Abstract
We present a comparative study of calorimetrically derived thermodynamic profiles for the binding of a series of drugs with selected DNA host duplexes. We use these data to demonstrate that comparisons between complete thermodynamic profiles (delta G zero, delta H zero, delta S zero, delta Cp) are required before drug binding can be used as a probe of DNA conformation, since enthalpy-entropy compensations can cause two drug-DNA binding events to exhibit similar binding free energies (delta G zero) despite being driven by entirely different thermodynamic forces (delta H zero, delta S zero). In this work, we employ a combination of spectroscopic and calorimetric techniques to characterize thermodynamically the DNA binding of netropsin and distamycin (two minor groove-directed ligands), ethidium (an intercalator), and daunomycin (a combined intercalator/groove binder). Our free energy data (delta G zero) show that each drug exhibits similar binding affinities at 25 degrees C for the alternating copolymer duplex poly[d(A-T)].poly[d(A-T)] and for the homopolymer duplex poly(dA).poly(dT). However, our calorimetric measurements reveal that the nature of the thermodynamic forces (delta H zero, delta S zero) that drive drug binding to these two host duplexes at 25 degrees C are entirely different, despite similar binding free energies (delta G zero) and similar salt dependencies (lnK/ln[Na+]). Specifically, the 25 degrees C binding of all four drugs to the alternating copolymer poly[d(A-T)].poly[d(A-T)] is overwhelmingly enthalpy driven, whereas the corresponding binding of each drug to the homopolymer duplex poly(dA).poly(dT) is overwhelmingly entropy driven. Thus, the similar binding free energies (delta G zero) we measure for complexation of each drug with poly[d(A-T)].poly[d(A-T)] and poly(dA).poly(dT) result from compensating changes in the enthalpy and entropy terms. Comparison with the thermodynamic profiles for the complexation of these drug molecules to other DNA host duplexes at 25 degrees C reveals that the binding of each is strongly enthalpy driven, except when the poly(dA).poly(dT) homopolymer serves as the host duplex. This comparison allows us to conclude that poly[d(A-T)].poly[d(A-T)] behaves thermodynamically as the more "normal" host duplex toward drug binding, whereas the entropy-driven binding to the poly(dA).poly(dT) duplex represents "aberrant" behavior. Furthermore, since each of the four drugs exhibits different modes of DNA binding, we conclude that the observed entropy-driven behavior for binding to poly(dA).poly(dT) reflects an intrinsic property of the homopolymer duplex that is perturbed in a common manner upon ligation rather than a common property of all four binding ligands. To rationalize the large positive entropy changes that drive drug complexation with poly(dA).poly(dT) duplex, we propose a model that emphasizes binding-induced perturbations of the more highly hydrated, altered B conformation of the homopolymer. Our results suggest that an aberrant thermodynamic binding profile may reflect an unusual DNA conformation in the host duplex. However, before such a conclusion can be reached, complete thermodynamic binding profiles must be examined, since enthalpy-entropy compensations can cause two binding events to exhibit similar binding constants even when they are driven by very different thermodynamic forces.
