Abstract
In our previous works we have shown that the oligonucleotides 5'-GGGGAGGGGGAGG-3' and 5'-GGAGGGGGAGGGG-3' give very stable and specific triplexes with their target double stranded DNAs [Svinarchuk, F., Bertrand, J.-R. and Malvy, C. (1994) Nucleic Acids Res., 22, 3742-3747; Svinarchuk, F., Paoletti, J. and Malvy, C. (1995) J. Biol. Chem., 270, 14 068-14,071]. The target for the invariable part of these oligonucleotides, 5'-GGAGGGGGAGG-3', is found in a highly conserved 20 bp long purine/pyrimidine tract of the vpx gene of the SIV and HIV-2 viruses and could be a target for oligonucleotide directed antivirus therapy. Here were report on the ability of four purine oligonucleotides with different lengths (11-, 14-, 17- and 20-mer) to form triplexes with the purine/pyrimidine stretch of the vpx gene. Triplex formation was tested by joint dimethyl sulfate (DMS) footprint, gel-retardation assay, circular dichroism (CD) and UV-melting studies. Dimethyl sulfate footprint studies revealed the antiparallel orientation of the third strand to the purine strand of the Watson-Crick duplex. However, the protection of the guanines at the ends of the target sequence decreased as the length of the third strand oligonucleotide increased. Melting temperature studies provided profiles