Abstract
Oxygen-derived free radicals have been implicated in the pathogenesis of cardiac dysfunction during ischemia, postischemic myocardial "stunning," and reperfusion injury. We investigated the effects of oxygen-derived free radicals on cardiac function in intact isolated rabbit hearts and single guinea pig ventricular myocytes. In the intact rabbit ventricle, exposure to free radical-generating systems caused increased cellular K+ efflux, shortening of the action potential duration, changes in tension, and depletion of high energy phosphates similar to ischemia and metabolic inhibition. In patch-clamped single ventricular myocytes, free radical-generating systems activated ATP-sensitive K+ channels, decreased the calcium current, and caused cell shortening by irreversibly inhibiting glycolytic and oxidative metabolism. The results suggest that free radicals generated during ischemia and reperfusion may contribute to electrophysiologic abnormalities and contractile dysfunction by inhibiting glycolysis and oxidative phosphorylation. Inhibition of metabolism by free radicals may be an important factor limiting functional recovery from an ischemic insult after reestablishment of effective blood flow.
Full text
PDF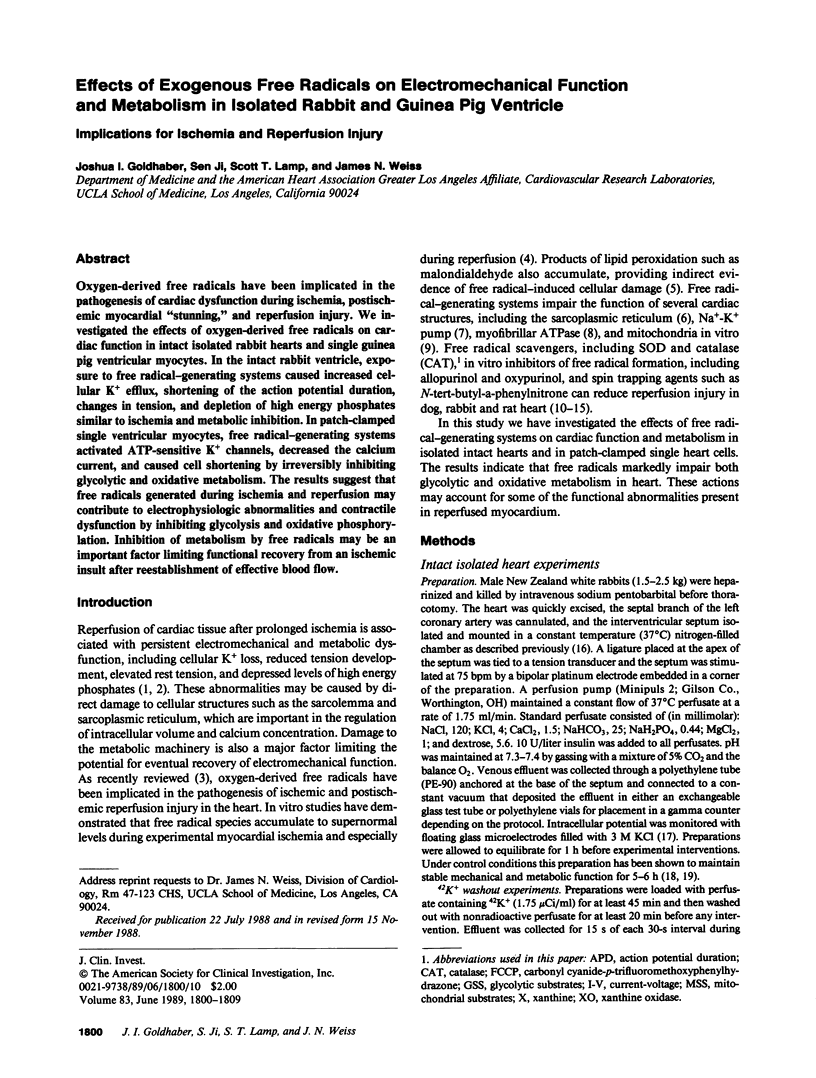
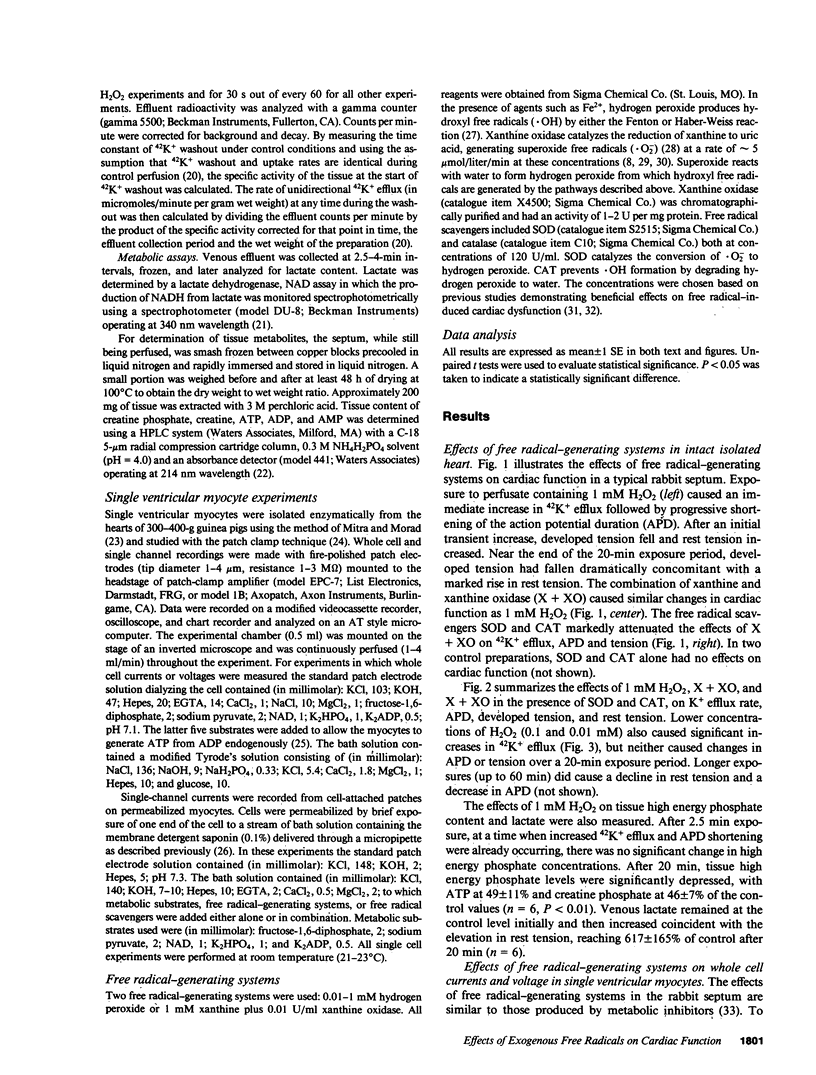
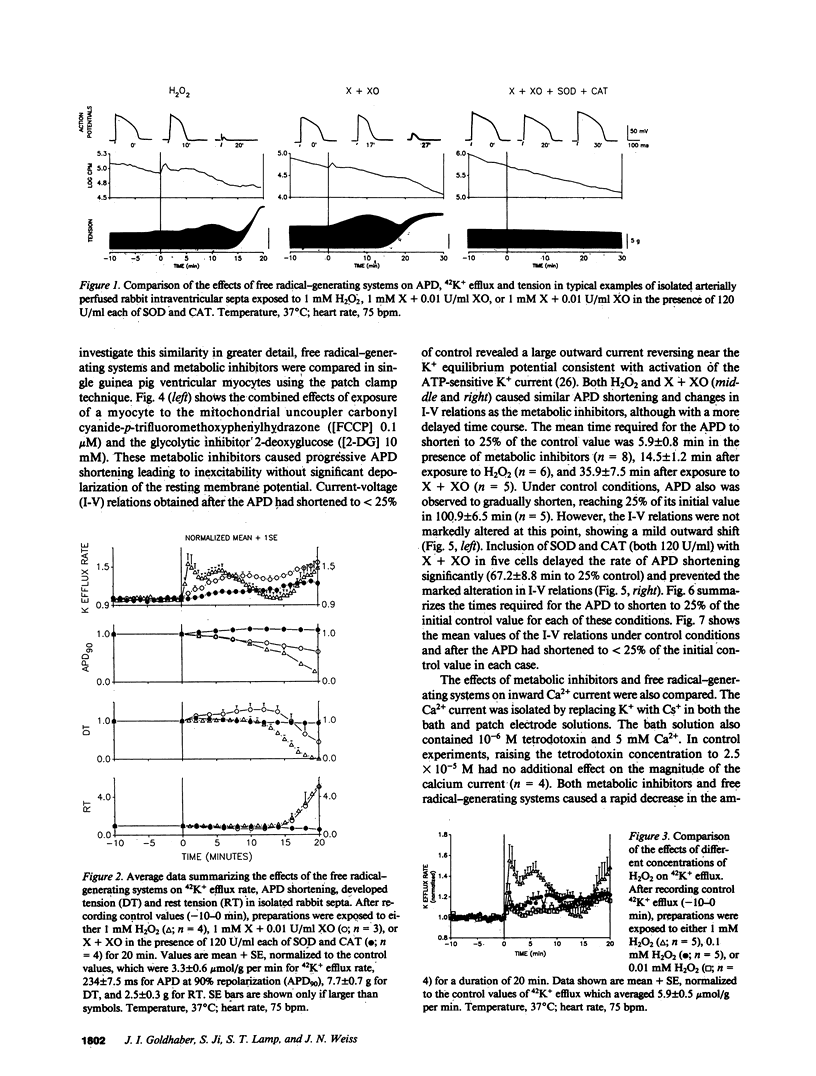
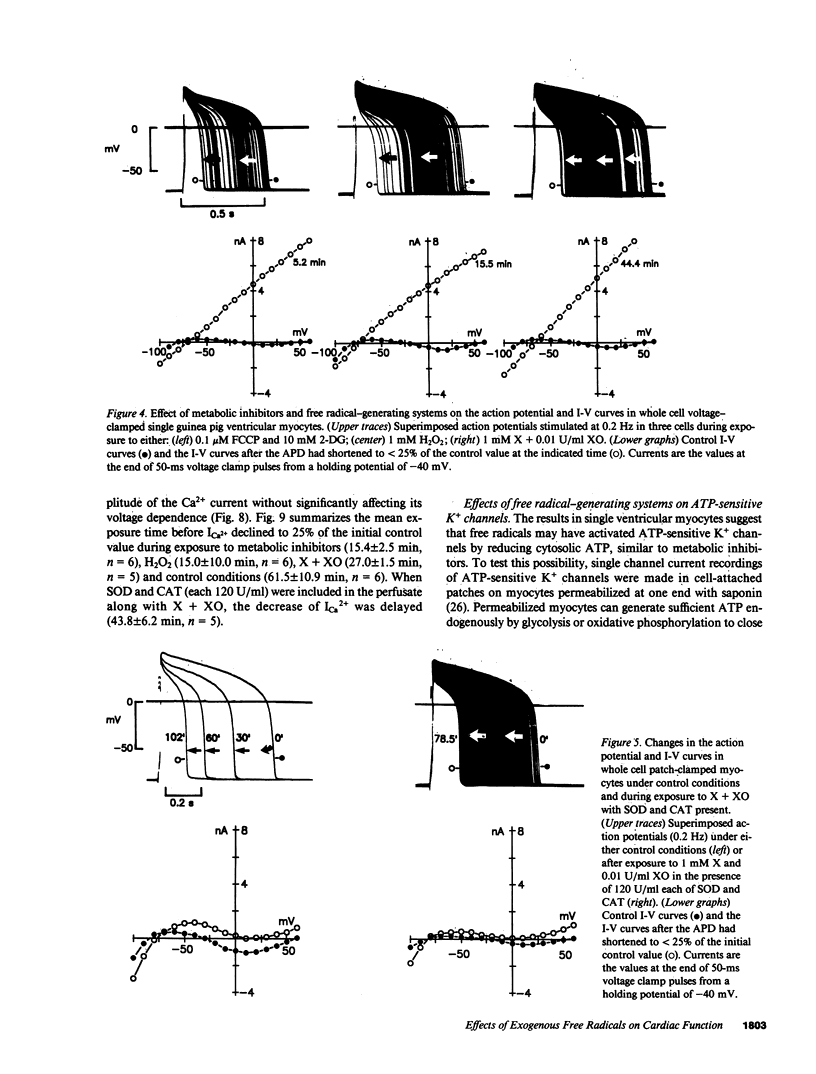
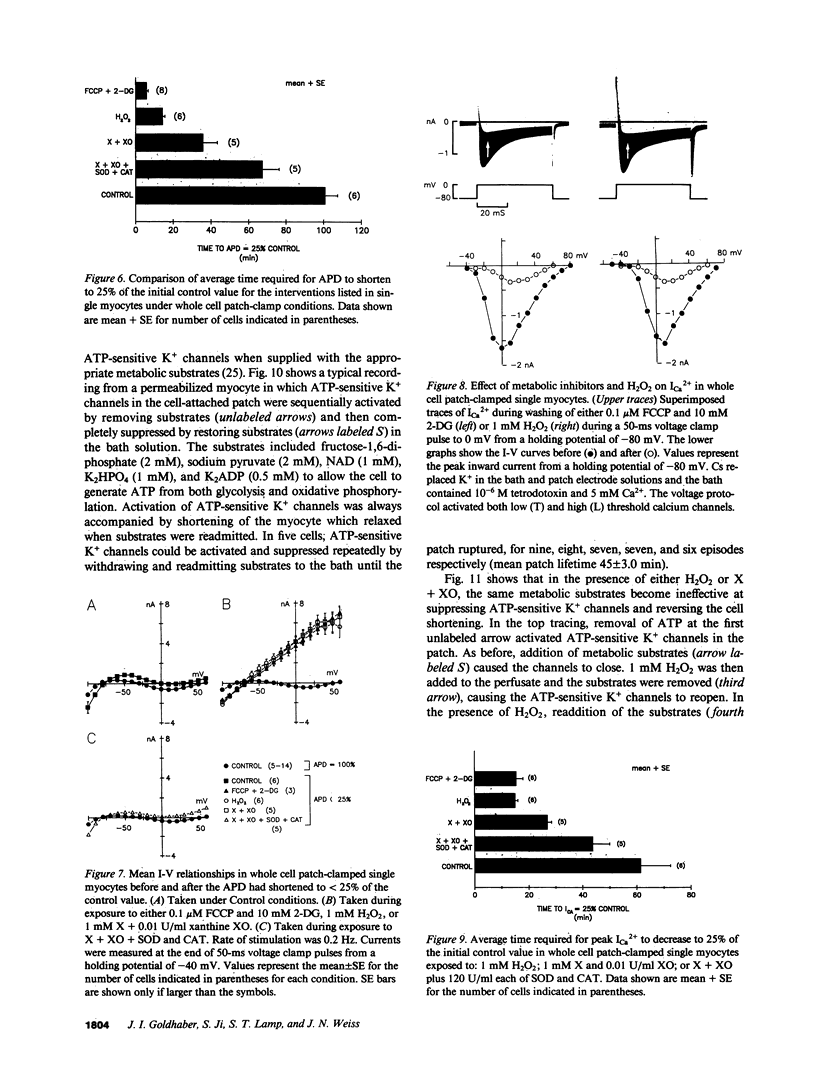
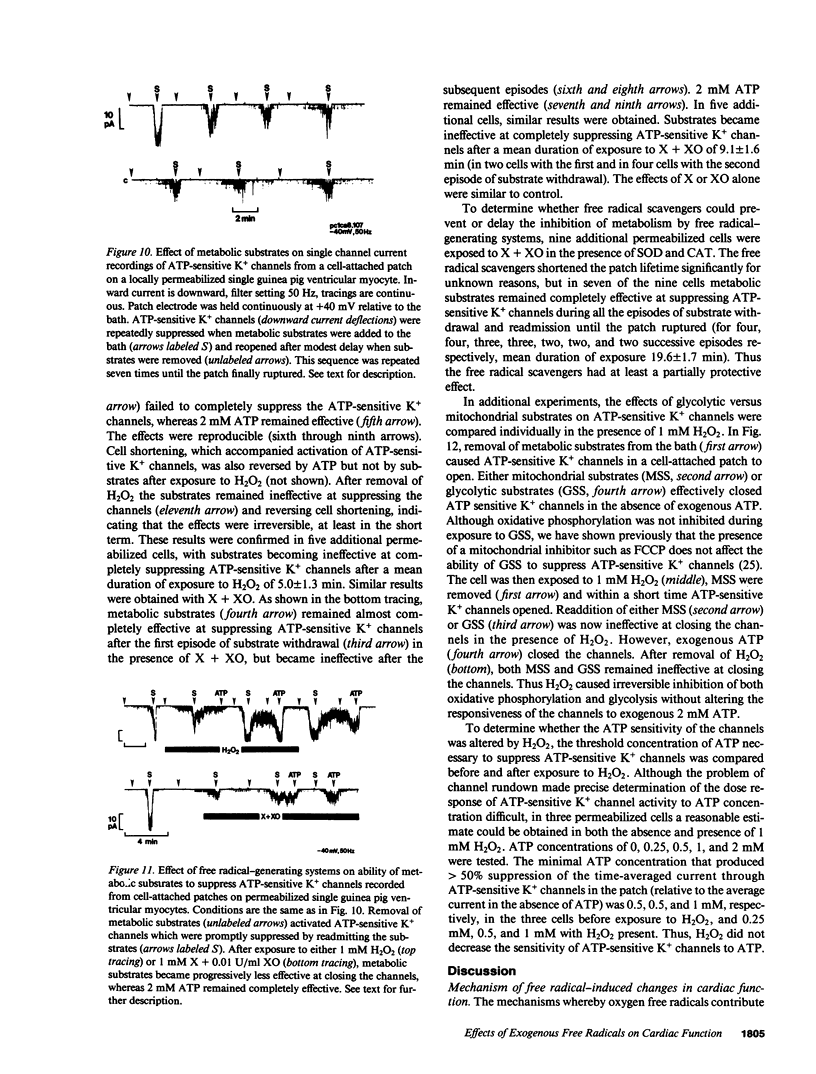
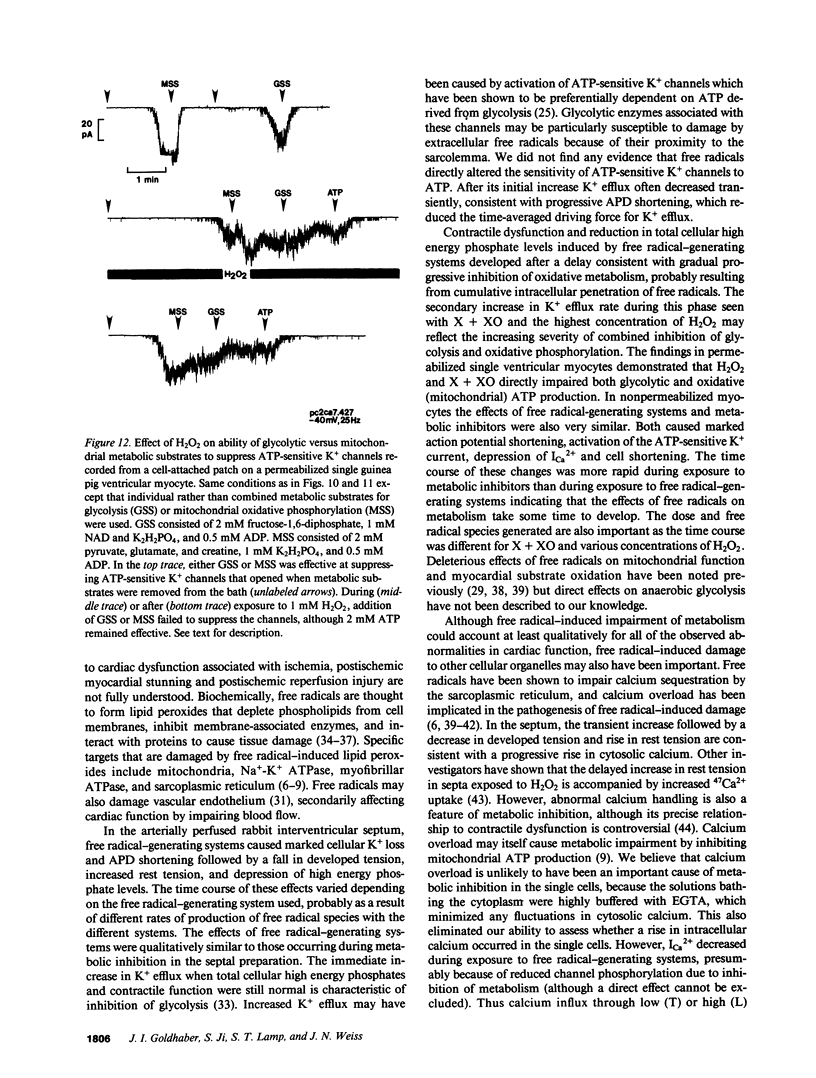
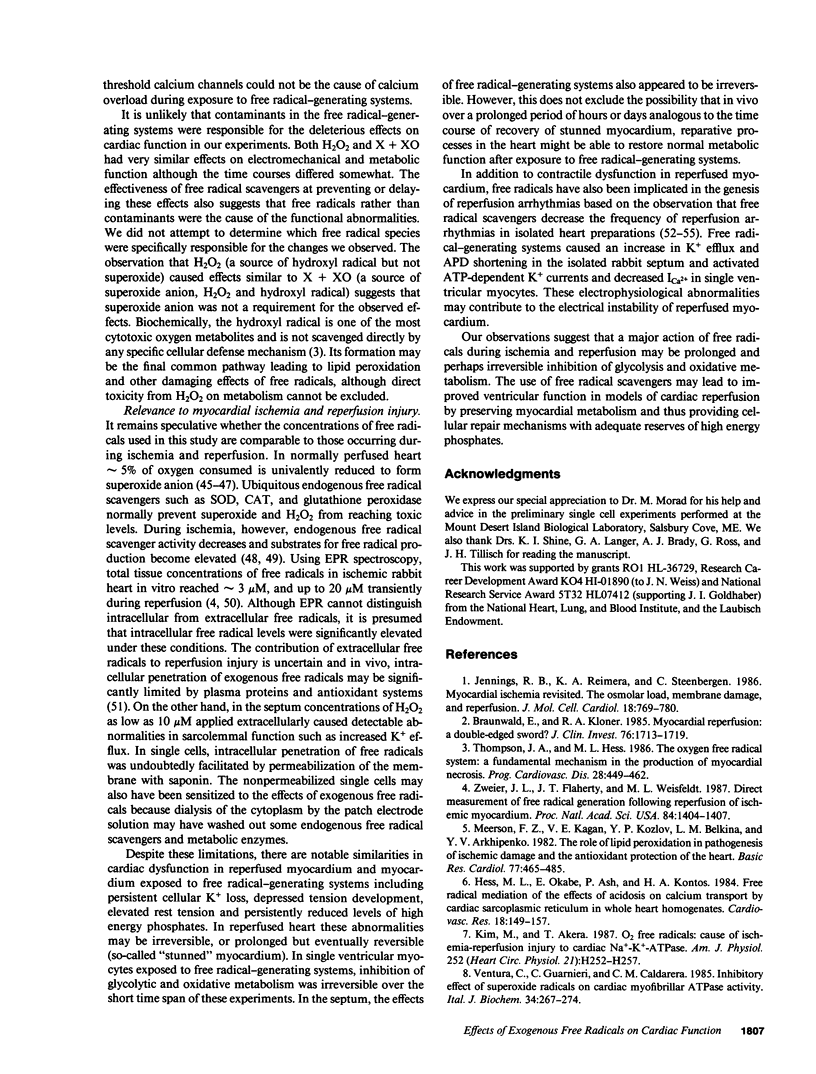
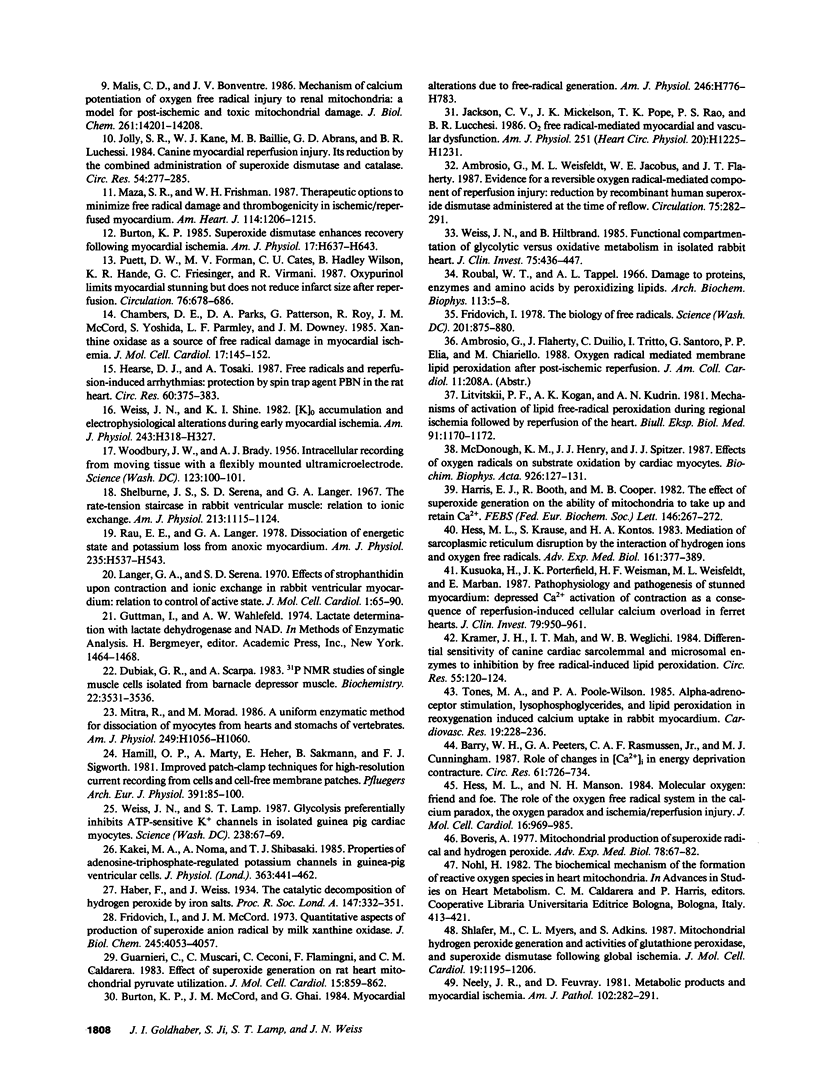
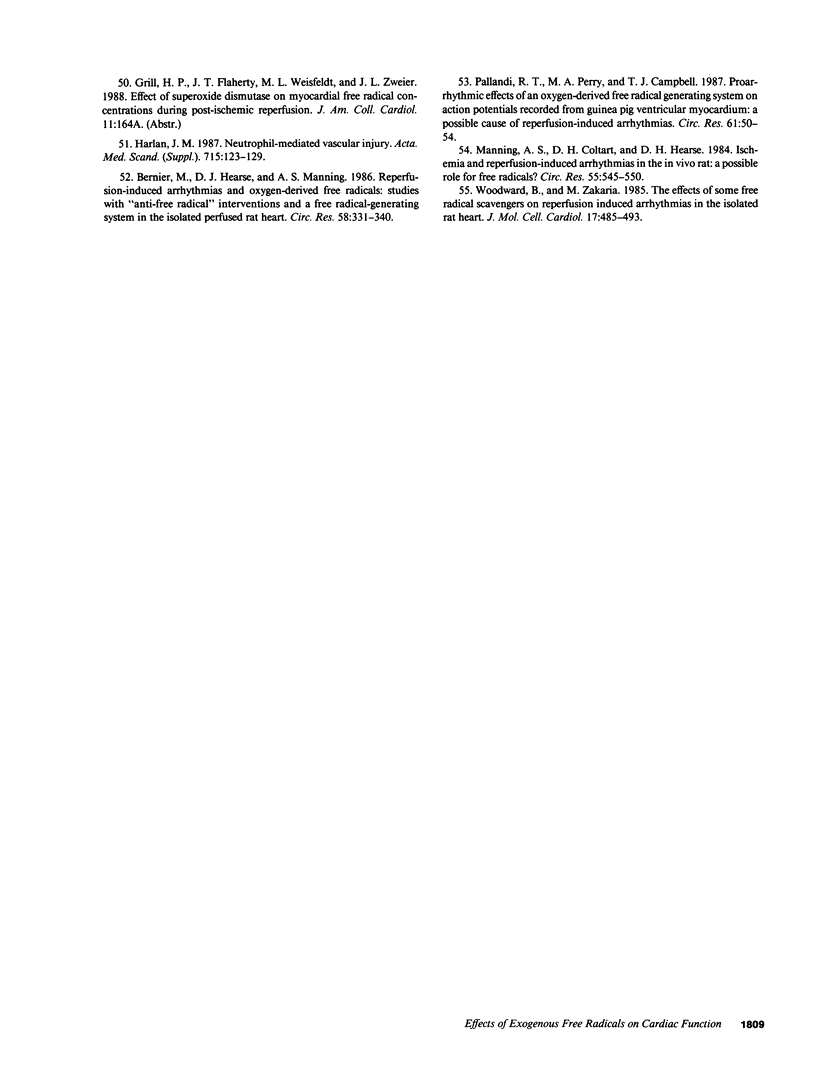
Selected References
These references are in PubMed. This may not be the complete list of references from this article.
