Abstract
It is proposed that the stability of a protein can be increased by selected amino acid substitutions that decrease the configurational entropy of unfolding. Two such substitutions, one of the form Xaa----Pro and the other of the form Gly----Xaa, were constructed in bacteriophage T4 lysozyme at sites consistent with the known three-dimensional structure. Both substitutions stabilize the protein toward reversible and irreversible thermal denaturation at physiological pH. The substitutions have no effect on enzymatic activity. High-resolution crystallographic analysis of the proline-containing mutant protein (Ala-82----Pro) shows that its three-dimensional structure is essentially identical with the wild-type enzyme. The overall structure of the other mutant enzyme (Gly-77----Ala) is also very similar to wild-type lysozyme, although there are localized conformational adjustments in the vicinity of the altered amino acid. The combination of a number of such amino acid replacements, each of which is expected to contribute approximately 1 kcal/mol (1 cal = 4.184 J) to the free energy of folding, may provide a general strategy for substantial improvement in the stability of a protein.
Full text
PDF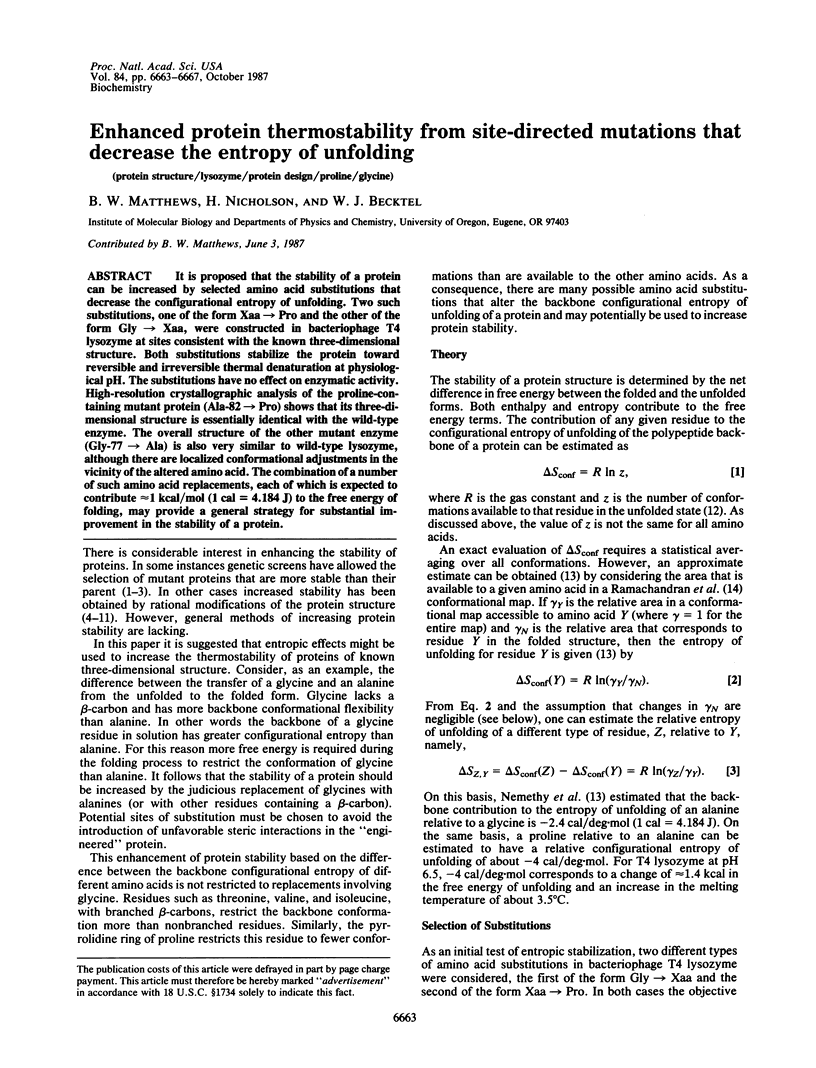
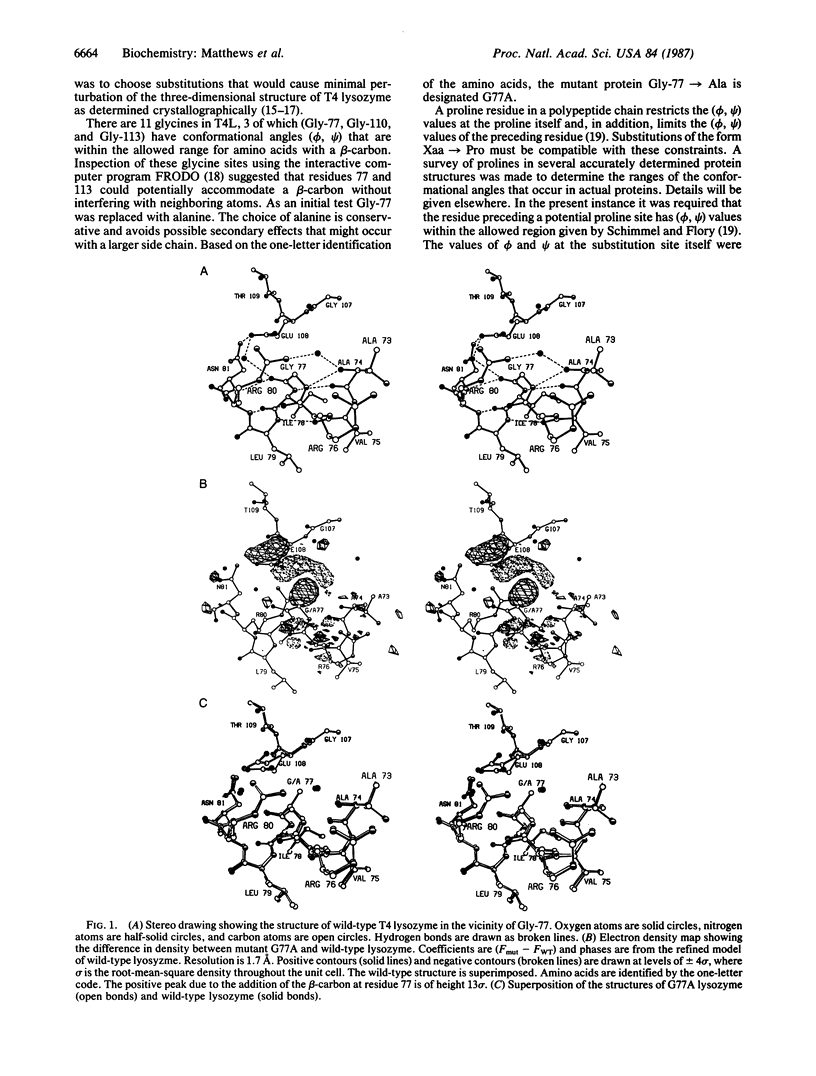
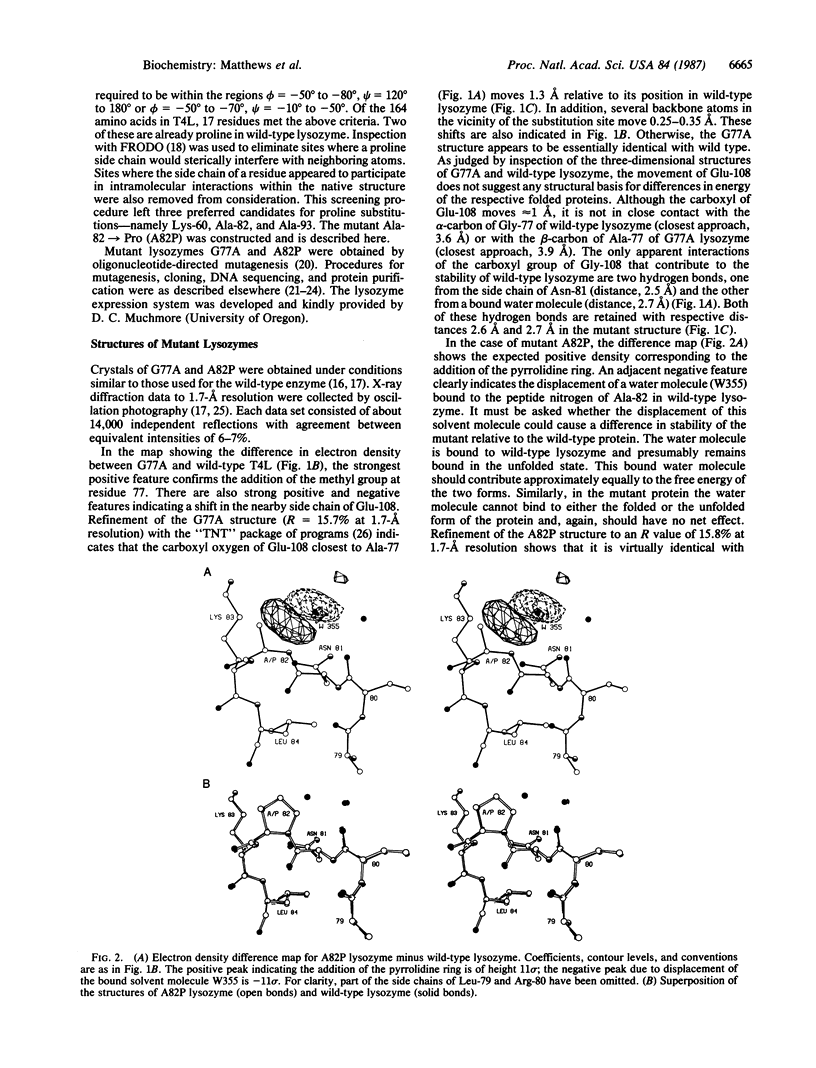
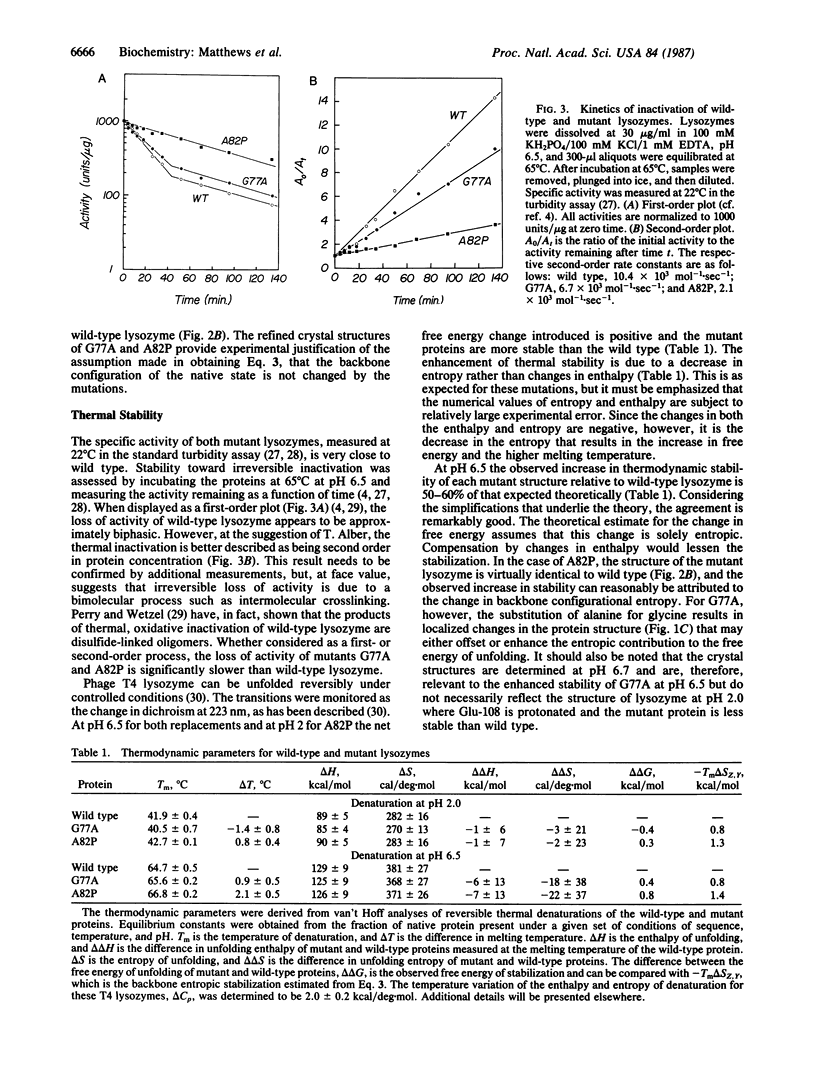
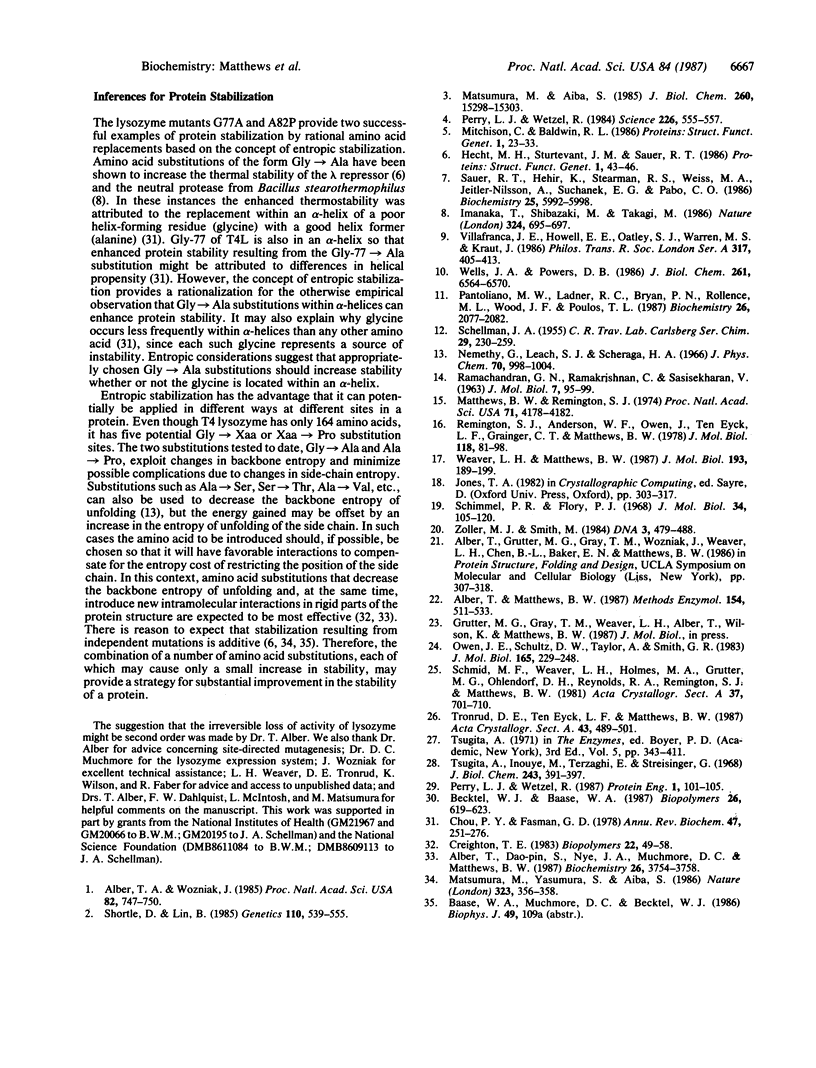
Selected References
These references are in PubMed. This may not be the complete list of references from this article.
