Abstract
The proposed mechanism for DNA (cytosine-5)-methyltransferases envisions a key role for a cysteine residue. It is expected to form a covalent link with carbon 6 of the target cytosine, activating the normally inactive carbon 5 for methyl transfer. There is a single conserved cysteine among all DNA (cytosine-5)-methyltransferases making it the candidate nucleophile. We have changed this cysteine to other amino acids for the EcoRII methylase; which methylates the second cytosine in the sequence 5'-CCWGG-3'. Mutants were tested for their methyl transferring ability and for their ability to form covalent complexes with DNA. The latter property was tested indirectly with the use of a genetic assay involving sensitivity of cells to 5-azacytidine. Replacement of the conserved cysteine with glycine, valine, tryptophan or serine led to an apparent loss of methyl transferring ability. Interestingly, cells carrying the mutant with serine did show sensitivity to 5-azacytidine, suggesting the ability to link to DNA. Unexpectedly, substitution of the cysteine with glycine results in the inhibition of cell growth and the mutant allele can be maintained in the cells only when it is poorly expressed. These results suggest that the conserved cysteine in the EcoRII methylase is essential for methylase action and it may play more than one role in it.
Full text
PDF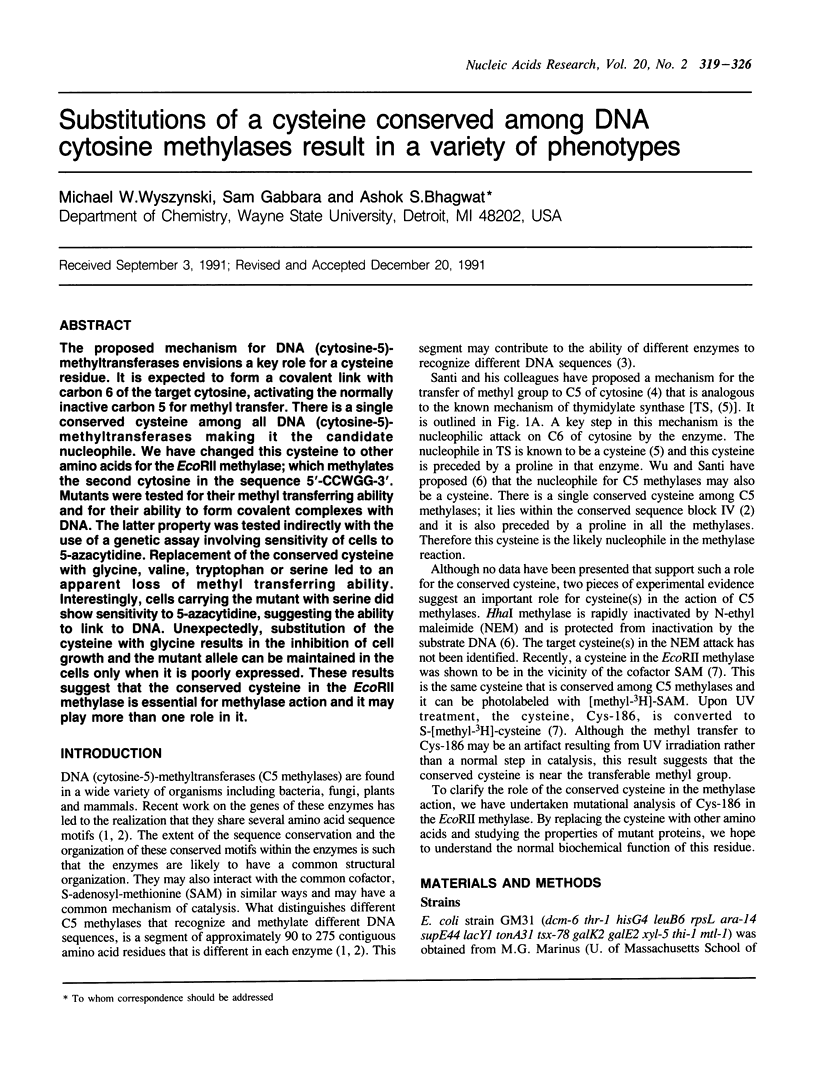
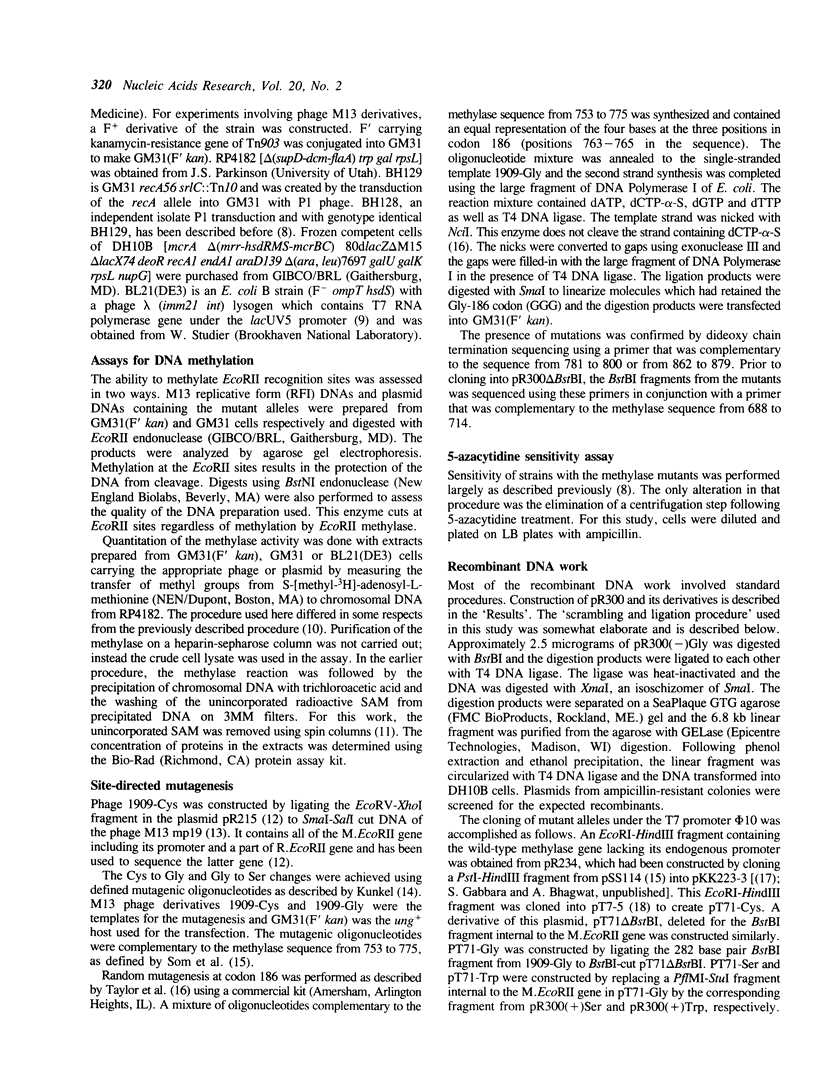
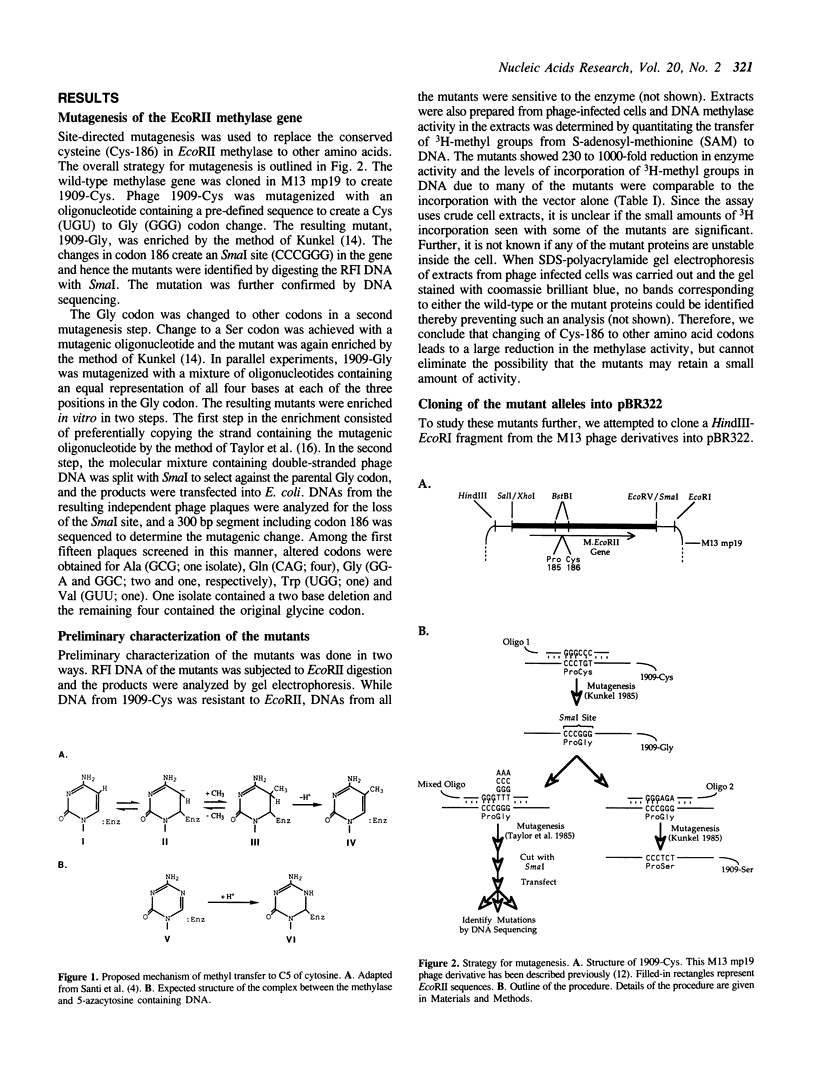
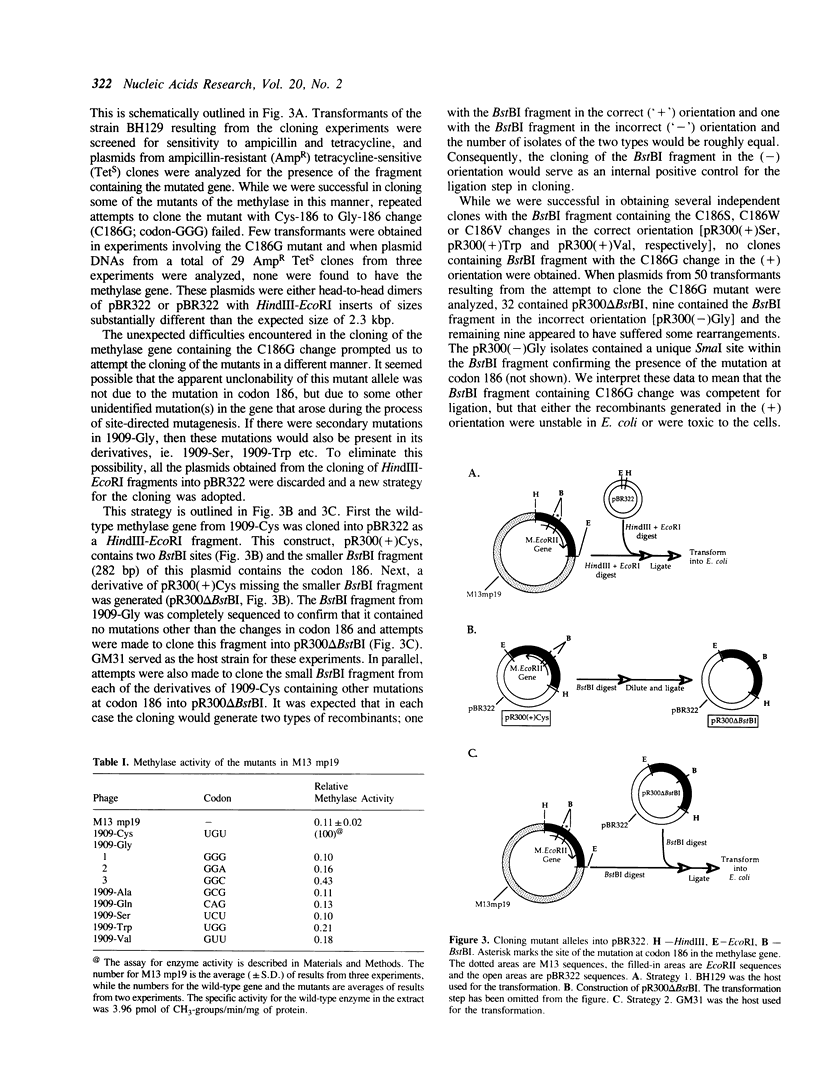