- Ambrosio G., Weisfeldt M. L., Jacobus W. E., Flaherty J. T. Evidence for a reversible oxygen radical-mediated component of reperfusion injury: reduction by recombinant human superoxide dismutase administered at the time of reflow. Circulation. 1987 Jan;75(1):282–291. doi: 10.1161/01.cir.75.1.282. [DOI] [PubMed] [Google Scholar]
- Barry W. H., Peeters G. A., Rasmussen C. A., Jr, Cunningham M. J. Role of changes in [Ca2+]i in energy deprivation contracture. Circ Res. 1987 Nov;61(5):726–734. doi: 10.1161/01.res.61.5.726. [DOI] [PubMed] [Google Scholar]
- Bernier M., Hearse D. J., Manning A. S. Reperfusion-induced arrhythmias and oxygen-derived free radicals. Studies with "anti-free radical" interventions and a free radical-generating system in the isolated perfused rat heart. Circ Res. 1986 Mar;58(3):331–340. doi: 10.1161/01.res.58.3.331. [DOI] [PubMed] [Google Scholar]
- Boveris A. Mitochondrial production of superoxide radical and hydrogen peroxide. Adv Exp Med Biol. 1977;78:67–82. doi: 10.1007/978-1-4615-9035-4_5. [DOI] [PubMed] [Google Scholar]
- Braunwald E., Kloner R. A. Myocardial reperfusion: a double-edged sword? J Clin Invest. 1985 Nov;76(5):1713–1719. doi: 10.1172/JCI112160. [DOI] [PMC free article] [PubMed] [Google Scholar]
- Burton K. P., McCord J. M., Ghai G. Myocardial alterations due to free-radical generation. Am J Physiol. 1984 Jun;246(6 Pt 2):H776–H783. doi: 10.1152/ajpheart.1984.246.6.H776. [DOI] [PubMed] [Google Scholar]
- Burton K. P. Superoxide dismutase enhances recovery following myocardial ischemia. Am J Physiol. 1985 May;248(5 Pt 2):H637–H643. doi: 10.1152/ajpheart.1985.248.5.H637. [DOI] [PubMed] [Google Scholar]
