Abstract
Four alanine-based peptides were designed, synthesized, and tested by circular dichroism for alpha-helix formation in H2O. Each peptide has three glutamic/lysine residue pairs, is 16 or 17 amino acids long, and has blocked alpha-NH2 and alpha-COOH groups. In one set of peptides ("i+4"), the glutamic and lysine residues are spaced 4 residues or 1 residue apart. In the other set ("i+3"), the spacing is 3 or 2 residues. Within each of these sets, a pair of peptides was made in which the positions of the glutamic and lysine residues are reversed [Glu, Lys (E,K) vs. Lys, Glu (K,E)] in order to assess the interaction of the charged side chains with the helix dipole. Since the amino acid compositions of these peptides differ at most by a single alanine residue, differences in helicity are caused chiefly by the spacing and positions of the charged residues. The basic aim of this study was to test for helix stabilization by (Glu-, Lys+) ion pairs or salt bridges (H-bonded ion pairs). The results are as follows. (i) All four peptides show significant helix formation, and the stability of the alpha-helix does not depend on peptide concentration in the range studied. The best helix-former is (i+4)E,K, which shows approximately 80% helicity in 0.01 M NaCl at pH 7 and 0 degree C. (ii) The two i+4 peptides show more helix formation than the i+3 peptides. pH titration gives no evidence for helix stabilization by i+3 ion pairs. (iii) Surprisingly, the i+4 peptides form more stable helices than the i+3 peptides at extremes of pH (pH 2 and pH 12) as well as at pH 7. These results may be explained by helix stabilization through Glu-...Lys+ salt bridges at pH 7 and singly charged H bonds at pH 2 (Glu0...Lys+) and pH 12 (Glu-...Lys0). The reason why these links stabilize the alpha-helix more effectively in the i+4 than in the i+3 peptides is not known. (iv) Reversal of the