Full text
PDF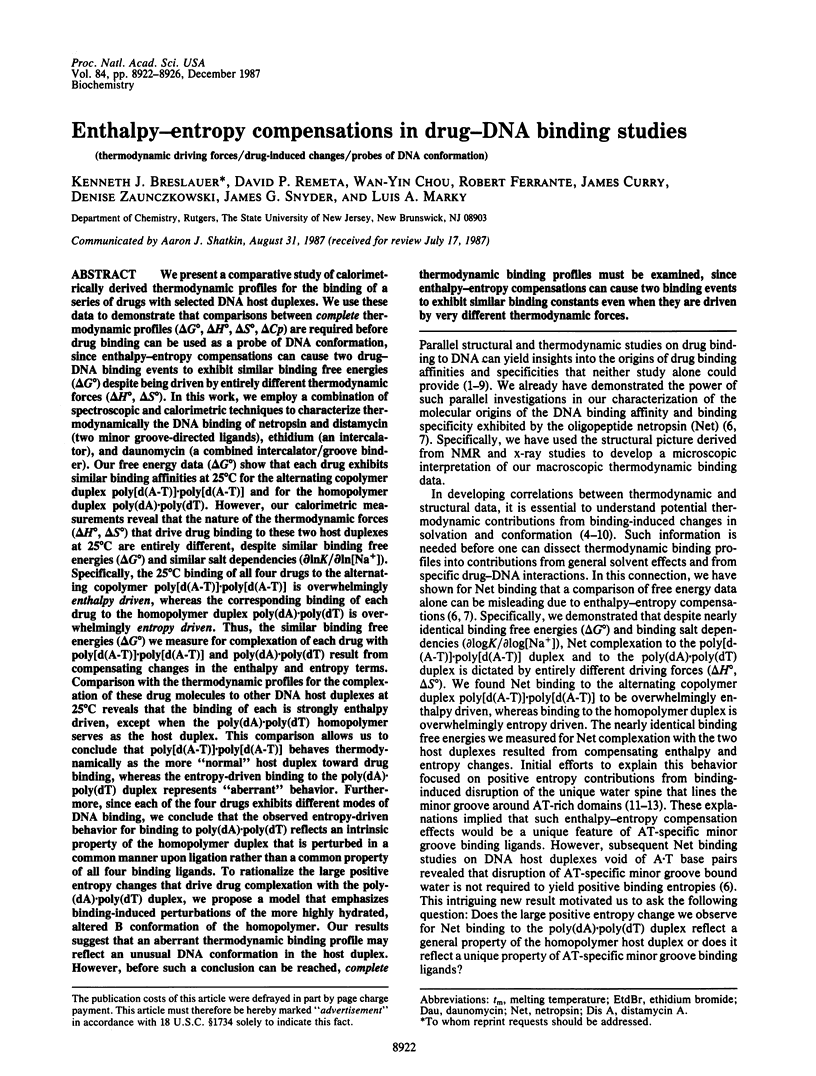
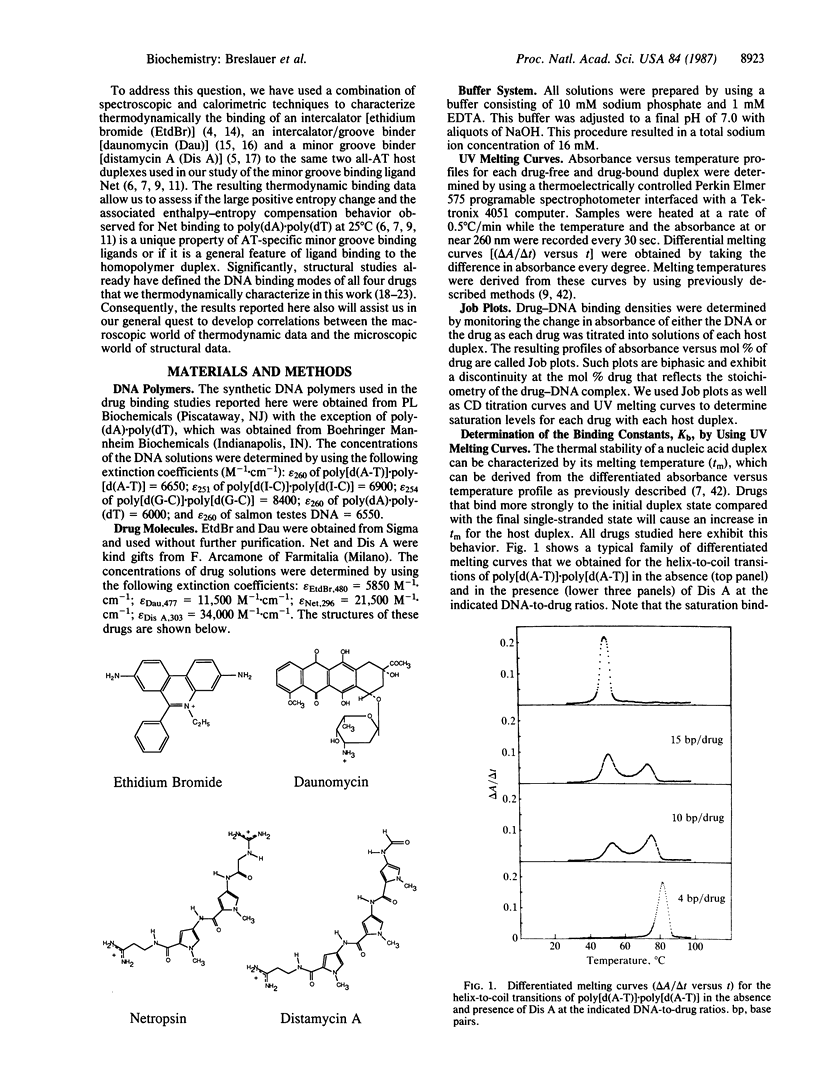
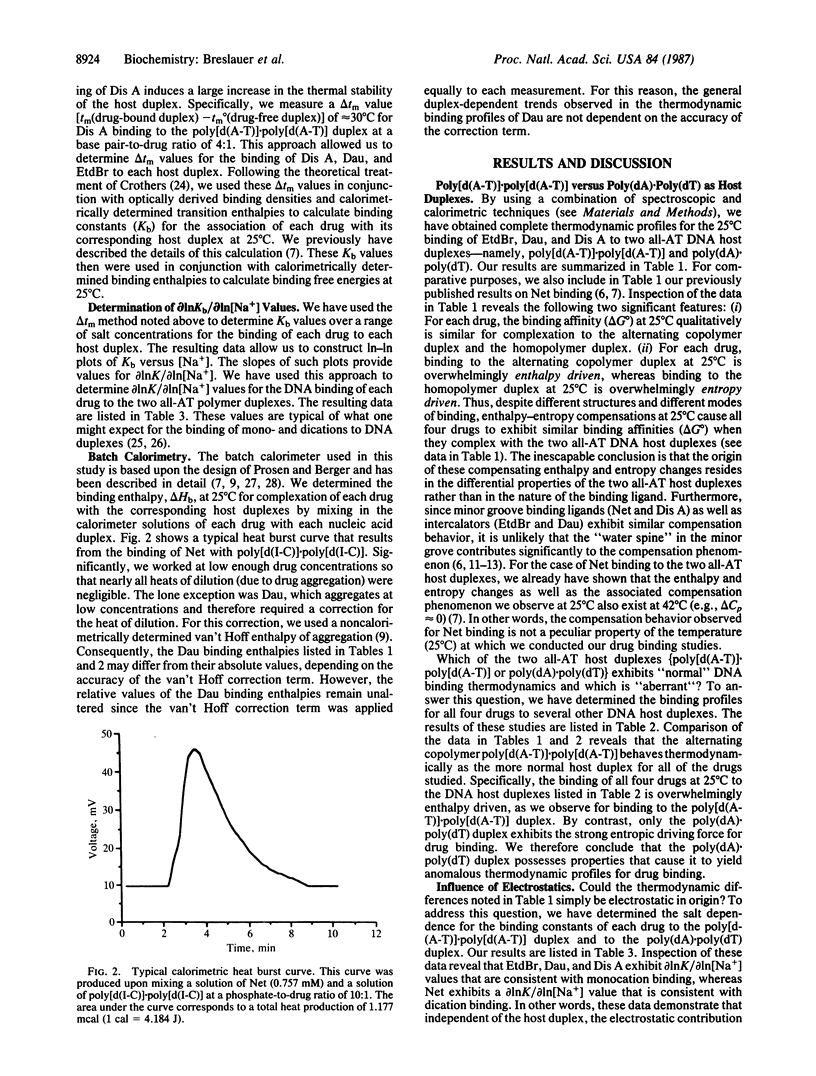
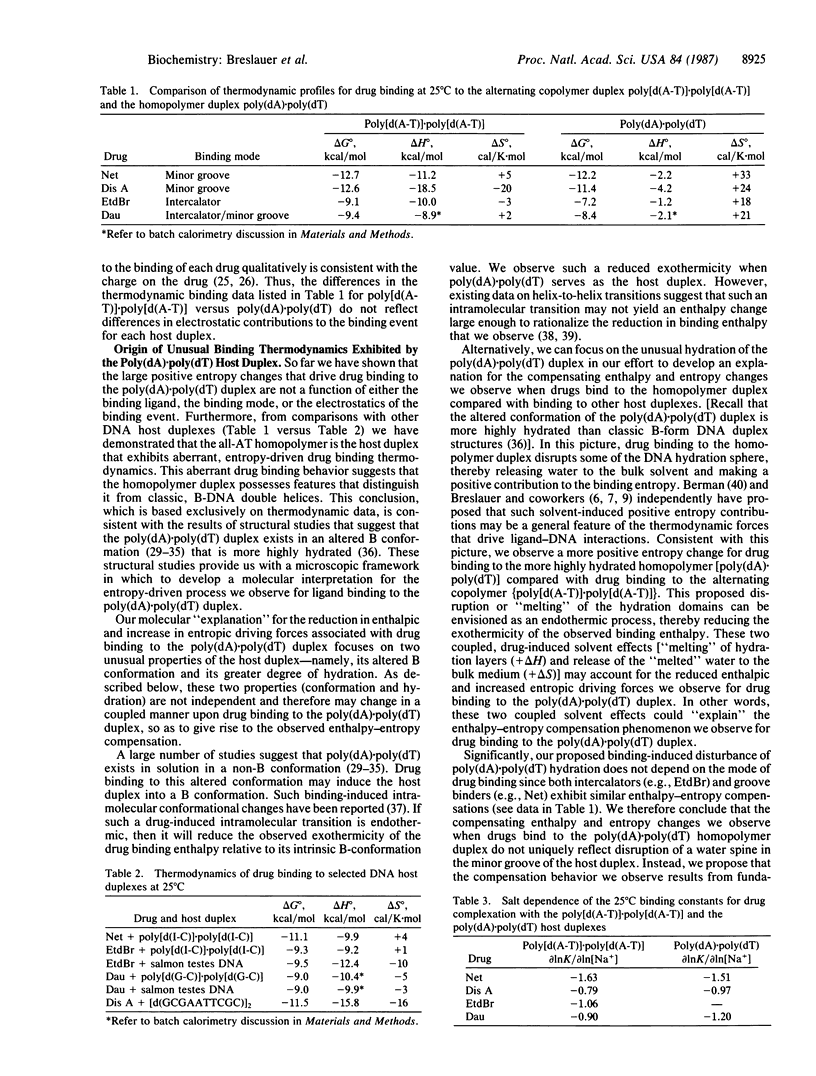
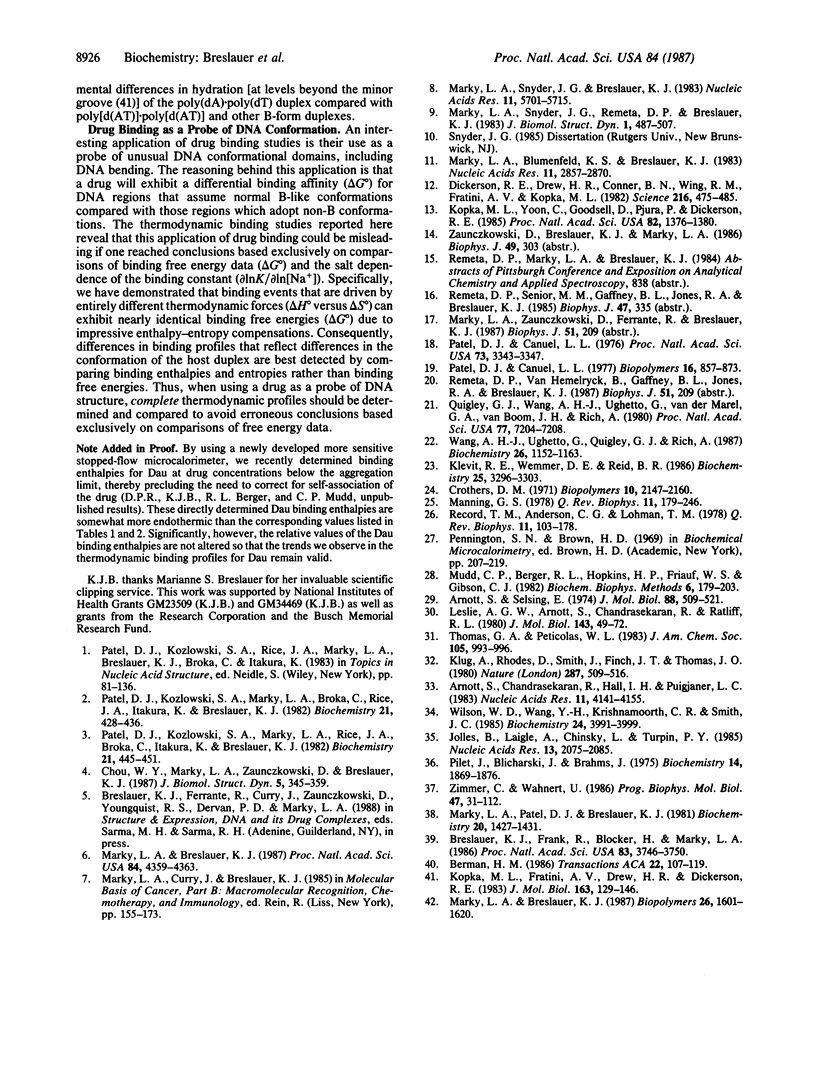
Selected References
These references are in PubMed. This may not be the complete list of references from this article.
- Arnott S., Chandrasekaran R., Hall I. H., Puigjaner L. C. Heteronomous DNA. Nucleic Acids Res. 1983 Jun 25;11(12):4141–4155. doi: 10.1093/nar/11.12.4141. [DOI] [PMC free article] [PubMed] [Google Scholar]
- Arnott S., Selsing E. Structures for the polynucleotide complexes poly(dA) with poly (dT) and poly(dT) with poly(dA) with poly (dT). J Mol Biol. 1974 Sep 15;88(2):509–521. doi: 10.1016/0022-2836(74)90498-7. [DOI] [PubMed] [Google Scholar]
- Breslauer K. J., Frank R., Blöcker H., Marky L. A. Predicting DNA duplex stability from the base sequence. Proc Natl Acad Sci U S A. 1986 Jun;83(11):3746–3750. doi: 10.1073/pnas.83.11.3746. [DOI] [PMC free article] [PubMed] [Google Scholar]
- Chou W. Y., Marky L. A., Zaunczkowski D., Breslauer K. J. The thermodynamics of drug-DNA interactions: ethidium bromide and propidium iodide. J Biomol Struct Dyn. 1987 Oct;5(2):345–359. doi: 10.1080/07391102.1987.10506399. [DOI] [PubMed] [Google Scholar]