- Alber T., Matthews B. W. Structure and thermal stability of phage T4 lysozyme. Methods Enzymol. 1987;154:511–533. doi: 10.1016/0076-6879(87)54093-9. [DOI] [PubMed] [Google Scholar]
- Alber T., Sun D. P., Nye J. A., Muchmore D. C., Matthews B. W. Temperature-sensitive mutations of bacteriophage T4 lysozyme occur at sites with low mobility and low solvent accessibility in the folded protein. Biochemistry. 1987 Jun 30;26(13):3754–3758. doi: 10.1021/bi00387a002. [DOI] [PubMed] [Google Scholar]
- Alber T., Wozniak J. A. A genetic screen for mutations that increase the thermal stability of phage T4 lysozyme. Proc Natl Acad Sci U S A. 1985 Feb;82(3):747–750. doi: 10.1073/pnas.82.3.747. [DOI] [PMC free article] [PubMed] [Google Scholar]
- Becktel W. J., Baase W. A. Thermal denaturation of bacteriophage T4 lysozyme at neutral pH. Biopolymers. 1987 May;26(5):619–623. doi: 10.1002/bip.360260505. [DOI] [PubMed] [Google Scholar]
- Chou P. Y., Fasman G. D. Empirical predictions of protein conformation. Annu Rev Biochem. 1978;47:251–276. doi: 10.1146/annurev.bi.47.070178.001343. [DOI] [PubMed] [Google Scholar]
- Creighton T. E. An empirical approach to protein conformation stability and flexibility. Biopolymers. 1983 Jan;22(1):49–58. doi: 10.1002/bip.360220110. [DOI] [PubMed] [Google Scholar]
- Hecht M. H., Sturtevant J. M., Sauer R. T. Stabilization of lambda repressor against thermal denaturation by site-directed Gly----Ala changes in alpha-helix 3. Proteins. 1986 Sep;1(1):43–46. doi: 10.1002/prot.340010108. [DOI] [PubMed] [Google Scholar]
- Imanaka T., Shibazaki M., Takagi M. A new way of enhancing the thermostability of proteases. Nature. 1986 Dec 18;324(6098):695–697. doi: 10.1038/324695a0. [DOI] [PubMed] [Google Scholar]
- Matsumura M., Aiba S. Screening for thermostable mutant of kanamycin nucleotidyltransferase by the use of a transformation system for a thermophile, Bacillus stearothermophilus. J Biol Chem. 1985 Dec 5;260(28):15298–15303. [PubMed] [Google Scholar]
- Matsumura M., Yasumura S., Aiba S. Cumulative effect of intragenic amino-acid replacements on the thermostability of a protein. 1986 Sep 25-Oct 1Nature. 323(6086):356–358. doi: 10.1038/323356a0. [DOI] [PubMed] [Google Scholar]
- Matthews B. W., Remington S. J. The three dimensional structure of the lysozyme from bacteriophage T4. Proc Natl Acad Sci U S A. 1974 Oct;71(10):4178–4182. doi: 10.1073/pnas.71.10.4178. [DOI] [PMC free article] [PubMed] [Google Scholar]
- Mitchinson C., Baldwin R. L. The design and production of semisynthetic ribonucleases with increased thermostability by incorporation of S-peptide analogues with enhanced helical stability. Proteins. 1986 Sep;1(1):23–33. doi: 10.1002/prot.340010106. [DOI] [PubMed] [Google Scholar]
- Owen J. E., Schultz D. W., Taylor A., Smith G. R. Nucleotide sequence of the lysozyme gene of bacteriophage T4. Analysis of mutations involving repeated sequences. J Mol Biol. 1983 Apr 5;165(2):229–248. doi: 10.1016/s0022-2836(83)80255-1. [DOI] [PubMed] [Google Scholar]
