Abstract
The concept of antigene therapy of disease is based on the ability of an oligonucleotide (the therapeutic agent) to bind to double-stranded genomic DNA (the target associated with the disease). Examples are herein given of the linkage of a series of polyamines to a 21-mer homopyrimidine oligonucleotide. These conjugated 21-mers can each form a triple helix with an appropriate double-stranded homopurine-homopyrimidine DNA according to Hoogsteen base-pairing rules. No triple helix was found when unmodified third strand was used at 10 mM sodium phosphate, pH 6.5, 100 mM sodium chloride solution. In contrast, the spermine-conjugated oligonucleotide had a melting temperature of 42 degrees C. According to the melting profile, the appended spermine moiety was found to affect the Tm only of the triple helix, but not of the subsequent melting of the underlying double helix. The Tm enhancing ability of the spermine-conjugate was found to be better than that of other polyamine-conjugates.
Full text
PDF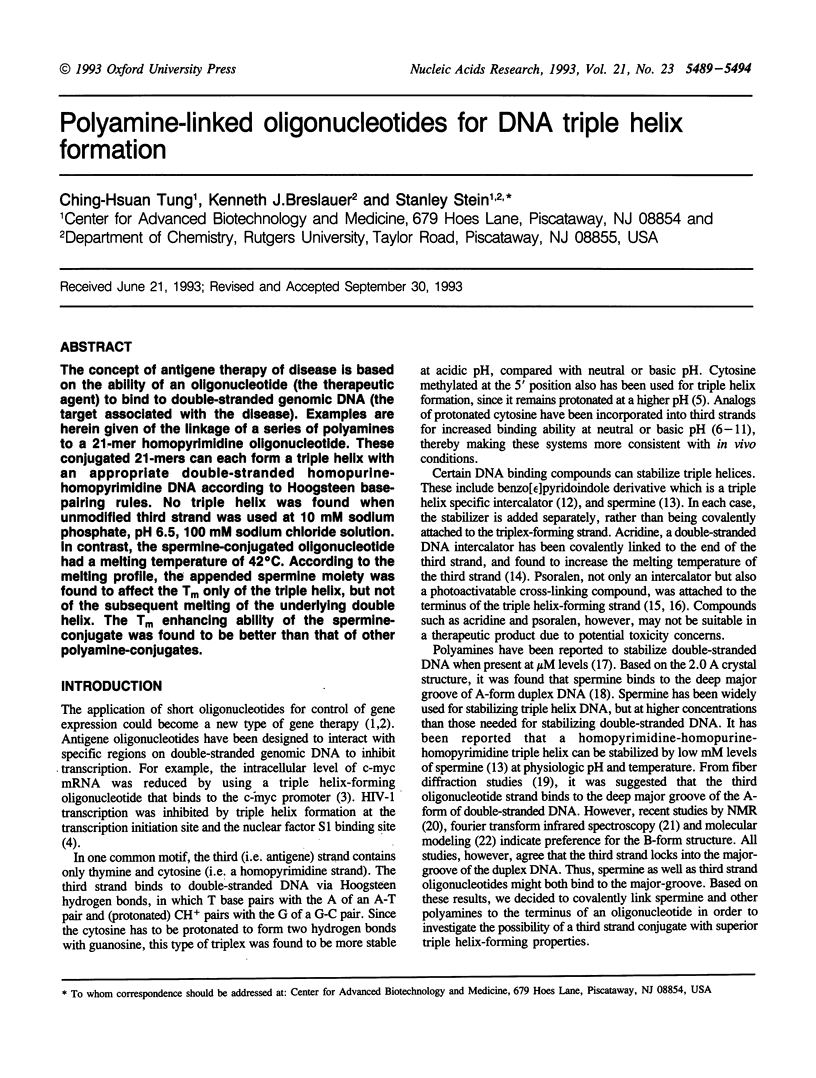
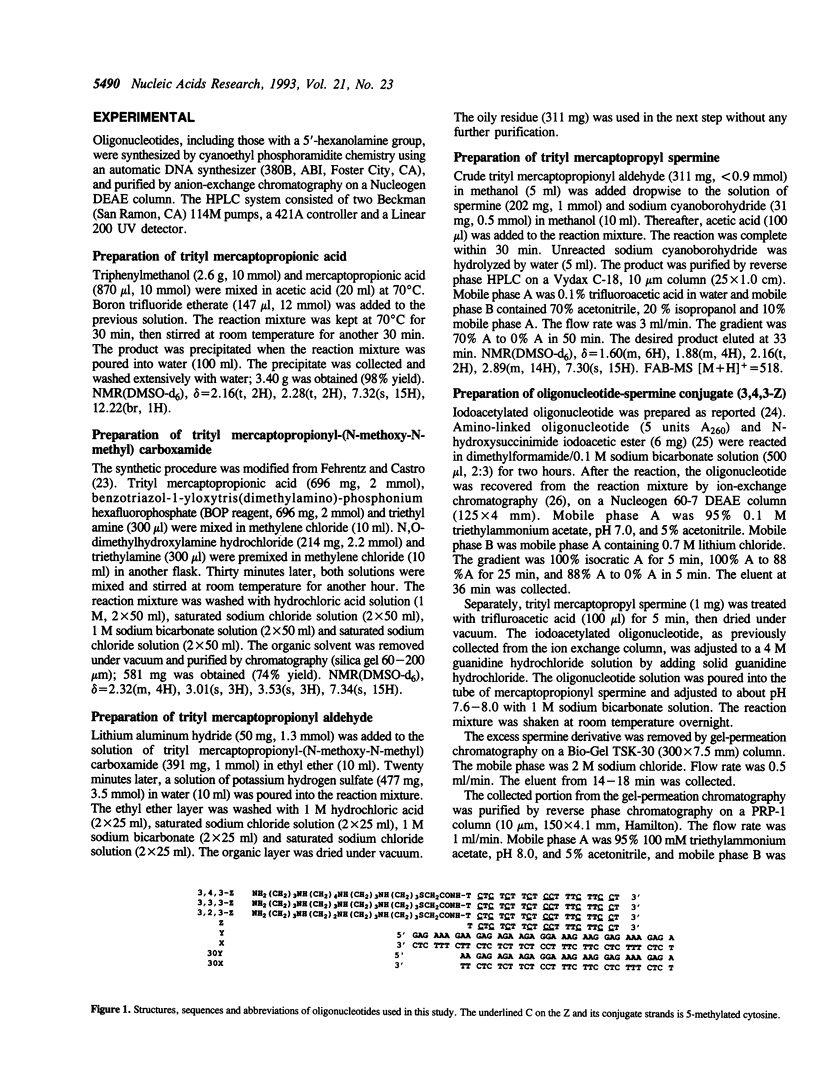