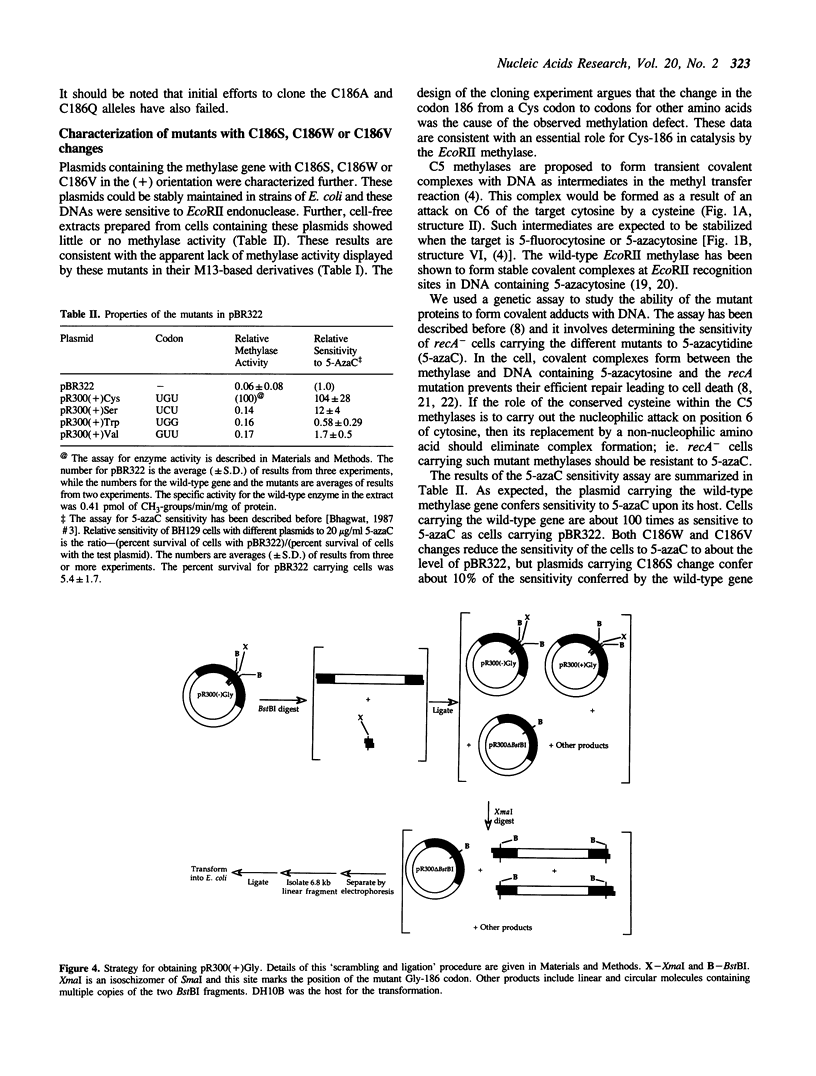
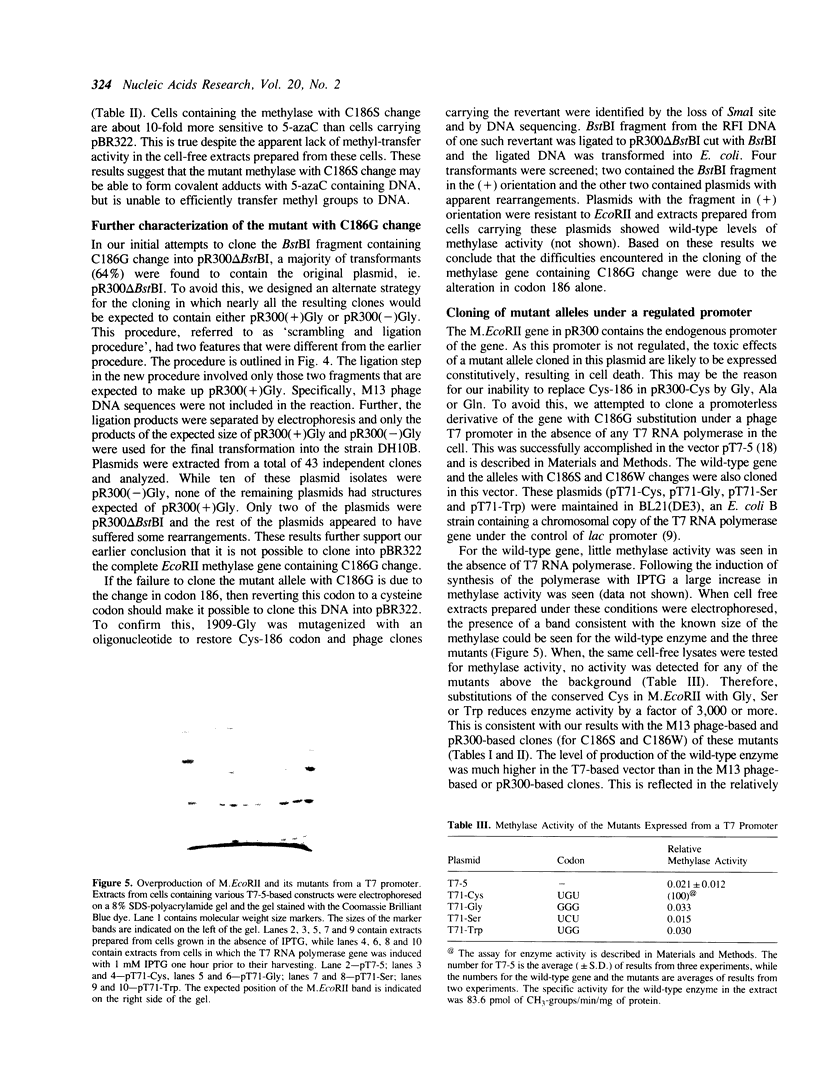
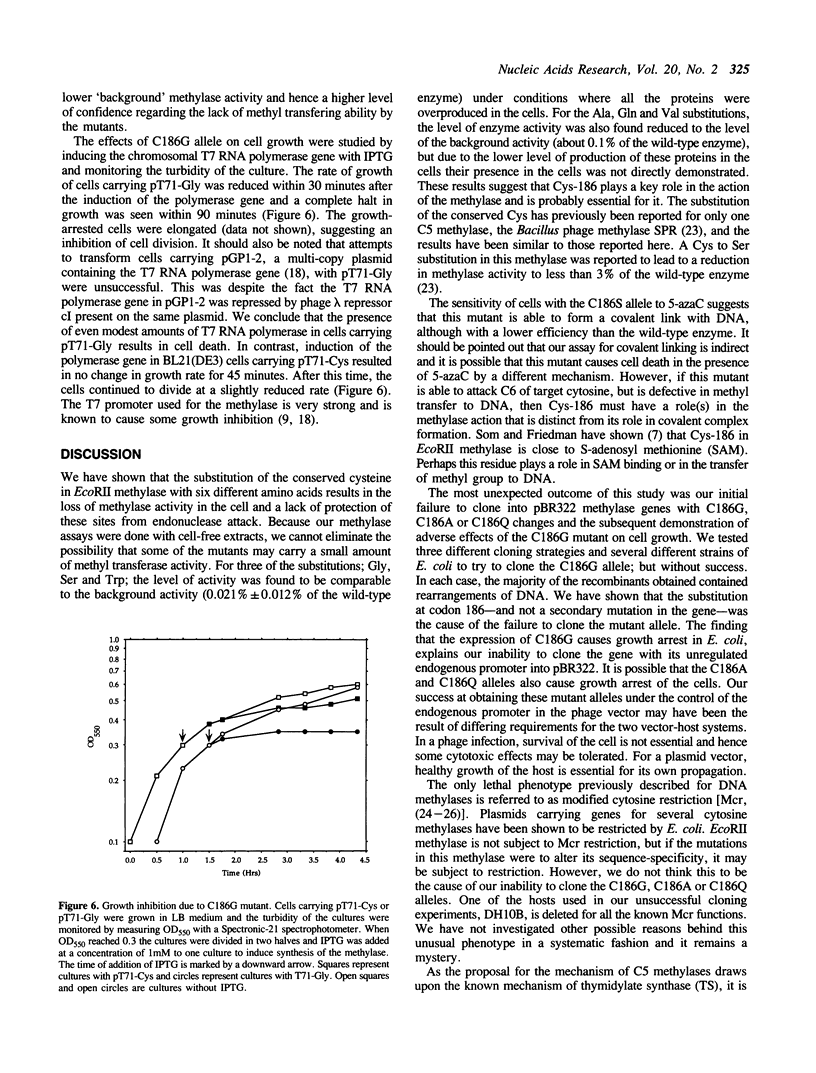
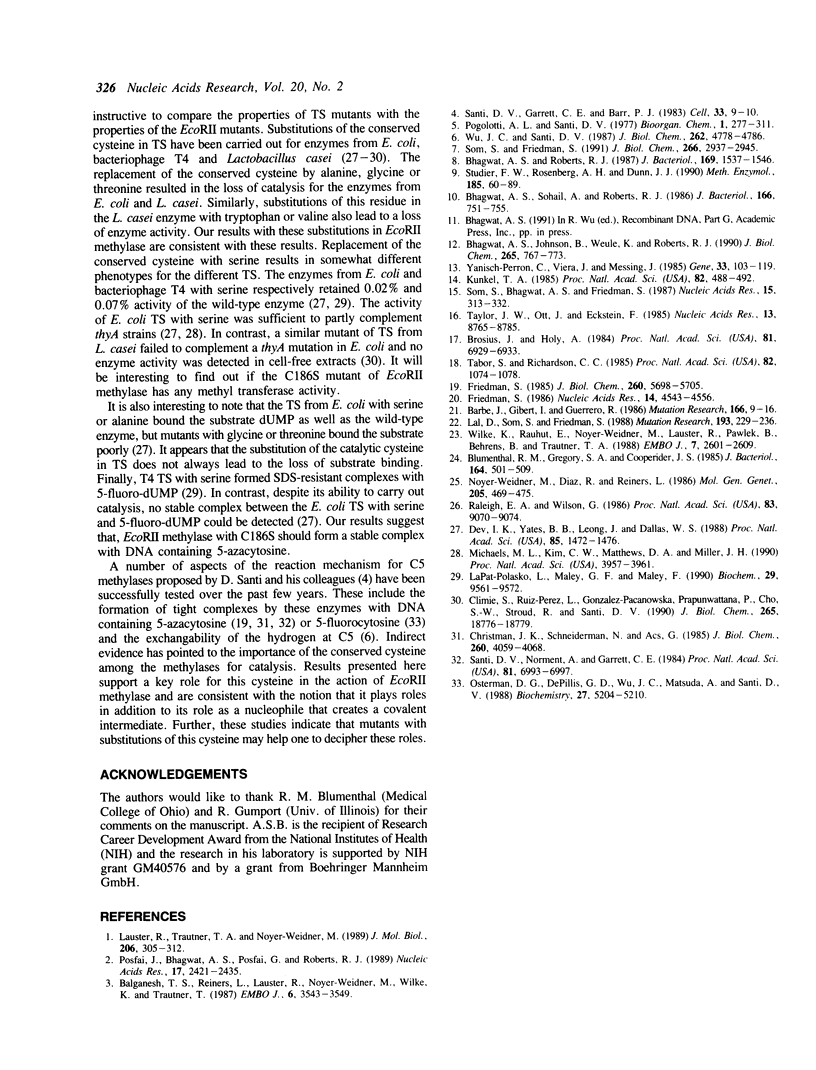
Images in this article
Selected References
These references are in PubMed. This may not be the complete list of references from this article.