- Pantoliano M. W., Ladner R. C., Bryan P. N., Rollence M. L., Wood J. F., Poulos T. L. Protein engineering of subtilisin BPN': enhanced stabilization through the introduction of two cysteines to form a disulfide bond. Biochemistry. 1987 Apr 21;26(8):2077–2082. doi: 10.1021/bi00382a002. [DOI] [PubMed] [Google Scholar]
- Perry L. J., Wetzel R. Disulfide bond engineered into T4 lysozyme: stabilization of the protein toward thermal inactivation. Science. 1984 Nov 2;226(4674):555–557. doi: 10.1126/science.6387910. [DOI] [PubMed] [Google Scholar]
- Perry L. J., Wetzel R. The role of cysteine oxidation in the thermal inactivation of T4 lysozyme. Protein Eng. 1987 Feb-Mar;1(2):101–105. doi: 10.1093/protein/1.2.101. [DOI] [PubMed] [Google Scholar]
- RAMACHANDRAN G. N., RAMAKRISHNAN C., SASISEKHARAN V. Stereochemistry of polypeptide chain configurations. J Mol Biol. 1963 Jul;7:95–99. doi: 10.1016/s0022-2836(63)80023-6. [DOI] [PubMed] [Google Scholar]
- Remington S. J., Anderson W. F., Owen J., Ten Eyck L. F., Grainger C. T., Matthews B. W. Structure of the lysozyme from bacteriophage T4: an electron density map at 2.4 A resolution. J Mol Biol. 1978 Jan 5;118(1):81–98. doi: 10.1016/0022-2836(78)90245-0. [DOI] [PubMed] [Google Scholar]
- SCHELLMAN J. A. The stability of hydrogen-bonded peptide structures in aqueous solution. C R Trav Lab Carlsberg Chim. 1955;29(14-15):230–259. [PubMed] [Google Scholar]
- Sauer R. T., Hehir K., Stearman R. S., Weiss M. A., Jeitler-Nilsson A., Suchanek E. G., Pabo C. O. An engineered intersubunit disulfide enhances the stability and DNA binding of the N-terminal domain of lambda repressor. Biochemistry. 1986 Oct 7;25(20):5992–5998. doi: 10.1021/bi00368a024. [DOI] [PubMed] [Google Scholar]
- Schimmel P. R., Flory P. J. Conformational energies and configurational statistics of copolypeptides containing L-proline. J Mol Biol. 1968 May 28;34(1):105–120. doi: 10.1016/0022-2836(68)90237-4. [DOI] [PubMed] [Google Scholar]
- Shortle D., Lin B. Genetic analysis of staphylococcal nuclease: identification of three intragenic "global" suppressors of nuclease-minus mutations. Genetics. 1985 Aug;110(4):539–555. doi: 10.1093/genetics/110.4.539. [DOI] [PMC free article] [PubMed] [Google Scholar]
- Tsugita A., Inouye M. Purification of bacteriophage T4 lysozyme. J Biol Chem. 1968 Jan 25;243(2):391–397. [PubMed] [Google Scholar]
- Weaver L. H., Matthews B. W. Structure of bacteriophage T4 lysozyme refined at 1.7 A resolution. J Mol Biol. 1987 Jan 5;193(1):189–199. doi: 10.1016/0022-2836(87)90636-x. [DOI] [PubMed] [Google Scholar]
- Wells J. A., Powers D. B. In vivo formation and stability of engineered disulfide bonds in subtilisin. J Biol Chem. 1986 May 15;261(14):6564–6570. [PubMed] [Google Scholar]
- Zoller M. J., Smith M. Oligonucleotide-directed mutagenesis: a simple method using two oligonucleotide primers and a single-stranded DNA template. DNA. 1984 Dec;3(6):479–488. doi: 10.1089/dna.1.1984.3.479. [DOI] [PubMed] [Google Scholar]