- Chambers D. E., Parks D. A., Patterson G., Roy R., McCord J. M., Yoshida S., Parmley L. F., Downey J. M. Xanthine oxidase as a source of free radical damage in myocardial ischemia. J Mol Cell Cardiol. 1985 Feb;17(2):145–152. doi: 10.1016/s0022-2828(85)80017-1. [DOI] [PubMed] [Google Scholar]
- Dubyak G. R., Scarpa A. Phosphorus-31 nuclear magnetic resonance studies of single muscle cells isolated from barnacle depressor muscle. Biochemistry. 1983 Jul 5;22(14):3531–3536. doi: 10.1021/bi00283a035. [DOI] [PubMed] [Google Scholar]
- Fridovich I. Quantitative aspects of the production of superoxide anion radical by milk xanthine oxidase. J Biol Chem. 1970 Aug 25;245(16):4053–4057. [PubMed] [Google Scholar]
- Fridovich I. The biology of oxygen radicals. Science. 1978 Sep 8;201(4359):875–880. doi: 10.1126/science.210504. [DOI] [PubMed] [Google Scholar]
- Guarnieri C., Muscari C., Ceconi C., Flamigni F., Caldarera C. M. Effect of superoxide generation on rat heart mitochondrial pyruvate utilization. J Mol Cell Cardiol. 1983 Dec;15(12):859–862. doi: 10.1016/0022-2828(83)90348-6. [DOI] [PubMed] [Google Scholar]
- Hamill O. P., Marty A., Neher E., Sakmann B., Sigworth F. J. Improved patch-clamp techniques for high-resolution current recording from cells and cell-free membrane patches. Pflugers Arch. 1981 Aug;391(2):85–100. doi: 10.1007/BF00656997. [DOI] [PubMed] [Google Scholar]
- Harlan J. M. Neutrophil-mediated vascular injury. Acta Med Scand Suppl. 1987;715:123–129. doi: 10.1111/j.0954-6820.1987.tb09912.x. [DOI] [PubMed] [Google Scholar]
- Harris E. J., Booth R., Cooper M. B. The effect of superoxide generation on the ability of mitochondria to take up and retain Ca2+. FEBS Lett. 1982 Sep 20;146(2):267–272. doi: 10.1016/0014-5793(82)80932-0. [DOI] [PubMed] [Google Scholar]