- Balganesh T. S., Reiners L., Lauster R., Noyer-Weidner M., Wilke K., Trautner T. A. Construction and use of chimeric SPR/phi 3T DNA methyltransferases in the definition of sequence recognizing enzyme regions. EMBO J. 1987 Nov;6(11):3543–3549. doi: 10.1002/j.1460-2075.1987.tb02681.x. [DOI] [PMC free article] [PubMed] [Google Scholar]
- Barbé J., Gibert I., Guerrero R. 5-Azacytidine: survival and induction of the SOS response in Escherichia coli K-12. Mutat Res. 1986 Jul;166(1):9–16. doi: 10.1016/0167-8817(86)90035-0. [DOI] [PubMed] [Google Scholar]
- Bhagwat A. S., Johnson B., Weule K., Roberts R. J. Primary sequence of the EcoRII endonuclease and properties of its fusions with beta-galactosidase. J Biol Chem. 1990 Jan 15;265(2):767–773. [PubMed] [Google Scholar]
- Bhagwat A. S., Roberts R. J. Genetic analysis of the 5-azacytidine sensitivity of Escherichia coli K-12. J Bacteriol. 1987 Apr;169(4):1537–1546. doi: 10.1128/jb.169.4.1537-1546.1987. [DOI] [PMC free article] [PubMed] [Google Scholar]
- Bhagwat A. S., Sohail A., Roberts R. J. Cloning and characterization of the dcm locus of Escherichia coli K-12. J Bacteriol. 1986 Jun;166(3):751–755. doi: 10.1128/jb.166.3.751-755.1986. [DOI] [PMC free article] [PubMed] [Google Scholar]
- Blumenthal R. M., Gregory S. A., Cooperider J. S. Cloning of a restriction-modification system from Proteus vulgaris and its use in analyzing a methylase-sensitive phenotype in Escherichia coli. J Bacteriol. 1985 Nov;164(2):501–509. doi: 10.1128/jb.164.2.501-509.1985. [DOI] [PMC free article] [PubMed] [Google Scholar]
- Brosius J., Holy A. Regulation of ribosomal RNA promoters with a synthetic lac operator. Proc Natl Acad Sci U S A. 1984 Nov;81(22):6929–6933. doi: 10.1073/pnas.81.22.6929. [DOI] [PMC free article] [PubMed] [Google Scholar]
- Christman J. K., Schneiderman N., Acs G. Formation of highly stable complexes between 5-azacytosine-substituted DNA and specific non-histone nuclear proteins. Implications for 5-azacytidine-mediated effects on DNA methylation and gene expression. J Biol Chem. 1985 Apr 10;260(7):4059–4068. [PubMed] [Google Scholar]
- Climie S., Ruiz-Perez L., Gonzalez-Pacanowska D., Prapunwattana P., Cho S. W., Stroud R., Santi D. V. Saturation site-directed mutagenesis of thymidylate synthase. J Biol Chem. 1990 Nov 5;265(31):18776–18779. [PubMed] [Google Scholar]
- Dev I. K., Yates B. B., Leong J., Dallas W. S. Functional role of cysteine-146 in Escherichia coli thymidylate synthase. Proc Natl Acad Sci U S A. 1988 Mar;85(5):1472–1476. doi: 10.1073/pnas.85.5.1472. [DOI] [PMC free article] [PubMed] [Google Scholar]
- Friedman S. Binding of the EcoRII methylase to azacytosine-containing DNA. Nucleic Acids Res. 1986 Jun 11;14(11):4543–4556. doi: 10.1093/nar/14.11.4543. [DOI] [PMC free article] [PubMed] [Google Scholar]
- Friedman S. The irreversible binding of azacytosine-containing DNA fragments to bacterial DNA(cytosine-5)methyltransferases. J Biol Chem. 1985 May 10;260(9):5698–5705. [PubMed] [Google Scholar]
- Kunkel T. A. Rapid and efficient site-specific mutagenesis without phenotypic selection. Proc Natl Acad Sci U S A. 1985 Jan;82(2):488–492. doi: 10.1073/pnas.82.2.488. [DOI] [PMC free article] [PubMed] [Google Scholar]
- LaPat-Polasko L., Maley G. F., Maley F. Properties of bacteriophage T4 thymidylate synthase following mutagenic changes in the active site and folate binding region. Biochemistry. 1990 Oct 16;29(41):9561–9572. doi: 10.1021/bi00493a010. [DOI] [PubMed] [Google Scholar]
- Lal D., Som S., Friedman S. Survival and mutagenic effects of 5-azacytidine in Escherichia coli. Mutat Res. 1988 May;193(3):229–236. doi: 10.1016/0167-8817(88)90033-8. [DOI] [PubMed] [Google Scholar]
- Lauster R., Trautner T. A., Noyer-Weidner M. Cytosine-specific type II DNA methyltransferases. A conserved enzyme core with variable target-recognizing domains. J Mol Biol. 1989 Mar 20;206(2):305–312. doi: 10.1016/0022-2836(89)90480-4. [DOI] [PubMed] [Google Scholar]