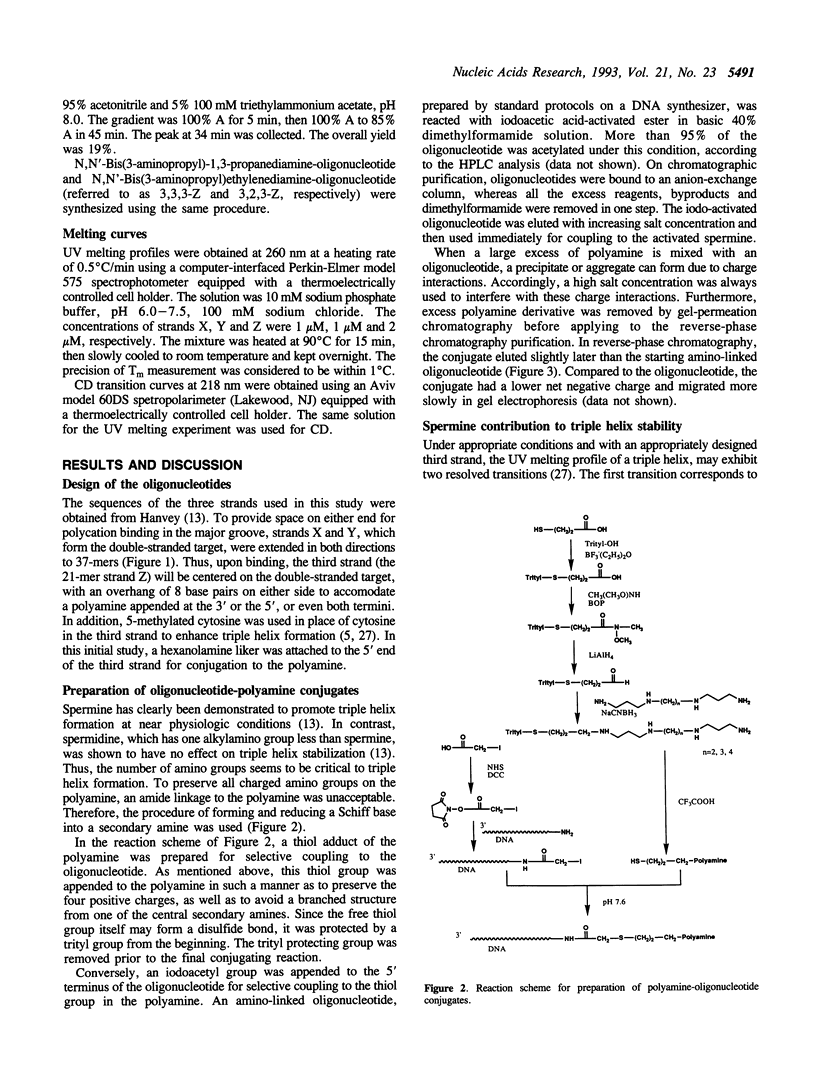
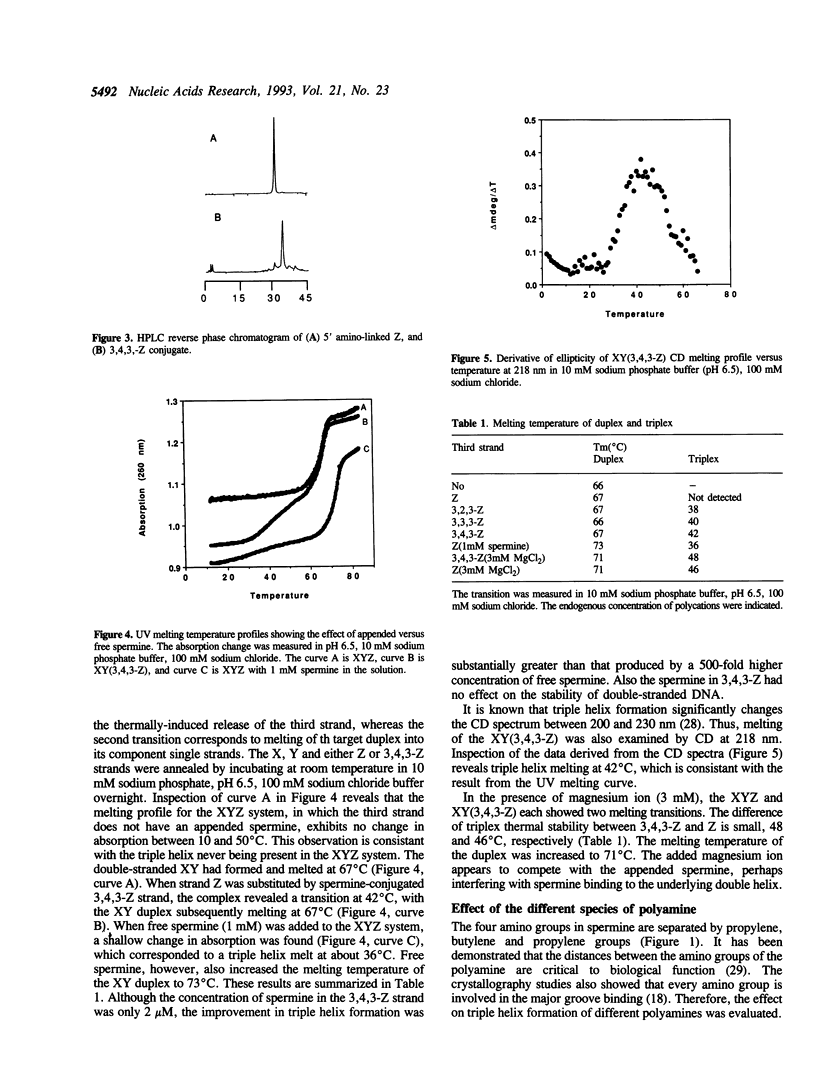
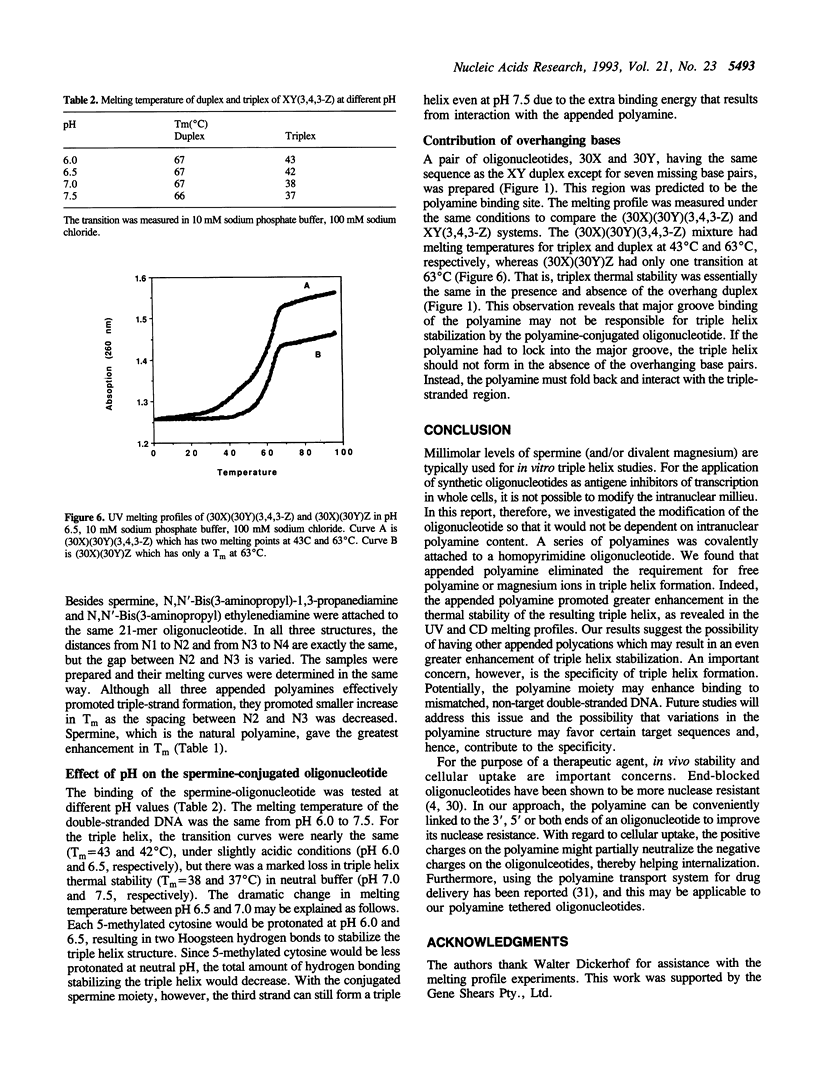
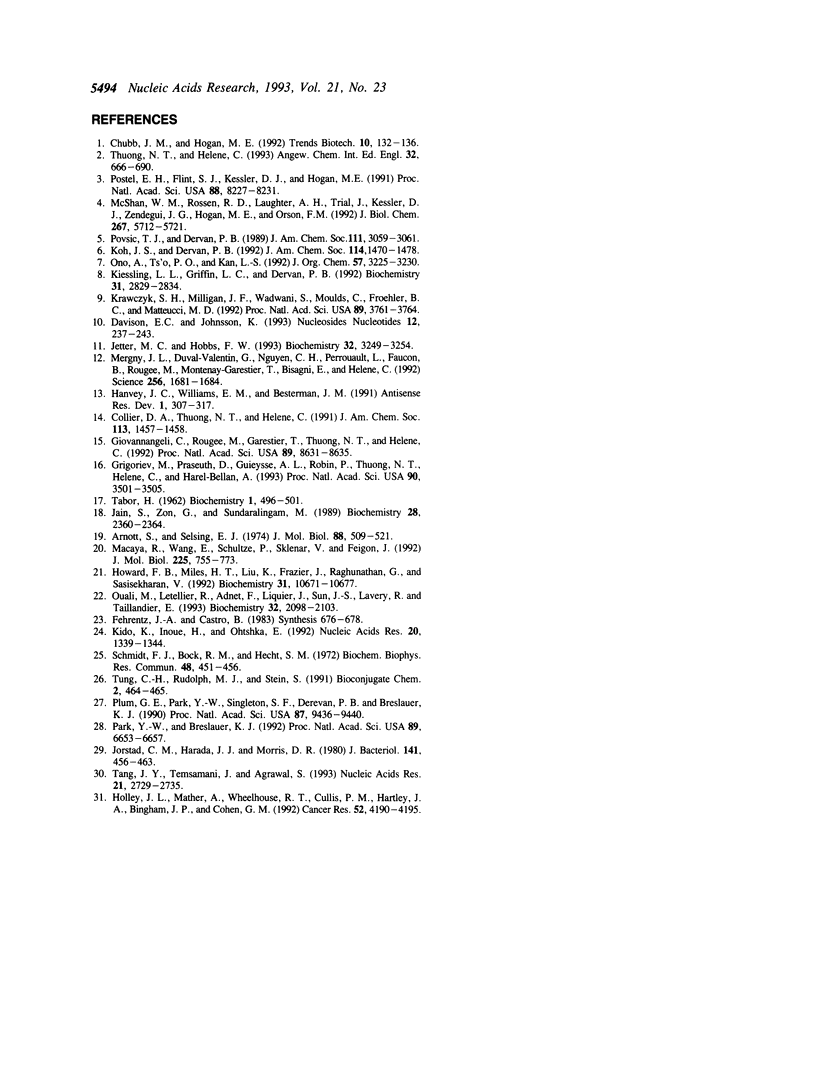
Selected References
These references are in PubMed. This may not be the complete list of references from this article.