- Hearse D. J., Tosaki A. Free radicals and reperfusion-induced arrhythmias: protection by spin trap agent PBN in the rat heart. Circ Res. 1987 Mar;60(3):375–383. doi: 10.1161/01.res.60.3.375. [DOI] [PubMed] [Google Scholar]
- Hess M. L., Krause S., Kontos H. A. Mediation of sarcoplasmic reticulum disruption in the ischemic myocardium: proposed mechanism by the interaction of hydrogen ions and oxygen free radicals. Adv Exp Med Biol. 1983;161:377–389. doi: 10.1007/978-1-4684-4472-8_21. [DOI] [PubMed] [Google Scholar]
- Hess M. L., Manson N. H. Molecular oxygen: friend and foe. The role of the oxygen free radical system in the calcium paradox, the oxygen paradox and ischemia/reperfusion injury. J Mol Cell Cardiol. 1984 Nov;16(11):969–985. doi: 10.1016/s0022-2828(84)80011-5. [DOI] [PubMed] [Google Scholar]
- Hess M. L., Okabe E., Ash P., Kontos H. A. Free radical mediation of the effects of acidosis on calcium transport by cardiac sarcoplasmic reticulum in whole heart homogenates. Cardiovasc Res. 1984 Mar;18(3):149–157. doi: 10.1093/cvr/18.3.149. [DOI] [PubMed] [Google Scholar]
- Jackson C. V., Mickelson J. K., Pope T. K., Rao P. S., Lucchesi B. R. O2 free radical-mediated myocardial and vascular dysfunction. Am J Physiol. 1986 Dec;251(6 Pt 2):H1225–H1231. doi: 10.1152/ajpheart.1986.251.6.H1225. [DOI] [PubMed] [Google Scholar]
- Jennings R. B., Reimer K. A., Steenbergen C. Myocardial ischemia revisited. The osmolar load, membrane damage, and reperfusion. J Mol Cell Cardiol. 1986 Aug;18(8):769–780. doi: 10.1016/s0022-2828(86)80952-x. [DOI] [PubMed] [Google Scholar]