positions of glutamic and lysine residues usually affects helix stability in the manner expected for interaction of these charged groups with the helix dipole. (v) alpha-Helix formation in these alanine-based peptides is enthalpy-driven, as is helix formation by the C-peptide of ribonuclease A.
Full text
PDF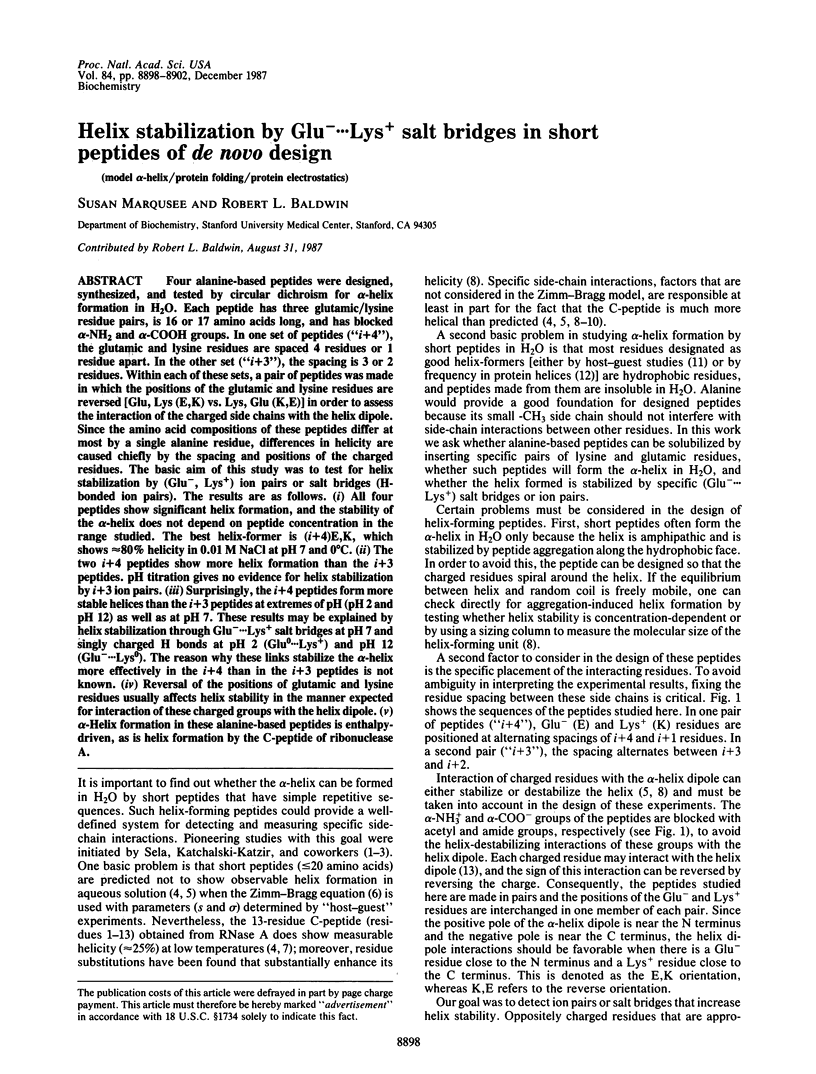
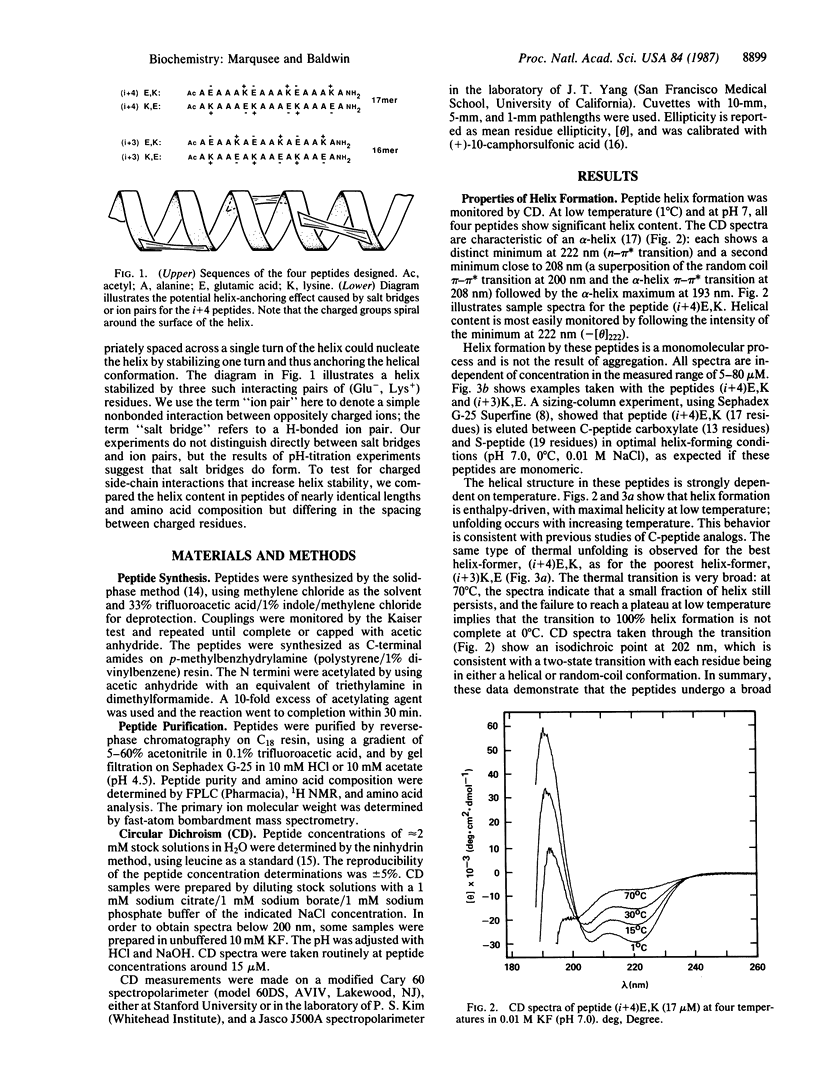
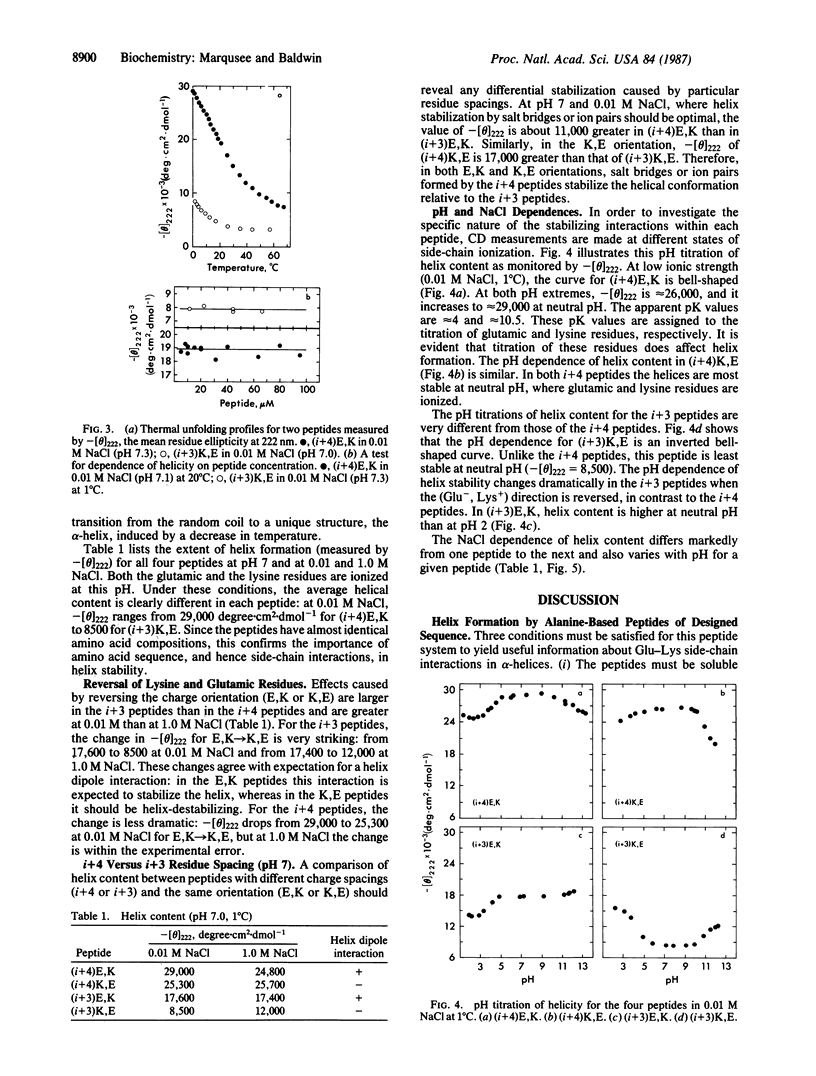
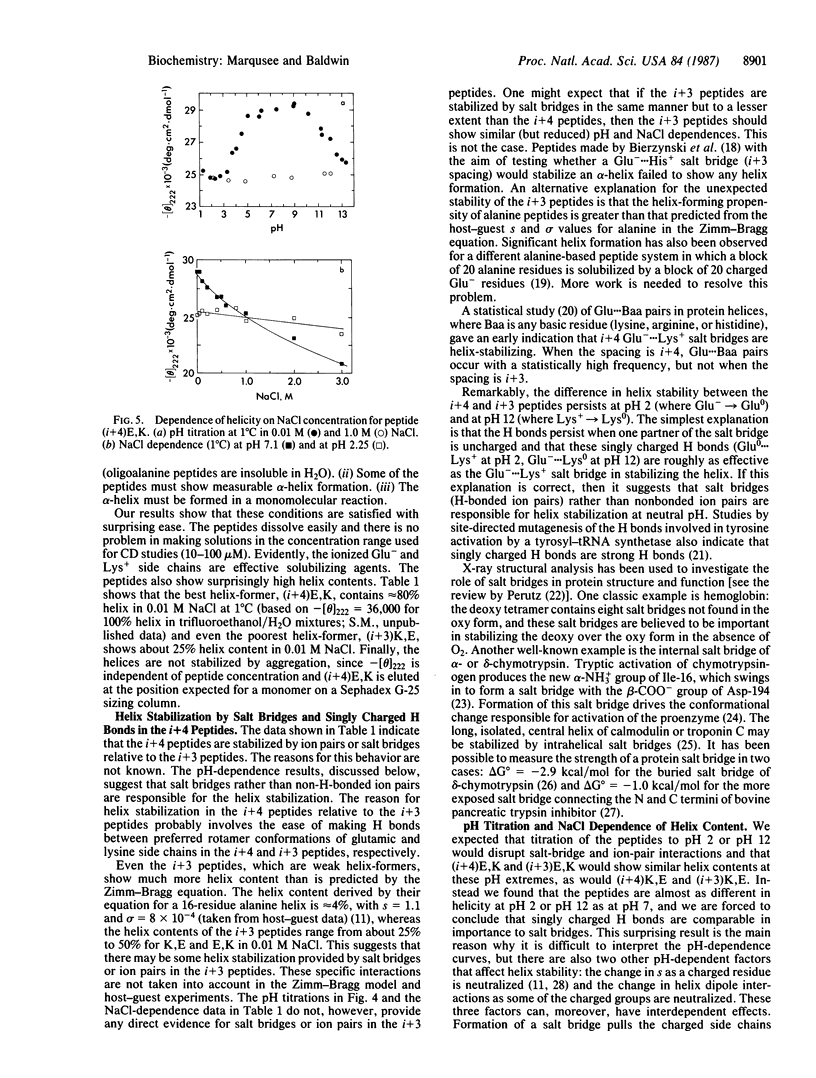
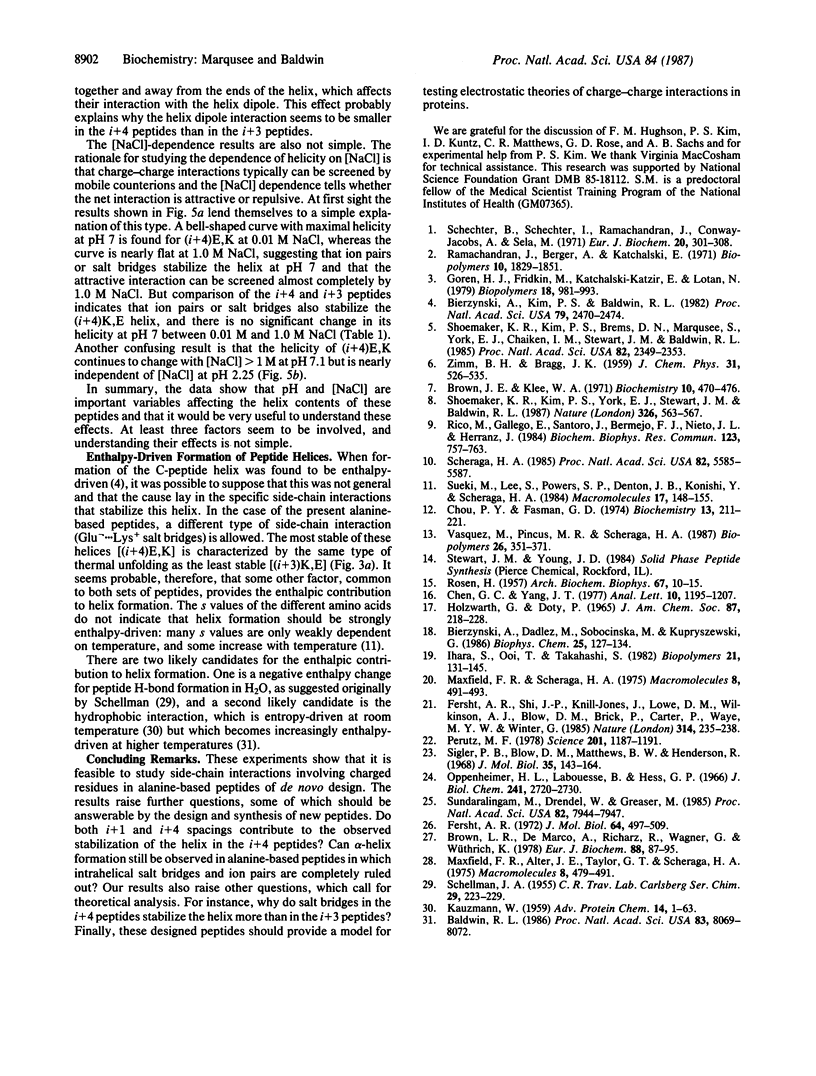
Selected References
These references are in PubMed. This may not be the complete list of references from this article.