- Crothers D. M. Statistical thermodynamics of nucleic acid melting transitions with coupled binding equilibria. Biopolymers. 1971 Nov;10(11):2147–2160. doi: 10.1002/bip.360101110. [DOI] [PubMed] [Google Scholar]
- Dickerson R. E., Drew H. R., Conner B. N., Wing R. M., Fratini A. V., Kopka M. L. The anatomy of A-, B-, and Z-DNA. Science. 1982 Apr 30;216(4545):475–485. doi: 10.1126/science.7071593. [DOI] [PubMed] [Google Scholar]
- Jollès B., Laigle A., Chinsky L., Turpin P. Y. The poly dA strand of poly dA.poly dT adopts an A-form in solution: a UV resonance Raman study. Nucleic Acids Res. 1985 Mar 25;13(6):2075–2085. doi: 10.1093/nar/13.6.2075. [DOI] [PMC free article] [PubMed] [Google Scholar]
- Klevit R. E., Wemmer D. E., Reid B. R. 1H NMR studies on the interaction between distamycin A and a symmetrical DNA dodecamer. Biochemistry. 1986 Jun 3;25(11):3296–3303. doi: 10.1021/bi00359a032. [DOI] [PubMed] [Google Scholar]
- Klug A., Rhodes D., Smith J., Finch J. T., Thomas J. O. A low resolution structure for the histone core of the nucleosome. Nature. 1980 Oct 9;287(5782):509–516. doi: 10.1038/287509a0. [DOI] [PubMed] [Google Scholar]
- Kopka M. L., Fratini A. V., Drew H. R., Dickerson R. E. Ordered water structure around a B-DNA dodecamer. A quantitative study. J Mol Biol. 1983 Jan 5;163(1):129–146. doi: 10.1016/0022-2836(83)90033-5. [DOI] [PubMed] [Google Scholar]