- Jolly S. R., Kane W. J., Bailie M. B., Abrams G. D., Lucchesi B. R. Canine myocardial reperfusion injury. Its reduction by the combined administration of superoxide dismutase and catalase. Circ Res. 1984 Mar;54(3):277–285. doi: 10.1161/01.res.54.3.277. [DOI] [PubMed] [Google Scholar]
- Kakei M., Noma A., Shibasaki T. Properties of adenosine-triphosphate-regulated potassium channels in guinea-pig ventricular cells. J Physiol. 1985 Jun;363:441–462. doi: 10.1113/jphysiol.1985.sp015721. [DOI] [PMC free article] [PubMed] [Google Scholar]
- Kim M. S., Akera T. O2 free radicals: cause of ischemia-reperfusion injury to cardiac Na+-K+-ATPase. Am J Physiol. 1987 Feb;252(2 Pt 2):H252–H257. doi: 10.1152/ajpheart.1987.252.2.H252. [DOI] [PubMed] [Google Scholar]
- Kramer J. H., Mak I. T., Weglicki W. B. Differential sensitivity of canine cardiac sarcolemmal and microsomal enzymes to inhibition by free radical-induced lipid peroxidation. Circ Res. 1984 Jul;55(1):120–124. doi: 10.1161/01.res.55.1.120. [DOI] [PubMed] [Google Scholar]
- Kusuoka H., Porterfield J. K., Weisman H. F., Weisfeldt M. L., Marban E. Pathophysiology and pathogenesis of stunned myocardium. Depressed Ca2+ activation of contraction as a consequence of reperfusion-induced cellular calcium overload in ferret hearts. J Clin Invest. 1987 Mar;79(3):950–961. doi: 10.1172/JCI112906. [DOI] [PMC free article] [PubMed] [Google Scholar]
- Langer G. A., Serena S. D. Effects of strophanthidin upon contraction and ionic exchange in rabbit ventricular myocardium: relation to control of active state. J Mol Cell Cardiol. 1970 Mar;1(1):65–90. doi: 10.1016/0022-2828(70)90029-5. [DOI] [PubMed] [Google Scholar]
- Malis C. D., Bonventre J. V. Mechanism of calcium potentiation of oxygen free radical injury to renal mitochondria. A model for post-ischemic and toxic mitochondrial damage. J Biol Chem. 1986 Oct 25;261(30):14201–14208. [PubMed] [Google Scholar]
