., Barchi R. L. Topographical localization of the C-terminal region of the voltage-dependent sodium channel from Electrophorus electricus using antibodies raised against a synthetic peptide. Proc Natl Acad Sci U S A. 1987 Jan;84(1):308–312. doi: 10.1073/pnas.84.1.308. [DOI] [PMC free article] [PubMed] [Google Scholar]
- Gordon R. D., Li Y., Fieles W. E., Schotland D. L., Barchi R. L. Topological localization of a segment of the eel voltage-dependent sodium channel primary sequence (AA 927-938) that discriminates between models of tertiary structure. J Neurosci. 1988 Oct;8(10):3742–3749. doi: 10.1523/JNEUROSCI.08-10-03742.1988. [DOI] [PMC free article] [PubMed] [Google Scholar]
- Greenblatt R. E., Blatt Y., Montal M. The structure of the voltage-sensitive sodium channel. Inferences derived from computer-aided analysis of the Electrophorus electricus channel primary structure. FEBS Lett. 1985 Dec 2;193(2):125–134. doi: 10.1016/0014-5793(85)80136-8. [DOI] [PubMed] [Google Scholar]
- Guy H. R., Seetharamulu P. Molecular model of the action potential sodium channel. Proc Natl Acad Sci U S A. 1986 Jan;83(2):508–512. doi: 10.1073/pnas.83.2.508. [DOI] [PMC free article] [PubMed] [Google Scholar]
- Hartshorne R. P., Catterall W. A. The sodium channel from rat brain. Purification and subunit composition. J Biol Chem. 1984 Feb 10;259(3):1667–1675. [PubMed] [Google Scholar]
- Jover E., Massacrier A., Cau P., Martin M. F., Couraud F. The correlation between Na+ channel subunits and scorpion toxin-binding sites. A study in rat brain synaptosomes and in brain neurons developing in vitro. J Biol Chem. 1988 Jan 25;263(3):1542–1548. [PubMed] [Google Scholar]
- Kanner B. I. Active transport of gamma-aminobutyric acid by membrane vesicles isolated from rat brain. Biochemistry. 1978 Apr 4;17(7):1207–1211. doi: 10.1021/bi00600a011. [DOI] [PubMed] [Google Scholar]
- Meves H., Simard J. M., Watt D. D. Interactions of scorpion toxins with the sodium channel. Ann N Y Acad Sci. 1986;479:113–132. doi: 10.1111/j.1749-6632.1986.tb15565.x. [DOI] [PubMed] [Google Scholar]
- Noda M., Ikeda T., Kayano T., Suzuki H., Takeshima H., Kurasaki M., Takahashi H., Numa S. Existence of distinct sodium channel messenger RNAs in rat brain. Nature. 1986 Mar 13;320(6058):188–192. doi: 10.1038/320188a0. [DOI] [PubMed] [Google Scholar]
- Rossie S., Gordon D., Catterall W. A. Identification of an intracellular domain of the sodium channel having multiple cAMP-dependent phosphorylation sites. J Biol Chem. 1987 Dec 25;262(36):17530–17535. [PubMed] [Google Scholar]
- Sharkey R. G., Beneski D. A., Catterall W. A. Differential labeling of the alpha and beta 1 subunits of the sodium channel by photoreactive derivatives of scorpion toxin. Biochemistry. 1984 Dec 4;23(25):6078–6086. doi: 10.1021/bi00320a027. [DOI] [PubMed] [Google Scholar]
- Strichartz G., Rando T., Wang G. K. An integrated view of the molecular toxinology of sodium channel gating in excitable cells. Annu Rev Neurosci. 1987;10:237–267. doi: 10.1146/annurev.ne.10.030187.001321. [DOI] [PubMed] [Google Scholar]
- Stühmer W., Conti F., Suzuki H., Wang X. D., Noda M., Yahagi N., Kubo H., Numa S. Structural parts involved in activation and inactivation of the sodium channel. Nature. 1989 Jun 22;339(6226):597–603. doi: 10.1038/339597a0. [DOI] [PubMed] [Google Scholar]
- Tamkun M. M., Talvenheimo J. A., Catterall W. A. The sodium channel from rat brain. Reconstitution of neurotoxin-activated ion flux and scorpion toxin binding from purified components. J Biol Chem. 1984 Feb 10;259(3):1676–1688. [PubMed] [Google Scholar]
- Tejedor F. J., Catterall W. A. Site of covalent attachment of alpha-scorpion toxin derivatives in domain I of the sodium channel alpha subunit. Proc Natl Acad Sci U S A. 1988 Nov;85(22):8742–8746. doi: 10.1073/pnas.85.22.8742. [DOI] [PMC free article] [PubMed] [Google Scholar]
- Vassilev P. M., Scheuer T., Catterall W. A. Identification of an intracellular peptide segment involved in sodium channel inactivation. Science. 1988 Sep 23;241(4873):1658–1661. doi: 10.1126/science.241.4873.1658. [DOI] [PubMed] [Google Scholar]
- Vassilev P., Scheuer T., Catterall W. A. Inhibition of inactivation of single sodium channels by a site-directed antibody. Proc Natl Acad Sci U S A. 1989 Oct;86(20):8147–8151. doi: 10.1073/pnas.86.20.8147. [DOI] [PMC free article] [PubMed] [Google Scholar]