- Kopka M. L., Yoon C., Goodsell D., Pjura P., Dickerson R. E. The molecular origin of DNA-drug specificity in netropsin and distamycin. Proc Natl Acad Sci U S A. 1985 Mar;82(5):1376–1380. doi: 10.1073/pnas.82.5.1376. [DOI] [PMC free article] [PubMed] [Google Scholar]
- Leslie A. G., Arnott S., Chandrasekaran R., Ratliff R. L. Polymorphism of DNA double helices. J Mol Biol. 1980 Oct 15;143(1):49–72. doi: 10.1016/0022-2836(80)90124-2. [DOI] [PubMed] [Google Scholar]
- Manning G. S. The molecular theory of polyelectrolyte solutions with applications to the electrostatic properties of polynucleotides. Q Rev Biophys. 1978 May;11(2):179–246. doi: 10.1017/s0033583500002031. [DOI] [PubMed] [Google Scholar]
- Marky L. A., Blumenfeld K. S., Breslauer K. J. Calorimetric and spectroscopic investigation of drug-DNA interactions. I. The binding of netropsin to poly d(AT). Nucleic Acids Res. 1983 May 11;11(9):2857–2870. doi: 10.1093/nar/11.9.2857. [DOI] [PMC free article] [PubMed] [Google Scholar]
- Marky L. A., Breslauer K. J. Calculating thermodynamic data for transitions of any molecularity from equilibrium melting curves. Biopolymers. 1987 Sep;26(9):1601–1620. doi: 10.1002/bip.360260911. [DOI] [PubMed] [Google Scholar]
- Marky L. A., Breslauer K. J. Origins of netropsin binding affinity and specificity: correlations of thermodynamic and structural data. Proc Natl Acad Sci U S A. 1987 Jul;84(13):4359–4363. doi: 10.1073/pnas.84.13.4359. [DOI] [PMC free article] [PubMed] [Google Scholar]