- Manning A. S., Coltart D. J., Hearse D. J. Ischemia and reperfusion-induced arrhythmias in the rat. Effects of xanthine oxidase inhibition with allopurinol. Circ Res. 1984 Oct;55(4):545–548. doi: 10.1161/01.res.55.4.545. [DOI] [PubMed] [Google Scholar]
- Maza S. R., Frishman W. H. Therapeutic options to minimize free radical damage and thrombogenicity in ischemic/reperfused myocardium. Am Heart J. 1987 Nov;114(5):1206–1215. doi: 10.1016/0002-8703(87)90198-0. [DOI] [PubMed] [Google Scholar]
- McDonough K. H., Henry J. J., Spitzer J. J. Effects of oxygen radicals on substrate oxidation by cardiac myocytes. Biochim Biophys Acta. 1987 Nov 6;926(2):127–131. doi: 10.1016/0304-4165(87)90228-5. [DOI] [PubMed] [Google Scholar]
- Meerson F. Z., Kagan V. E., Kozlov YuP, Belkina L. M., Arkhipenko YuV The role of lipid peroxidation in pathogenesis of ischemic damage and the antioxidant protection of the heart. Basic Res Cardiol. 1982 Sep-Oct;77(5):465–485. doi: 10.1007/BF01907940. [DOI] [PubMed] [Google Scholar]
- Mitra R., Morad M. A uniform enzymatic method for dissociation of myocytes from hearts and stomachs of vertebrates. Am J Physiol. 1985 Nov;249(5 Pt 2):H1056–H1060. doi: 10.1152/ajpheart.1985.249.5.H1056. [DOI] [PubMed] [Google Scholar]
- Neely J. R., Feuvray D. Metabolic products and myocardial ischemia. Am J Pathol. 1981 Feb;102(2):282–291. [PMC free article] [PubMed] [Google Scholar]
- Pallandi R. T., Perry M. A., Campbell T. J. Proarrhythmic effects of an oxygen-derived free radical generating system on action potentials recorded from guinea pig ventricular myocardium: a possible cause of reperfusion-induced arrhythmias. Circ Res. 1987 Jul;61(1):50–54. doi: 10.1161/01.res.61.1.50. [DOI] [PubMed] [Google Scholar]