- Baldwin R. L. Temperature dependence of the hydrophobic interaction in protein folding. Proc Natl Acad Sci U S A. 1986 Nov;83(21):8069–8072. doi: 10.1073/pnas.83.21.8069. [DOI] [PMC free article] [PubMed] [Google Scholar]
- Bierzynski A., Kim P. S., Baldwin R. L. A salt bridge stabilizes the helix formed by isolated C-peptide of RNase A. Proc Natl Acad Sci U S A. 1982 Apr;79(8):2470–2474. doi: 10.1073/pnas.79.8.2470. [DOI] [PMC free article] [PubMed] [Google Scholar]
- Bierzyński A., Dadlez M., Sobocińska M., Kupryszewski G. Conformational study of two synthetic peptides with sequence analogies to the N-terminal fragment of RNase A. Biophys Chem. 1986 Dec 15;25(2):127–134. doi: 10.1016/0301-4622(86)87003-x. [DOI] [PubMed] [Google Scholar]
- Brown J. E., Klee W. A. Helix-coil transition of the isolated amino terminus of ribonuclease. Biochemistry. 1971 Feb 2;10(3):470–476. doi: 10.1021/bi00779a019. [DOI] [PubMed] [Google Scholar]
- Brown L. R., De Marco A., Richarz R., Wagner G., Wüthrich K. The influence of a single salt bridge on static and dynamic features of the globular solution conformation of the basic pancreatic trypsin inhibitor. 1H and 13C nuclear-magnetic-resonance studies of the native and the transaminated inhibitor. Eur J Biochem. 1978 Jul 17;88(1):87–95. doi: 10.1111/j.1432-1033.1978.tb12425.x. [DOI] [PubMed] [Google Scholar]
- Chou P. Y., Fasman G. D. Conformational parameters for amino acids in helical, beta-sheet, and random coil regions calculated from proteins. Biochemistry. 1974 Jan 15;13(2):211–222. doi: 10.1021/bi00699a001. [DOI] [PubMed] [Google Scholar]
- Fersht A. R. Conformational equilibria in -and -chymotrypsin. The energetics and importance of the salt bridge. J Mol Biol. 1972 Mar 14;64(2):497–509. doi: 10.1016/0022-2836(72)90513-x. [DOI] [PubMed] [Google Scholar]
- Fersht A. R., Shi J. P., Knill-Jones J., Lowe D. M., Wilkinson A. J., Blow D. M., Brick P., Carter P., Waye M. M., Winter G. Hydrogen bonding and biological specificity analysed by protein engineering. Nature. 1985 Mar 21;314(6008):235–238. doi: 10.1038/314235a0. [DOI] [PubMed] [Google Scholar]
- HOLZWARTH G., DOTY P. THE ULTRAVIOLET CIRCULAR DICHROISM OF POLYPEPTIDES. J Am Chem Soc. 1965 Jan 20;87:218–228. doi: 10.1021/ja01080a015. [DOI] [PubMed] [Google Scholar]
- KAUZMANN W. Some factors in the interpretation of protein denaturation. Adv Protein Chem. 1959;14:1–63. doi: 10.1016/s0065-3233(08)60608-7. [DOI] [PubMed] [Google Scholar]
- Maxfield F. R., Alter J. E., Taylor G. T., Scheraga H. A. Helix-coil stability constants for the naturally occurring amino acids in water. IX. Glutamic acid parameters from random poly(hydroxybutylglutamine-co-L-glutamic acid). Macromolecules. 1975 Jul-Aug;8(4):479–491. doi: 10.1021/ma60046a021. [DOI] [PubMed] [Google Scholar]
- Maxfield F. R., Scheraga H. A. The effect of neighboring charges on the helix forming ability of charged amino acids in proteins. Macromolecules. 1975 Jul-Aug;8(4):491–493. doi: 10.1021/ma60046a022. [DOI] [PubMed] [Google Scholar]