- Marky L. A., Patel D., Breslauer K. J. Effect of tetramethylammonium ion on the helix-to-coil transition of poly(deoxyadenylylthymidine): a nuclear magnetic resonance and calorimetric investigation. Biochemistry. 1981 Mar 17;20(6):1427–1431. doi: 10.1021/bi00509a004. [DOI] [PubMed] [Google Scholar]
- Marky L. A., Snyder J. G., Breslauer K. J. Calorimetric and spectroscopic investigation of drug--DNA interactions: II. Dipyrandium binding to poly d(AT). Nucleic Acids Res. 1983 Aug 25;11(16):5701–5715. doi: 10.1093/nar/11.16.5701. [DOI] [PMC free article] [PubMed] [Google Scholar]
- Marky L. A., Snyder J. G., Remeta D. P., Breslauer K. J. Thermodynamics of drug-DNA interactions. J Biomol Struct Dyn. 1983 Oct;1(2):487–507. doi: 10.1080/07391102.1983.10507457. [DOI] [PubMed] [Google Scholar]
- Mudd C., Berger R. L., Hopkins H. P., Friauf W. S., Gibson C. An optimized differential heat conduction solution microcalorimeter for thermal kinetic measurements. J Biochem Biophys Methods. 1982 Aug;6(3):179–203. doi: 10.1016/0165-022x(82)90042-2. [DOI] [PubMed] [Google Scholar]
- Patel D. J., Canuel L. L. Biphasic helix-coil transition of the ethidium bromide-poly (dA-dT) and the propidium diiodide - poly (dA-dT) complexes. Stabilization of base-pair regions centered about the intercalation site. Biopolymers. 1977 Apr;16(4):857–873. doi: 10.1002/bip.1977.360160410. [DOI] [PubMed] [Google Scholar]