with only one transition for all of the triplexes. The melting temperatures of the triplexes were found to be the same as for the targeted duplex in the case of the 11- and 14-mer third strands while for the 17- and 20-mer third strands the melting temperature of the triplexes were correspondingly 4 and 8 degrees C higher than for the duplex. Heating and cooling melting curves were reversible for all of the tested triplexes except one with the 20-mer third strand oligonucleotide. Circular dichroism spectra showed the ability of the target DNA to adopt an A-like DNA conformation. Upon triplex formation the A-DNA form becomes even more pronounced. This effect depends on the length of the third strand oligonucleotide: the CD spectrum shows a 'classical' A-DNA shape with the 20-mer. This is not observed with the purine/pyrimidine stretch of the HIV-1 DNA which keeps a B-like spectrum even after triplex formation. We suggest, that an A-like duplex DNA is required for the formation of a stable DNA purine(purine-pyrimidine) triplex.
Full text
PDF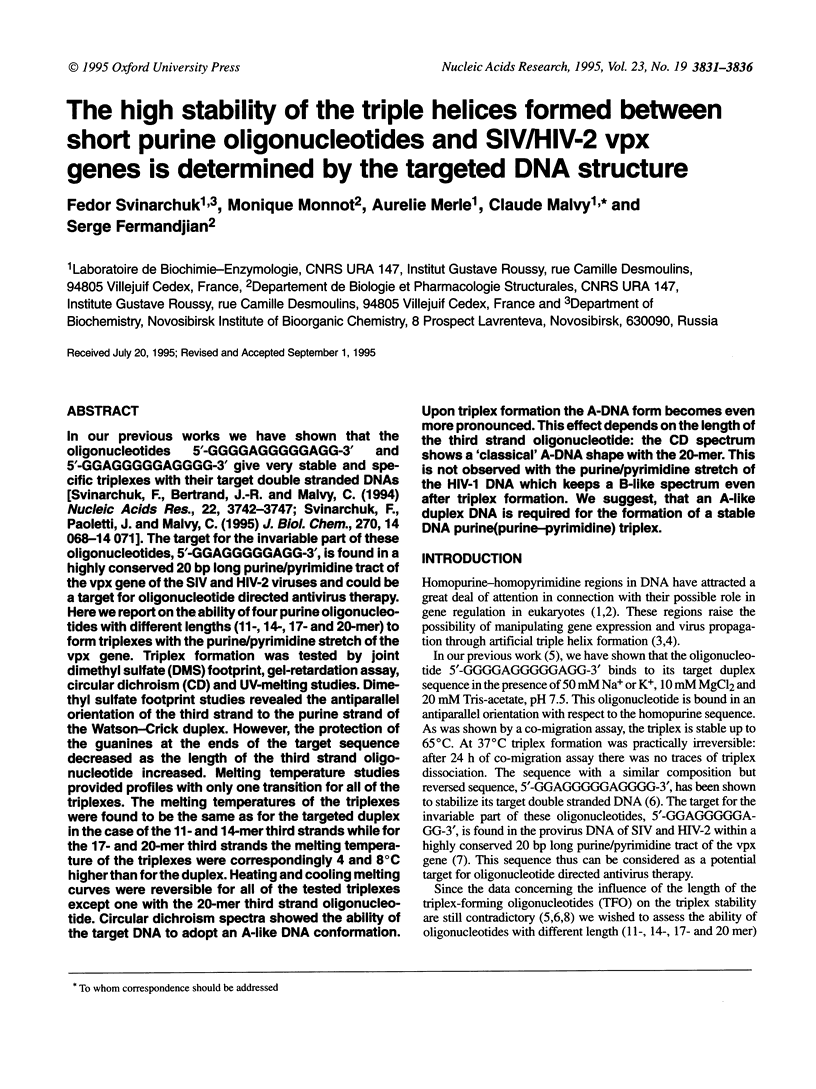
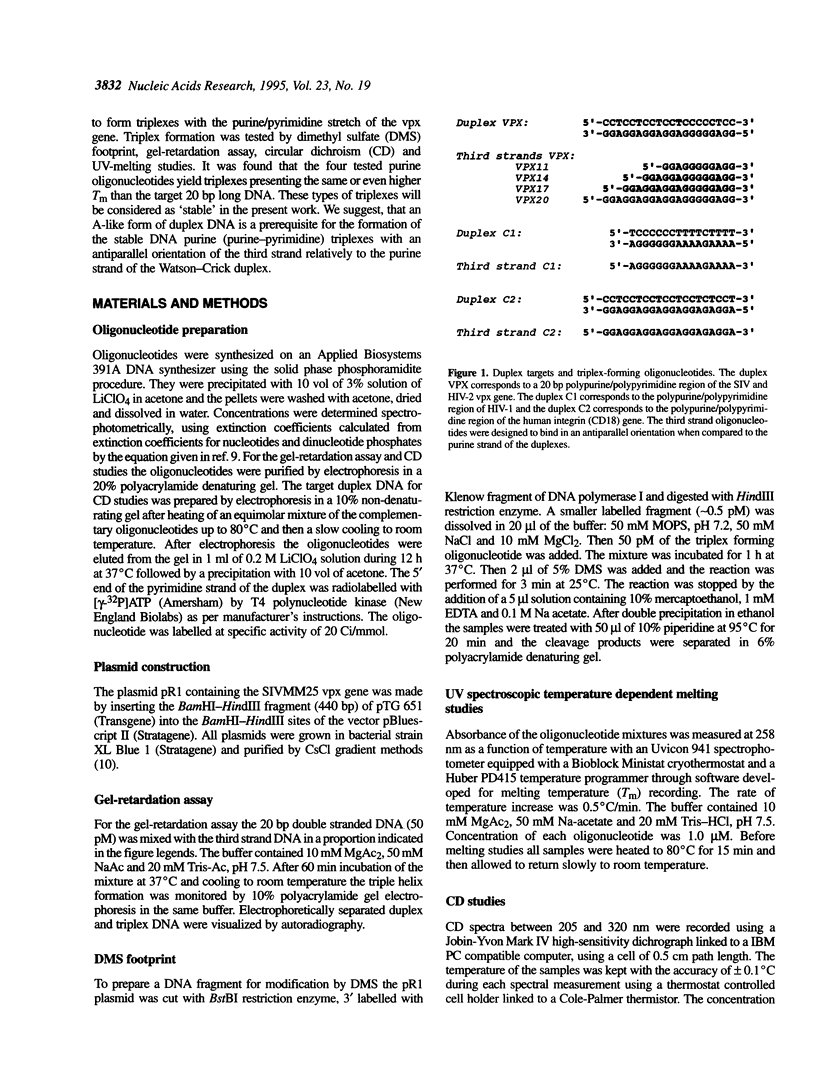
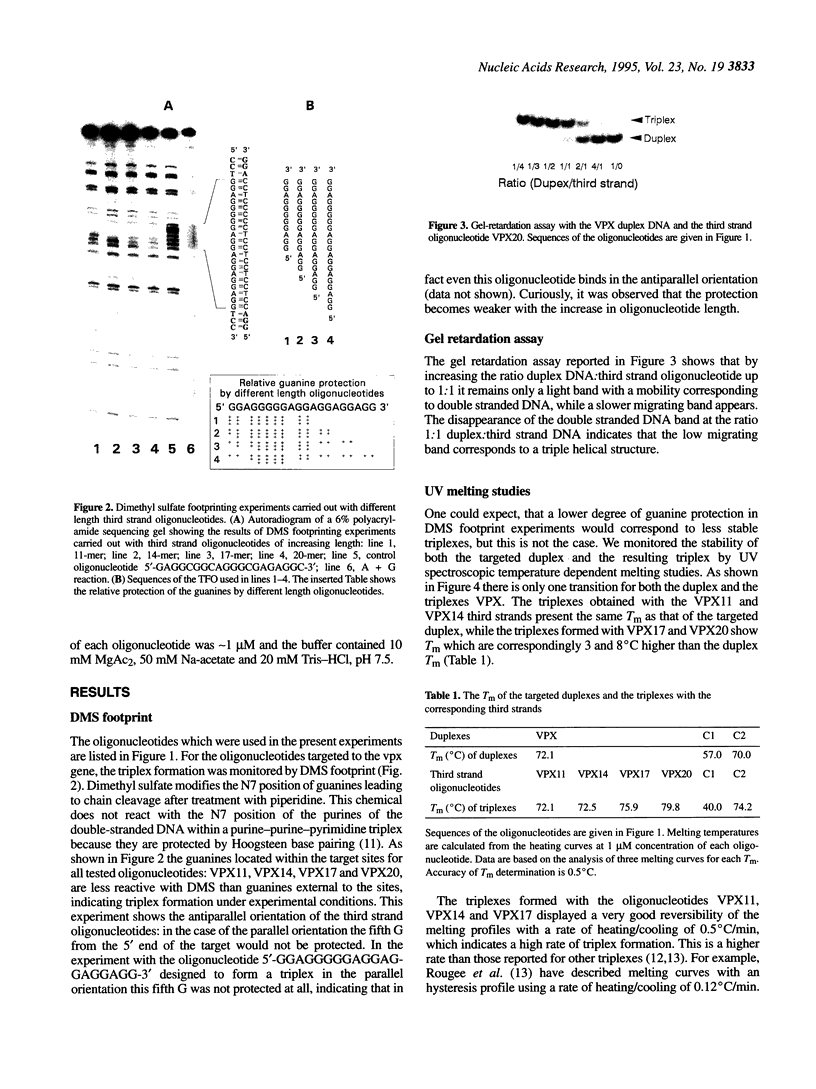
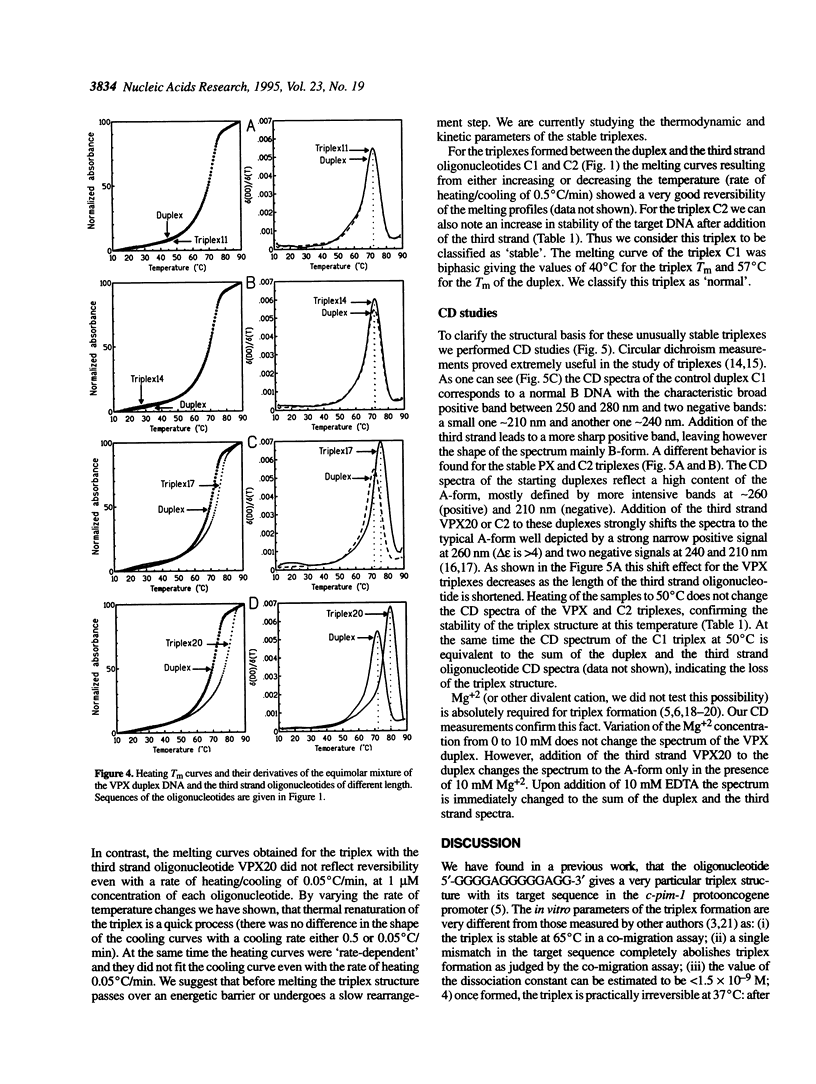
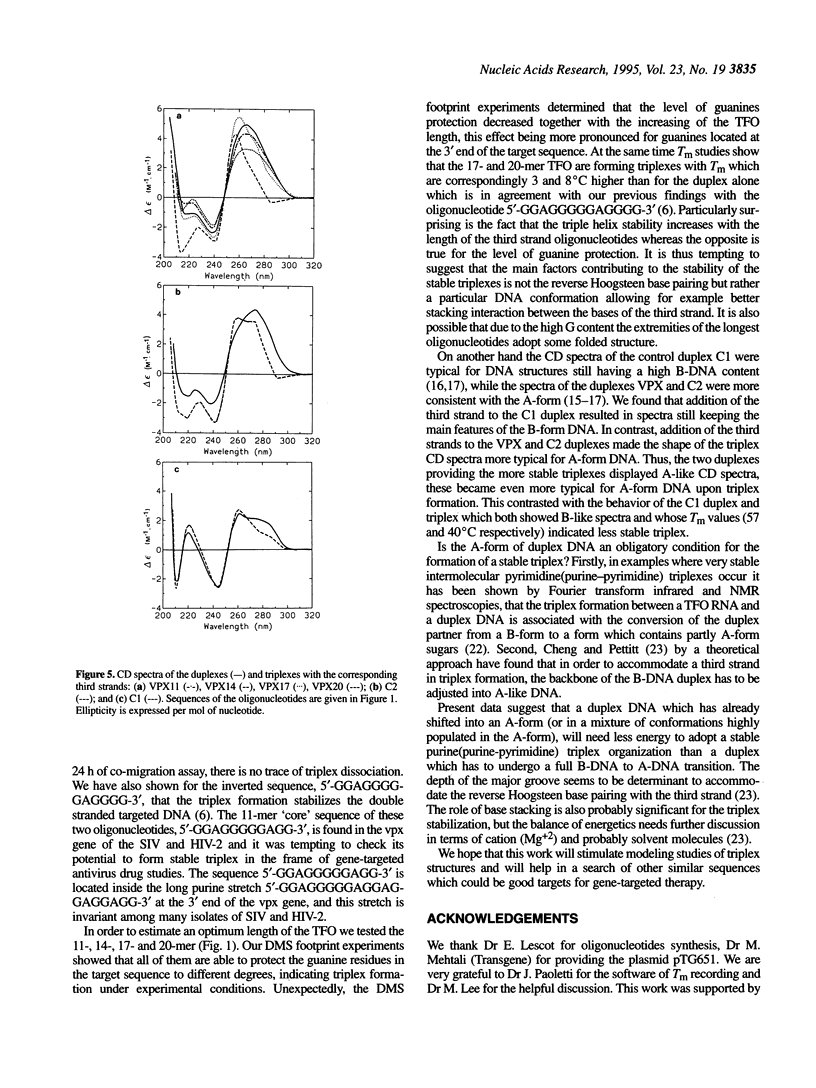
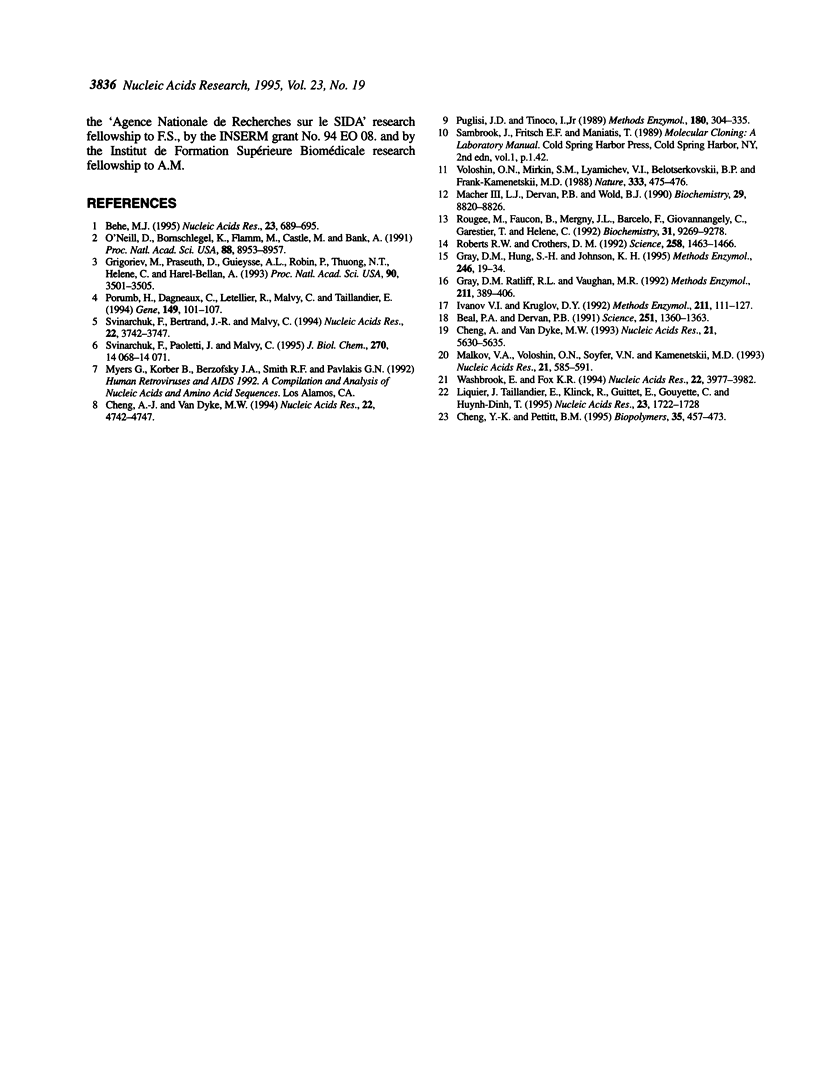