- Oppenheimer H. L., Labouesse B., Hess G. P. Implication of an ionizing group in the control of conformation and activity of chymotrypsin. J Biol Chem. 1966 Jun 10;241(11):2720–2730. [PubMed] [Google Scholar]
- Perutz M. F. Electrostatic effects in proteins. Science. 1978 Sep 29;201(4362):1187–1191. doi: 10.1126/science.694508. [DOI] [PubMed] [Google Scholar]
- ROSEN H. A modified ninhydrin colorimetric analysis for amino acids. Arch Biochem Biophys. 1957 Mar;67(1):10–15. doi: 10.1016/0003-9861(57)90241-2. [DOI] [PubMed] [Google Scholar]
- Ramachandran J., Berger A., Katchalski E. Synthesis and physicochemical properties in aqueous solution of the sequential polypeptide poly(Tyr-Ala-Glu). Biopolymers. 1971 Oct;10(10):1829–1851. doi: 10.1002/bip.360101007. [DOI] [PubMed] [Google Scholar]
- Rico M., Gallego E., Santoro J., Bermejo F. J., Nieto J. L., Herranz J. On the fundamental role of the Glu 2- ... Arg 10+ salt bridge in the folding of isolated ribonuclease A S-peptide. Biochem Biophys Res Commun. 1984 Sep 17;123(2):757–763. doi: 10.1016/0006-291x(84)90294-8. [DOI] [PubMed] [Google Scholar]
- SCHELLMAN J. A. The thermodynamics of urea solutions and the heat of formation of the peptide hydrogen bond. C R Trav Lab Carlsberg Chim. 1955;29(14-15):223–229. [PubMed] [Google Scholar]
- Schechter B., Schechter I., Ramachandran J., Conway-Jacobs A., Sela M. The synthesis and circular dichroism of a series of peptides possessing the structure (L-tyrosyl-L-alanyl-L-glutamyl)n. Eur J Biochem. 1971 Jun 11;20(3):301–308. doi: 10.1111/j.1432-1033.1971.tb01394.x. [DOI] [PubMed] [Google Scholar]
- Scheraga H. A. Effect of side chain-backbone electrostatic interactions on the stability of alpha-helices. Proc Natl Acad Sci U S A. 1985 Sep;82(17):5585–5587. doi: 10.1073/pnas.82.17.5585. [DOI] [PMC free article] [PubMed] [Google Scholar]
- Shoemaker K. R., Kim P. S., Brems D. N., Marqusee S., York E. J., Chaiken I. M., Stewart J. M., Baldwin R. L. Nature of the charged-group effect on the stability of the C-peptide helix. Proc Natl Acad Sci U S A. 1985 Apr;82(8):2349–2353. doi: 10.1073/pnas.82.8.2349. [DOI] [PMC free article] [PubMed] [Google Scholar]
- Shoemaker K. R., Kim P. S., York E. J., Stewart J. M., Baldwin R. L. Tests of the helix dipole model for stabilization of alpha-helices. Nature. 1987 Apr 9;326(6113):563–567. doi: 10.1038/326563a0. [DOI] [PubMed] [Google Scholar]
- Sigler P. B., Blow D. M., Matthews B. W., Henderson R. Structure of crystalline -chymotrypsin. II. A preliminary report including a hypothesis for the activation mechanism. J Mol Biol. 1968 Jul 14;35(1):143–164. doi: 10.1016/s0022-2836(68)80043-9. [DOI] [PubMed] [Google Scholar]
- Sundaralingam M., Drendel W., Greaser M. Stabilization of the long central helix of troponin C by intrahelical salt bridges between charged amino acid side chains. Proc Natl Acad Sci U S A. 1985 Dec;82(23):7944–7947. doi: 10.1073/pnas.82.23.7944. [DOI] [PMC free article] [PubMed] [Google Scholar]
- Vasquez M., Pincus M. R., Scheraga H. A. Helix-coil transition theory including long-range electrostatic interactions: application to globular proteins. Biopolymers. 1987 Mar;26(3):351–371. doi: 10.1002/bip.360260305. [DOI] [PubMed] [Google Scholar]