- Puett D. W., Forman M. B., Cates C. U., Wilson B. H., Hande K. R., Friesinger G. C., Virmani R. Oxypurinol limits myocardial stunning but does not reduce infarct size after reperfusion. Circulation. 1987 Sep;76(3):678–686. doi: 10.1161/01.cir.76.3.678. [DOI] [PubMed] [Google Scholar]
- Rau E. E., Langer G. A. Dissociation of energetic state and potassium loss from anoxic myocardium. Am J Physiol. 1978 Nov;235(5):H537–H543. doi: 10.1152/ajpheart.1978.235.5.H537. [DOI] [PubMed] [Google Scholar]
- Roubal W. T., Tappel A. L. Damage to proteins, enzymes, and amino acids by peroxidizing lipids. Arch Biochem Biophys. 1966 Jan;113(1):5–8. doi: 10.1016/0003-9861(66)90150-0. [DOI] [PubMed] [Google Scholar]
- Shelburne J. C., Serena S. D., Langer G. A. Rate-tension staircase in rabbit ventricular muscle: relation to ionic exchange. Am J Physiol. 1967 Nov;213(5):1115–1124. doi: 10.1152/ajplegacy.1967.213.5.1115. [DOI] [PubMed] [Google Scholar]
- Shlafer M., Myers C. L., Adkins S. Mitochondrial hydrogen peroxide generation and activities of glutathione peroxidase and superoxide dismutase following global ischemia. J Mol Cell Cardiol. 1987 Dec;19(12):1195–1206. doi: 10.1016/s0022-2828(87)80530-8. [DOI] [PubMed] [Google Scholar]
- Thompson J. A., Hess M. L. The oxygen free radical system: a fundamental mechanism in the production of myocardial necrosis. Prog Cardiovasc Dis. 1986 May-Jun;28(6):449–462. doi: 10.1016/0033-0620(86)90027-7. [DOI] [PubMed] [Google Scholar]
- Tones M. A., Poole-Wilson P. A. Alpha-adrenoceptor stimulation, lysophosphoglycerides, and lipid peroxidation in reoxygenation induced calcium uptake in rabbit myocardium. Cardiovasc Res. 1985 Apr;19(4):228–236. doi: 10.1093/cvr/19.4.228. [DOI] [PubMed] [Google Scholar]
