Abstract
We have obtained room-temperature transient infrared difference spectra of the M412 photoproduct of bacteriorhodopsin (bR) by using a "rapid-sweep" Fourier-transform infrared (FT-IR) technique that permits the collection of an entire spectrum (extending from 1000 to 2000 cm-1 with 8-cm-1 resolution) in 5 ms. These spectra exhibit less than 10(-4) absorbance unit of noise, even utilizing wet samples containing approximately 10 pmol of bR in the measuring beam. The bR----M transient difference spectrum is similar to FT-IR difference spectra previously obtained under conditions where M decay was blocked (low temperature or low humidity). In particular, the transient spectrum exhibits a set of vibrational difference bands that were previously attributed to protonation changes of several tyrosine residues on the basis of isotopic derivative spectra of M at low temperature. Our rapid-sweep FT-IR spectra demonstrate that these tyrosine/tyrosinate bands are also present under more physiological conditions. Despite the overall similarity to the low-temperature and low-humidity spectra, the room-temperature bR----M transient difference spectrum shows significant additional features in the amide I and amide II regions. These features' presence suggests that a small alteration of the protein backbone accompanies M formation under physiological conditions and that this conformational change is inhibited in the absence of liquid water.
Full text
PDF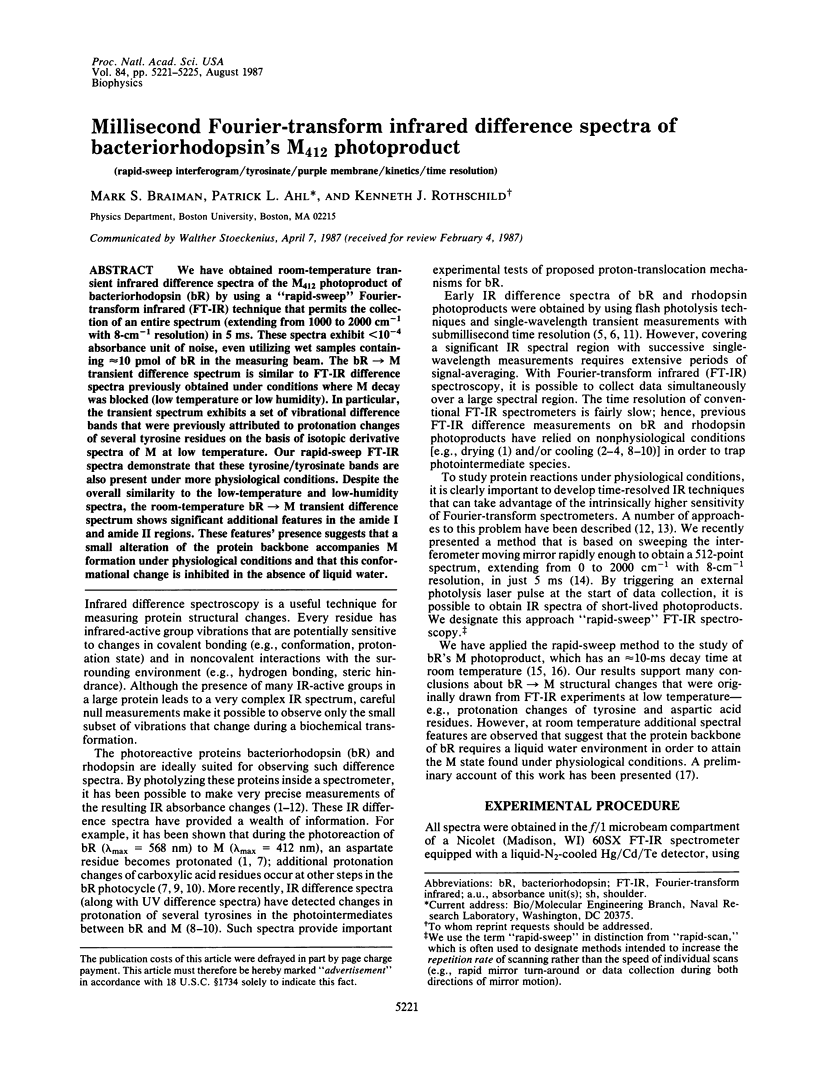
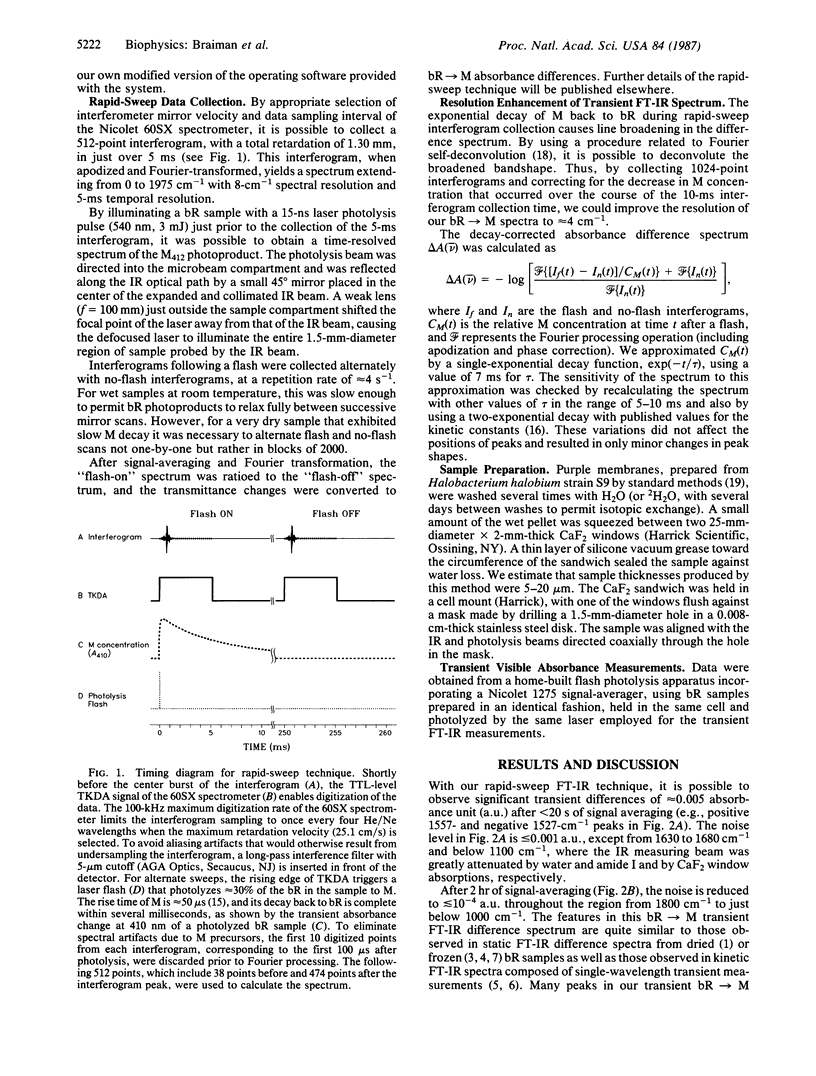
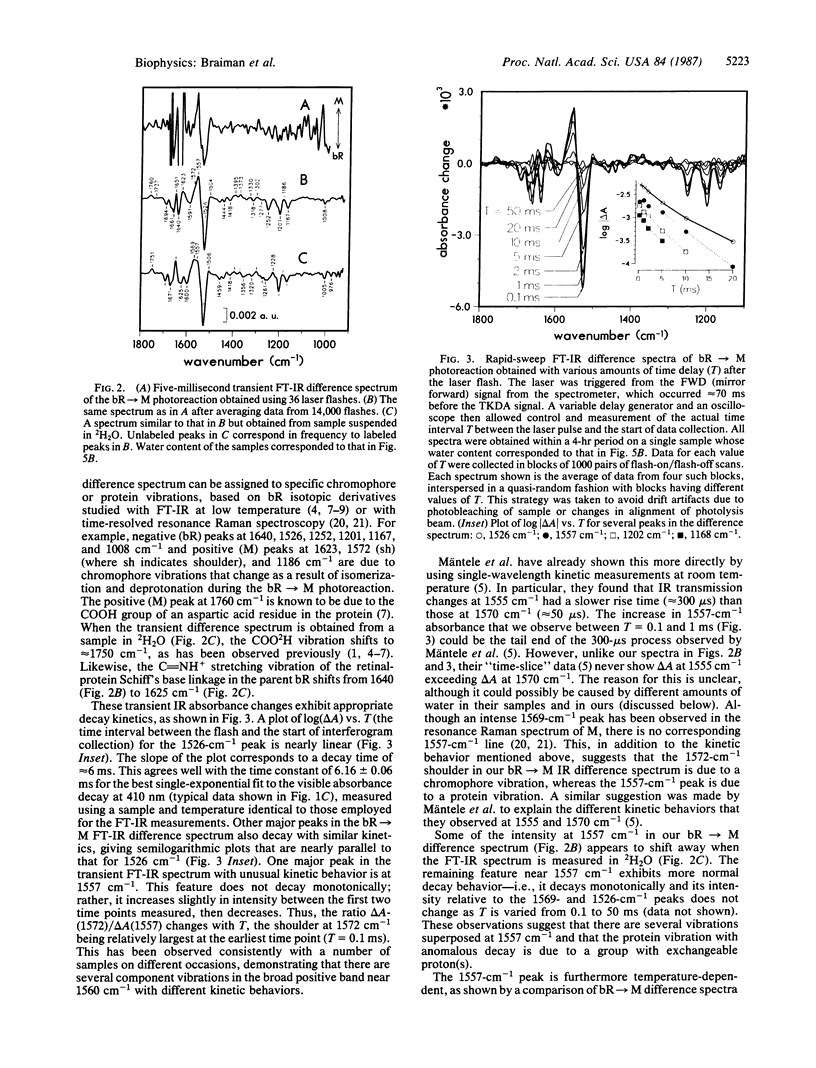
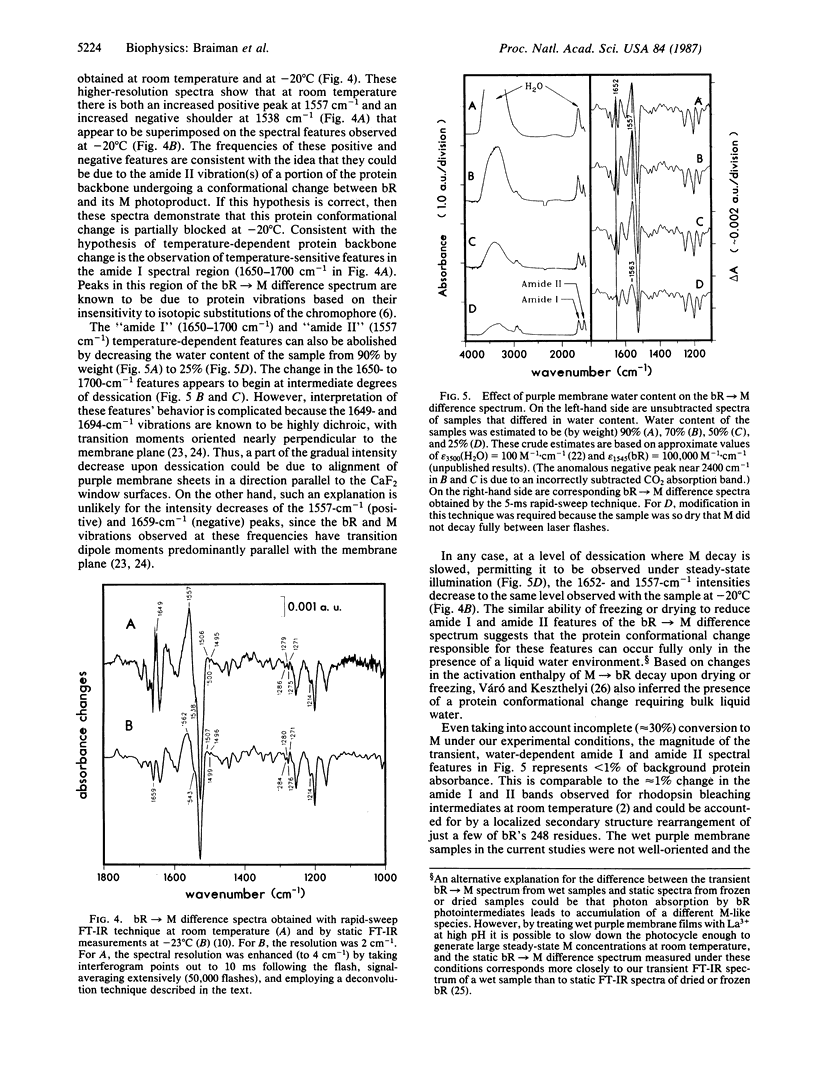
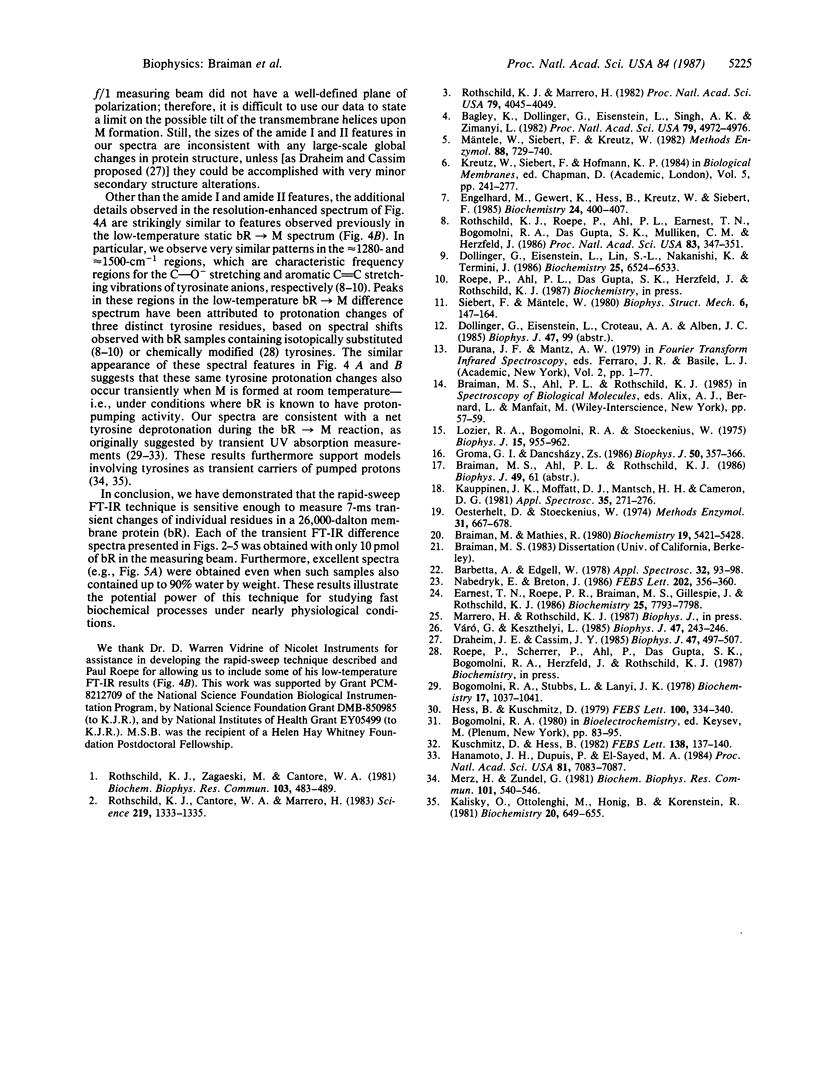
Selected References
These references are in PubMed. This may not be the complete list of references from this article.
- Bagley K., Dollinger G., Eisenstein L., Singh A. K., Zimányi L. Fourier transform infrared difference spectroscopy of bacteriorhodopsin and its photoproducts. Proc Natl Acad Sci U S A. 1982 Aug;79(16):4972–4976. doi: 10.1073/pnas.79.16.4972. [DOI] [PMC free article] [PubMed] [Google Scholar]
- Bogomolni R. A., Stubbs L., Lanyi J. K. Illumination-dependent changes in the intrinsic fluorescence of bacteriorhodopsin. Biochemistry. 1978 Mar 21;17(6):1037–1041. doi: 10.1021/bi00599a015. [DOI] [PubMed] [Google Scholar]
- Braiman M., Mathies R. Resonance Raman evidence for an all-trans to 13-cis isomerization in the proton-pumping cycle of bacteriorhodopsin. Biochemistry. 1980 Nov 11;19(23):5421–5428. doi: 10.1021/bi00564a042. [DOI] [PubMed] [Google Scholar]
- Dollinger G., Eisenstein L., Lin S. L., Nakanishi K., Termini J. Fourier transform infrared difference spectroscopy of bacteriorhodopsin and its photoproducts regenerated with deuterated tyrosine. Biochemistry. 1986 Oct 21;25(21):6524–6533. doi: 10.1021/bi00369a028. [DOI] [PubMed] [Google Scholar]