- Arnott S., Selsing E. Structures for the polynucleotide complexes poly(dA) with poly (dT) and poly(dT) with poly(dA) with poly (dT). J Mol Biol. 1974 Sep 15;88(2):509–521. doi: 10.1016/0022-2836(74)90498-7. [DOI] [PubMed] [Google Scholar]
- Chubb J. M., Hogan M. E. Human therapeutics based on triple helix technology. Trends Biotechnol. 1992 Apr;10(4):132–136. doi: 10.1016/0167-7799(92)90195-2. [DOI] [PubMed] [Google Scholar]
- Giovannangéli C., Rougée M., Garestier T., Thuong N. T., Hélène C. Triple-helix formation by oligonucleotides containing the three bases thymine, cytosine, and guanine. Proc Natl Acad Sci U S A. 1992 Sep 15;89(18):8631–8635. doi: 10.1073/pnas.89.18.8631. [DOI] [PMC free article] [PubMed] [Google Scholar]
- Grigoriev M., Praseuth D., Guieysse A. L., Robin P., Thuong N. T., Hélène C., Harel-Bellan A. Inhibition of gene expression by triple helix-directed DNA cross-linking at specific sites. Proc Natl Acad Sci U S A. 1993 Apr 15;90(8):3501–3505. doi: 10.1073/pnas.90.8.3501. [DOI] [PMC free article] [PubMed] [Google Scholar]
- Hanvey J. C., Williams E. M., Besterman J. M. DNA triple-helix formation at physiologic pH and temperature. Antisense Res Dev. 1991 Winter;1(4):307–317. doi: 10.1089/ard.1991.1.307. [DOI] [PubMed] [Google Scholar]
- Holley J. L., Mather A., Wheelhouse R. T., Cullis P. M., Hartley J. A., Bingham J. P., Cohen G. M. Targeting of tumor cells and DNA by a chlorambucil-spermidine conjugate. Cancer Res. 1992 Aug 1;52(15):4190–4195. [PubMed] [Google Scholar]
- Howard F. B., Miles H. T., Liu K., Frazier J., Raghunathan G., Sasisekharan V. Structure of d(T)n.d(A)n.d(T)n: the DNA triple helix has B-form geometry with C2'-endo sugar pucker. Biochemistry. 1992 Nov 10;31(44):10671–10677. doi: 10.1021/bi00159a005. [DOI] [PubMed] [Google Scholar]
- Jain S., Zon G., Sundaralingam M. Base only binding of spermine in the deep groove of the A-DNA octamer d(GTGTACAC). Biochemistry. 1989 Mar 21;28(6):2360–2364. doi: 10.1021/bi00432a002. [DOI] [PubMed] [Google Scholar]
- Jetter M. C., Hobbs F. W. 7,8-Dihydro-8-oxoadenine as a replacement for cytosine in the third strand of triple helices. Triplex formation without hypochromicity. Biochemistry. 1993 Apr 6;32(13):3249–3254. doi: 10.1021/bi00064a006. [DOI] [PubMed] [Google Scholar]
- Jorstad C. M., Harada J. J., Morris D. R. Structural specificity of the spermidine requirement of an Escherichia coli auxotroph. J Bacteriol. 1980 Feb;141(2):456–463. doi: 10.1128/jb.141.2.456-463.1980. [DOI] [PMC free article] [PubMed] [Google Scholar]
- Kido K., Inoue H., Ohtsuka E. Sequence-dependent cleavage of DNA by alkylation with antisense oligodeoxyribonucleotides containing a 2-(N-iodoacetylaminoethyl)thio-adenine. Nucleic Acids Res. 1992 Mar 25;20(6):1339–1344. doi: 10.1093/nar/20.6.1339. [DOI] [PMC free article] [PubMed] [Google Scholar]
- Kiessling L. L., Griffin L. C., Dervan P. B. Flanking sequence effects within the pyrimidine triple-helix motif characterized by affinity cleaving. Biochemistry. 1992 Mar 17;31(10):2829–2834. doi: 10.1021/bi00125a026. [DOI] [PubMed] [Google Scholar]