- Patel D. J., Canuel L. L. Ethidium bromide-(dC-dG-dC-dG)2 complex in solution: intercalation and sequence specificity of drug binding at the tetranucleotide duplex level. Proc Natl Acad Sci U S A. 1976 Oct;73(10):3343–3347. doi: 10.1073/pnas.73.10.3343. [DOI] [PMC free article] [PubMed] [Google Scholar]
- Patel D. J., Kozlowski S. A., Marky L. A., Broka C., Rice J. A., Itakura K., Breslauer K. J. Premelting and melting transitions in the d(CGCGAATTCGCG) self-complementary duplex in solution. Biochemistry. 1982 Feb 2;21(3):428–436. doi: 10.1021/bi00532a002. [DOI] [PubMed] [Google Scholar]
- Patel D. J., Kozlowski S. A., Marky L. A., Rice J. A., Broka C., Itakura K., Breslauer K. J. Extra adenosine stacks into the self-complementary d(CGCAGAATTCGCG) duplex in solution. Biochemistry. 1982 Feb 2;21(3):445–451. doi: 10.1021/bi00532a004. [DOI] [PubMed] [Google Scholar]
- Pilet J., Blicharski J., Brahms J. Conformations and structural transitions in polydeoxynucleotides. Biochemistry. 1975 May 6;14(9):1869–1876. doi: 10.1021/bi00680a011. [DOI] [PubMed] [Google Scholar]
- Quigley G. J., Wang A. H., Ughetto G., van der Marel G., van Boom J. H., Rich A. Molecular structure of an anticancer drug-DNA complex: daunomycin plus d(CpGpTpApCpG). Proc Natl Acad Sci U S A. 1980 Dec;77(12):7204–7208. doi: 10.1073/pnas.77.12.7204. [DOI] [PMC free article] [PubMed] [Google Scholar]