- Draheim J. E., Cassim J. Y. Large Scale Global Structural Changes of the Purple Membrane during the Photocycle. Biophys J. 1985 Apr;47(4):497–507. doi: 10.1016/S0006-3495(85)83943-6. [DOI] [PMC free article] [PubMed] [Google Scholar]
- Earnest T. N., Roepe P., Braiman M. S., Gillespie J., Rothschild K. J. Orientation of the bacteriorhodopsin chromophore probed by polarized Fourier transform infrared difference spectroscopy. Biochemistry. 1986 Dec 2;25(24):7793–7798. doi: 10.1021/bi00372a002. [DOI] [PubMed] [Google Scholar]
- Engelhard M., Gerwert K., Hess B., Kreutz W., Siebert F. Light-driven protonation changes of internal aspartic acids of bacteriorhodopsin: an investigation by static and time-resolved infrared difference spectroscopy using [4-13C]aspartic acid labeled purple membrane. Biochemistry. 1985 Jan 15;24(2):400–407. doi: 10.1021/bi00323a024. [DOI] [PubMed] [Google Scholar]
- Groma G. I., Dancshazy Z. How Many M Forms are there in the Bacteriorhodopsin Photocycle? Biophys J. 1986 Aug;50(2):357–366. doi: 10.1016/S0006-3495(86)83469-5. [DOI] [PMC free article] [PubMed] [Google Scholar]
- Hanamoto J. H., Dupuis P., El-Sayed M. A. On the protein (tyrosine)-chromophore (protonated Schiff base) coupling in bacteriorhodopsin. Proc Natl Acad Sci U S A. 1984 Nov;81(22):7083–7087. doi: 10.1073/pnas.81.22.7083. [DOI] [PMC free article] [PubMed] [Google Scholar]