- Ventura C., Guarnieri C., Caldarera C. M. Inhibitory effect of superoxide radicals on cardiac myofibrillar ATPase activity. Ital J Biochem. 1985 Jul-Aug;34(4):267–274. [PubMed] [Google Scholar]
- WOODBURY J. W., BRADY A. J. Intracellular recording from moving tissues with a flexibly mounted ultramicroelectrode. Science. 1956 Jan 20;123(3186):100–101. doi: 10.1126/science.123.3186.100-a. [DOI] [PubMed] [Google Scholar]
- Weiss J. N., Lamp S. T. Glycolysis preferentially inhibits ATP-sensitive K+ channels in isolated guinea pig cardiac myocytes. Science. 1987 Oct 2;238(4823):67–69. doi: 10.1126/science.2443972. [DOI] [PubMed] [Google Scholar]
- Weiss J., Hiltbrand B. Functional compartmentation of glycolytic versus oxidative metabolism in isolated rabbit heart. J Clin Invest. 1985 Feb;75(2):436–447. doi: 10.1172/JCI111718. [DOI] [PMC free article] [PubMed] [Google Scholar]
- Weiss J., Shine K. I. [K+]o accumulation and electrophysiological alterations during early myocardial ischemia. Am J Physiol. 1982 Aug;243(2):H318–H327. doi: 10.1152/ajpheart.1982.243.2.H318. [DOI] [PubMed] [Google Scholar]
- Woodward B., Zakaria M. N. Effect of some free radical scavengers on reperfusion induced arrhythmias in the isolated rat heart. J Mol Cell Cardiol. 1985 May;17(5):485–493. doi: 10.1016/s0022-2828(85)80053-5. [DOI] [PubMed] [Google Scholar]
- Zweier J. L., Flaherty J. T., Weisfeldt M. L. Direct measurement of free radical generation following reperfusion of ischemic myocardium. Proc Natl Acad Sci U S A. 1987 Mar;84(5):1404–1407. doi: 10.1073/pnas.84.5.1404. [DOI] [PMC free article] [PubMed] [Google Scholar]