Images in this article
Selected References
These references are in PubMed. This may not be the complete list of references from this article.
- Beal P. A., Dervan P. B. Second structural motif for recognition of DNA by oligonucleotide-directed triple-helix formation. Science. 1991 Mar 15;251(4999):1360–1363. doi: 10.1126/science.2003222. [DOI] [PubMed] [Google Scholar]
- Behe M. J. An overabundance of long oligopurine tracts occurs in the genome of simple and complex eukaryotes. Nucleic Acids Res. 1995 Feb 25;23(4):689–695. doi: 10.1093/nar/23.4.689. [DOI] [PMC free article] [PubMed] [Google Scholar]
- Cheng A. J., Van Dyke M. W. Monovalent cation effects on intermolecular purine-purine-pyrimidine triple-helix formation. Nucleic Acids Res. 1993 Dec 11;21(24):5630–5635. doi: 10.1093/nar/21.24.5630. [DOI] [PMC free article] [PubMed] [Google Scholar]
- Cheng A. J., Van Dyke M. W. Oligodeoxyribonucleotide length and sequence effects on intermolecular purine-purine-pyrimidine triple-helix formation. Nucleic Acids Res. 1994 Nov 11;22(22):4742–4747. doi: 10.1093/nar/22.22.4742. [DOI] [PMC free article] [PubMed] [Google Scholar]
- Cheng Y. K., Pettitt B. M. Solvent effects on model d(CG.G)7 and d(TA.T)7 DNA triple helices. Biopolymers. 1995 May;35(5):457–473. doi: 10.1002/bip.360350505. [DOI] [PubMed] [Google Scholar]