- Hess B., Kuschmitz D. Kinetic interaction between aromatic residues and the retinal chromophore of bacteriorhodopsin during the photocycle. FEBS Lett. 1979 Apr 15;100(2):334–340. doi: 10.1016/0014-5793(79)80364-6. [DOI] [PubMed] [Google Scholar]
- Kalisky O., Ottolenghi M., Honig B., Korenstein R. Environmental effects on formation and photoreaction of the M412 photoproduct of bacteriorhodopsin: implications for the mechanism of proton pumping. Biochemistry. 1981 Feb 3;20(3):649–655. doi: 10.1021/bi00506a031. [DOI] [PubMed] [Google Scholar]
- Lozier R. H., Bogomolni R. A., Stoeckenius W. Bacteriorhodopsin: a light-driven proton pump in Halobacterium Halobium. Biophys J. 1975 Sep;15(9):955–962. doi: 10.1016/S0006-3495(75)85875-9. [DOI] [PMC free article] [PubMed] [Google Scholar]
- Merz H., Zundel G. Proton conduction in bacteriorhodopsin via a hydrogen-bonded chain with large proton polarizability. Biochem Biophys Res Commun. 1981 Jul 30;101(2):540–546. doi: 10.1016/0006-291x(81)91293-6. [DOI] [PubMed] [Google Scholar]
- Oesterhelt D., Stoeckenius W. Isolation of the cell membrane of Halobacterium halobium and its fractionation into red and purple membrane. Methods Enzymol. 1974;31:667–678. doi: 10.1016/0076-6879(74)31072-5. [DOI] [PubMed] [Google Scholar]
- Rothschild K. J., Cantore W. A., Marrero H. Fourier transform infrared difference spectra of intermediates in rhodopsin bleaching. Science. 1983 Mar 18;219(4590):1333–1335. doi: 10.1126/science.6828860. [DOI] [PubMed] [Google Scholar]