- Record M. T., Jr, Anderson C. F., Lohman T. M. Thermodynamic analysis of ion effects on the binding and conformational equilibria of proteins and nucleic acids: the roles of ion association or release, screening, and ion effects on water activity. Q Rev Biophys. 1978 May;11(2):103–178. doi: 10.1017/s003358350000202x. [DOI] [PubMed] [Google Scholar]
- Wang A. H., Ughetto G., Quigley G. J., Rich A. Interactions between an anthracycline antibiotic and DNA: molecular structure of daunomycin complexed to d(CpGpTpApCpG) at 1.2-A resolution. Biochemistry. 1987 Feb 24;26(4):1152–1163. doi: 10.1021/bi00378a025. [DOI] [PubMed] [Google Scholar]
- Wilson W. D., Wang Y. H., Krishnamoorthy C. R., Smith J. C. Poly(dA).poly(dT) exists in an unusual conformation under physiological conditions: propidium binding to poly(dA).poly(dT) and poly[d(A-T)].poly[d(A-T)]. Biochemistry. 1985 Jul 16;24(15):3991–3999. doi: 10.1021/bi00336a029. [DOI] [PubMed] [Google Scholar]
- Zimmer C., Wähnert U. Nonintercalating DNA-binding ligands: specificity of the interaction and their use as tools in biophysical, biochemical and biological investigations of the genetic material. Prog Biophys Mol Biol. 1986;47(1):31–112. doi: 10.1016/0079-6107(86)90005-2. [DOI] [PubMed] [Google Scholar]