- Gray D. M., Hung S. H., Johnson K. H. Absorption and circular dichroism spectroscopy of nucleic acid duplexes and triplexes. Methods Enzymol. 1995;246:19–34. doi: 10.1016/0076-6879(95)46005-5. [DOI] [PubMed] [Google Scholar]
- Gray D. M., Ratliff R. L., Vaughan M. R. Circular dichroism spectroscopy of DNA. Methods Enzymol. 1992;211:389–406. doi: 10.1016/0076-6879(92)11021-a. [DOI] [PubMed] [Google Scholar]
- Grigoriev M., Praseuth D., Guieysse A. L., Robin P., Thuong N. T., Hélène C., Harel-Bellan A. Inhibition of gene expression by triple helix-directed DNA cross-linking at specific sites. Proc Natl Acad Sci U S A. 1993 Apr 15;90(8):3501–3505. doi: 10.1073/pnas.90.8.3501. [DOI] [PMC free article] [PubMed] [Google Scholar]
- Ivanov V. I., Krylov DYu A-DNA in solution as studied by diverse approaches. Methods Enzymol. 1992;211:111–127. doi: 10.1016/0076-6879(92)11008-7. [DOI] [PubMed] [Google Scholar]
- Liquier J., Taillandier E., Klinck R., Guittet E., Gouyette C., Huynh-Dinh T. Spectroscopic studies of chimeric DNA-RNA and RNA 29-base intramolecular triple helices. Nucleic Acids Res. 1995 May 25;23(10):1722–1728. doi: 10.1093/nar/23.10.1722. [DOI] [PMC free article] [PubMed] [Google Scholar]