- Michaels M. L., Kim C. W., Matthews D. A., Miller J. H. Escherichia coli thymidylate synthase: amino acid substitutions by suppression of amber nonsense mutations. Proc Natl Acad Sci U S A. 1990 May;87(10):3957–3961. doi: 10.1073/pnas.87.10.3957. [DOI] [PMC free article] [PubMed] [Google Scholar]
- Noyer-Weidner M., Diaz R., Reiners L. Cytosine-specific DNA modification interferes with plasmid establishment in Escherichia coli K12: involvement of rglB. Mol Gen Genet. 1986 Dec;205(3):469–475. doi: 10.1007/BF00338084. [DOI] [PubMed] [Google Scholar]
- Osterman D. G., DePillis G. D., Wu J. C., Matsuda A., Santi D. V. 5-Fluorocytosine in DNA is a mechanism-based inhibitor of HhaI methylase. Biochemistry. 1988 Jul 12;27(14):5204–5210. doi: 10.1021/bi00414a039. [DOI] [PubMed] [Google Scholar]
- Pósfai J., Bhagwat A. S., Pósfai G., Roberts R. J. Predictive motifs derived from cytosine methyltransferases. Nucleic Acids Res. 1989 Apr 11;17(7):2421–2435. doi: 10.1093/nar/17.7.2421. [DOI] [PMC free article] [PubMed] [Google Scholar]
- Raleigh E. A., Wilson G. Escherichia coli K-12 restricts DNA containing 5-methylcytosine. Proc Natl Acad Sci U S A. 1986 Dec;83(23):9070–9074. doi: 10.1073/pnas.83.23.9070. [DOI] [PMC free article] [PubMed] [Google Scholar]
- Santi D. V., Garrett C. E., Barr P. J. On the mechanism of inhibition of DNA-cytosine methyltransferases by cytosine analogs. Cell. 1983 May;33(1):9–10. doi: 10.1016/0092-8674(83)90327-6. [DOI] [PubMed] [Google Scholar]
- Santi D. V., Norment A., Garrett C. E. Covalent bond formation between a DNA-cytosine methyltransferase and DNA containing 5-azacytosine. Proc Natl Acad Sci U S A. 1984 Nov;81(22):6993–6997. doi: 10.1073/pnas.81.22.6993. [DOI] [PMC free article] [PubMed] [Google Scholar]
- Som S., Bhagwat A. S., Friedman S. Nucleotide sequence and expression of the gene encoding the EcoRII modification enzyme. Nucleic Acids Res. 1987 Jan 12;15(1):313–332. doi: 10.1093/nar/15.1.313. [DOI] [PMC free article] [PubMed] [Google Scholar]
- Som S., Friedman S. Identification of a highly conserved domain in the EcoRII methyltransferase which can be photolabeled with S-adenosyl-L-[methyl-3H]methionine. Evidence for UV-induced transmethylation of cysteine 186. J Biol Chem. 1991 Feb 15;266(5):2937–2945. [PubMed] [Google Scholar]
- Studier F. W., Rosenberg A. H., Dunn J. J., Dubendorff J. W. Use of T7 RNA polymerase to direct expression of cloned genes. Methods Enzymol. 1990;185:60–89. doi: 10.1016/0076-6879(90)85008-c. [DOI] [PubMed] [Google Scholar]
- Tabor S., Richardson C. C. A bacteriophage T7 RNA polymerase/promoter system for controlled exclusive expression of specific genes. Proc Natl Acad Sci U S A. 1985 Feb;82(4):1074–1078. doi: 10.1073/pnas.82.4.1074. [DOI] [PMC free article] [PubMed] [Google Scholar]
- Taylor J. W., Ott J., Eckstein F. The rapid generation of oligonucleotide-directed mutations at high frequency using phosphorothioate-modified DNA. Nucleic Acids Res. 1985 Dec 20;13(24):8765–8785. doi: 10.1093/nar/13.24.8765. [DOI] [PMC free article] [PubMed] [Google Scholar]
- Wilke K., Rauhut E., Noyer-Weidner M., Lauster R., Pawlek B., Behrens B., Trautner T. A. Sequential order of target-recognizing domains in multispecific DNA-methyltransferases. EMBO J. 1988 Aug;7(8):2601–2609. doi: 10.1002/j.1460-2075.1988.tb03110.x. [DOI] [PMC free article] [PubMed] [Google Scholar]
- Wu J. C., Santi D. V. Kinetic and catalytic mechanism of HhaI methyltransferase. J Biol Chem. 1987 Apr 5;262(10):4778–4786. [PubMed] [Google Scholar]
- Yanisch-Perron C., Vieira J., Messing J. Improved M13 phage cloning vectors and host strains: nucleotide sequences of the M13mp18 and pUC19 vectors. Gene. 1985;33(1):103–119. doi: 10.1016/0378-1119(85)90120-9. [DOI] [PubMed] [Google Scholar]