- Rothschild K. J., Marrero H. Infrared evidence that the Schiff base of bacteriorhodopsin is protonated: bR570 and K intermediates. Proc Natl Acad Sci U S A. 1982 Jul;79(13):4045–4049. doi: 10.1073/pnas.79.13.4045. [DOI] [PMC free article] [PubMed] [Google Scholar]
- Rothschild K. J., Roepe P., Ahl P. L., Earnest T. N., Bogomolni R. A., Das Gupta S. K., Mulliken C. M., Herzfeld J. Evidence for a tyrosine protonation change during the primary phototransition of bacteriorhodopsin at low temperature. Proc Natl Acad Sci U S A. 1986 Jan;83(2):347–351. doi: 10.1073/pnas.83.2.347. [DOI] [PMC free article] [PubMed] [Google Scholar]
- Rothschild K. J., Zagaeski M., Cantore W. A. Conformational changes of bacteriorhodopsin detected by Fourier transform infrared difference spectroscopy. Biochem Biophys Res Commun. 1981 Nov 30;103(2):483–489. doi: 10.1016/0006-291x(81)90478-2. [DOI] [PubMed] [Google Scholar]
- Siebert F., Mäntele W. Investigations of the rhodopsin/Meta I and rhodopsin/Meta II transitions of bovine rod outer segments by means of kinetic infrared spectroscopy. Biophys Struct Mech. 1980;6(2):147–164. doi: 10.1007/BF00535751. [DOI] [PubMed] [Google Scholar]
- Váró G., Keszthelyi L. Arrhenius parameters of the bacteriorhodopsin photocycle in dried oriented samples. Biophys J. 1985 Feb;47(2 Pt 1):243–246. doi: 10.1016/s0006-3495(85)83897-2. [DOI] [PMC free article] [PubMed] [Google Scholar]