- Maher L. J., 3rd, Dervan P. B., Wold B. J. Kinetic analysis of oligodeoxyribonucleotide-directed triple-helix formation on DNA. Biochemistry. 1990 Sep 18;29(37):8820–8826. doi: 10.1021/bi00489a045. [DOI] [PubMed] [Google Scholar]
- Malkov V. A., Voloshin O. N., Soyfer V. N., Frank-Kamenetskii M. D. Cation and sequence effects on stability of intermolecular pyrimidine-purine-purine triplex. Nucleic Acids Res. 1993 Feb 11;21(3):585–591. doi: 10.1093/nar/21.3.585. [DOI] [PMC free article] [PubMed] [Google Scholar]
- O'Neill D., Bornschlegel K., Flamm M., Castle M., Bank A. A DNA-binding factor in adult hematopoietic cells interacts with a pyrimidine-rich domain upstream from the human delta-globin gene. Proc Natl Acad Sci U S A. 1991 Oct 15;88(20):8953–8957. doi: 10.1073/pnas.88.20.8953. [DOI] [PMC free article] [PubMed] [Google Scholar]
- Porumb H., Dagneaux C., Letellier R., Malvy C., Taillandier E. Triple-helices targeted to the polypurine tract of a murine retrovirus. Gene. 1994 Nov 4;149(1):101–107. doi: 10.1016/0378-1119(94)90417-0. [DOI] [PubMed] [Google Scholar]
- Puglisi J. D., Tinoco I., Jr Absorbance melting curves of RNA. Methods Enzymol. 1989;180:304–325. doi: 10.1016/0076-6879(89)80108-9. [DOI] [PubMed] [Google Scholar]