- Krawczyk S. H., Milligan J. F., Wadwani S., Moulds C., Froehler B. C., Matteucci M. D. Oligonucleotide-mediated triple helix formation using an N3-protonated deoxycytidine analog exhibiting pH-independent binding within the physiological range. Proc Natl Acad Sci U S A. 1992 May 1;89(9):3761–3764. doi: 10.1073/pnas.89.9.3761. [DOI] [PMC free article] [PubMed] [Google Scholar]
- Macaya R., Wang E., Schultze P., Sklenár V., Feigon J. Proton nuclear magnetic resonance assignments and structural characterization of an intramolecular DNA triplex. J Mol Biol. 1992 Jun 5;225(3):755–773. doi: 10.1016/0022-2836(92)90399-5. [DOI] [PubMed] [Google Scholar]
- McShan W. M., Rossen R. D., Laughter A. H., Trial J., Kessler D. J., Zendegui J. G., Hogan M. E., Orson F. M. Inhibition of transcription of HIV-1 in infected human cells by oligodeoxynucleotides designed to form DNA triple helices. J Biol Chem. 1992 Mar 15;267(8):5712–5721. [PubMed] [Google Scholar]
- Mergny J. L., Duval-Valentin G., Nguyen C. H., Perrouault L., Faucon B., Rougée M., Montenay-Garestier T., Bisagni E., Hélène C. Triple helix-specific ligands. Science. 1992 Jun 19;256(5064):1681–1684. doi: 10.1126/science.256.5064.1681. [DOI] [PubMed] [Google Scholar]
- Ouali M., Letellier R., Adnet F., Liquier J., Sun J. S., Lavery R., Taillandier E. A possible family of B-like triple helix structures: comparison with the Arnott A-like triple helix. Biochemistry. 1993 Mar 2;32(8):2098–2103. doi: 10.1021/bi00059a030. [DOI] [PubMed] [Google Scholar]
- Park Y. W., Breslauer K. J. Drug binding to higher ordered DNA structures: netropsin complexation with a nucleic acid triple helix. Proc Natl Acad Sci U S A. 1992 Jul 15;89(14):6653–6657. doi: 10.1073/pnas.89.14.6653. [DOI] [PMC free article] [PubMed] [Google Scholar]
- Plum G. E., Park Y. W., Singleton S. F., Dervan P. B., Breslauer K. J. Thermodynamic characterization of the stability and the melting behavior of a DNA triplex: a spectroscopic and calorimetric study. Proc Natl Acad Sci U S A. 1990 Dec;87(23):9436–9440. doi: 10.1073/pnas.87.23.9436. [DOI] [PMC free article] [PubMed] [Google Scholar]
- Postel E. H., Flint S. J., Kessler D. J., Hogan M. E. Evidence that a triplex-forming oligodeoxyribonucleotide binds to the c-myc promoter in HeLa cells, thereby reducing c-myc mRNA levels. Proc Natl Acad Sci U S A. 1991 Sep 15;88(18):8227–8231. doi: 10.1073/pnas.88.18.8227. [DOI] [PMC free article] [PubMed] [Google Scholar]
- Schmidt F. J., Bock R. M., Hecht S. M. Chemical modifications of transfer rna species. Heavy atom derivatization of aminoacyl tRNA. Biochem Biophys Res Commun. 1972 Jul 25;48(2):451–456. doi: 10.1016/s0006-291x(72)80072-x. [DOI] [PubMed] [Google Scholar]
- TABOR H. The protective effect of spermine and other polyamines against heat denaturation of deoxyribonucleic acid. Biochemistry. 1962 May 25;1:496–501. doi: 10.1021/bi00909a021. [DOI] [PubMed] [Google Scholar]
- Tang J. Y., Temsamani J., Agrawal S. Self-stabilized antisense oligodeoxynucleotide phosphorothioates: properties and anti-HIV activity. Nucleic Acids Res. 1993 Jun 11;21(11):2729–2735. doi: 10.1093/nar/21.11.2729. [DOI] [PMC free article] [PubMed] [Google Scholar]
- Tung C. H., Rudolph M. J., Stein S. Preparation of oligonucleotide-peptide conjugates. Bioconjug Chem. 1991 Nov-Dec;2(6):464–465. doi: 10.1021/bc00012a015. [DOI] [PubMed] [Google Scholar]