- Roberts R. W., Crothers D. M. Stability and properties of double and triple helices: dramatic effects of RNA or DNA backbone composition. Science. 1992 Nov 27;258(5087):1463–1466. doi: 10.1126/science.1279808. [DOI] [PubMed] [Google Scholar]
- Rougée M., Faucon B., Mergny J. L., Barcelo F., Giovannangeli C., Garestier T., Hélène C. Kinetics and thermodynamics of triple-helix formation: effects of ionic strength and mismatches. Biochemistry. 1992 Sep 29;31(38):9269–9278. doi: 10.1021/bi00153a021. [DOI] [PubMed] [Google Scholar]
- Svinarchuk F., Bertrand J. R., Malvy C. A short purine oligonucleotide forms a highly stable triple helix with the promoter of the murine c-pim-1 proto-oncogene. Nucleic Acids Res. 1994 Sep 11;22(18):3742–3747. doi: 10.1093/nar/22.18.3742. [DOI] [PMC free article] [PubMed] [Google Scholar]
- Voloshin O. N., Mirkin S. M., Lyamichev V. I., Belotserkovskii B. P., Frank-Kamenetskii M. D. Chemical probing of homopurine-homopyrimidine mirror repeats in supercoiled DNA. Nature. 1988 Jun 2;333(6172):475–476. doi: 10.1038/333475a0. [DOI] [PubMed] [Google Scholar]
- Washbrook E., Fox K. R. Comparison of antiparallel A.AT and T.AT triplets within an alternate strand DNA triple helix. Nucleic Acids Res. 1994 Sep 25;22(19):3977–3982. doi: 10.1093/nar/22.19.3977. [DOI] [PMC free article] [PubMed] [Google Scholar]