Abstract
The specificity of binding of Watson-Crick base pairs by third strand nucleic acid residues via triple helix formation was investigated in a DNA pyrimidine triplex motif by thermal melting experiments. The host duplex was of the type A10-X-A10: T10-Y-T10, and the third strand T10-Z-T10, giving rise to 16 possible triplexes with Z:XY inserts, 4 duplexes with the Watson-Crick base pairs (XY) and 12 duplexes with mismatch pairs (XZ), all of whose stabilities were compared. Two Z:XY combinations confirm the primary binding of AT and GC target pairs in homopurine.homopyrimidine sequences by T and C residues, respectively. All other Z:XY combinations in the T:AT environment result in triplex destabilization. While some related observations have been reported, the present experiments differ importantly in that they were performed in a T:AT nearest neighbor environment and at physiological ionic strength and pH, all of which were previously untested. The conclusions now drawn also differ substantially from those in previous studies. Thus, by evaluating the depression in Tm due to base triplet mismatches strictly in terms of third strand residue affinity and specificity for the target base pair, it is shown that none of the triplet combinations that destabilize qualify for inclusion in the third strand binding code for the pyrimidine triplex motif. Hence, none of the mismatch triplets afford a general way of circumventing the requirement for homopurine.homopyrimidine targets when third strands are predominated by pyrimidines, as others have suggested. At the same time, the applicability of third strand binding is emphasized by the finding that triplexes are equally or much more sensitive to base triplet mismatches than are Watson-Crick duplexes to base pair mismatches.
Full text
PDF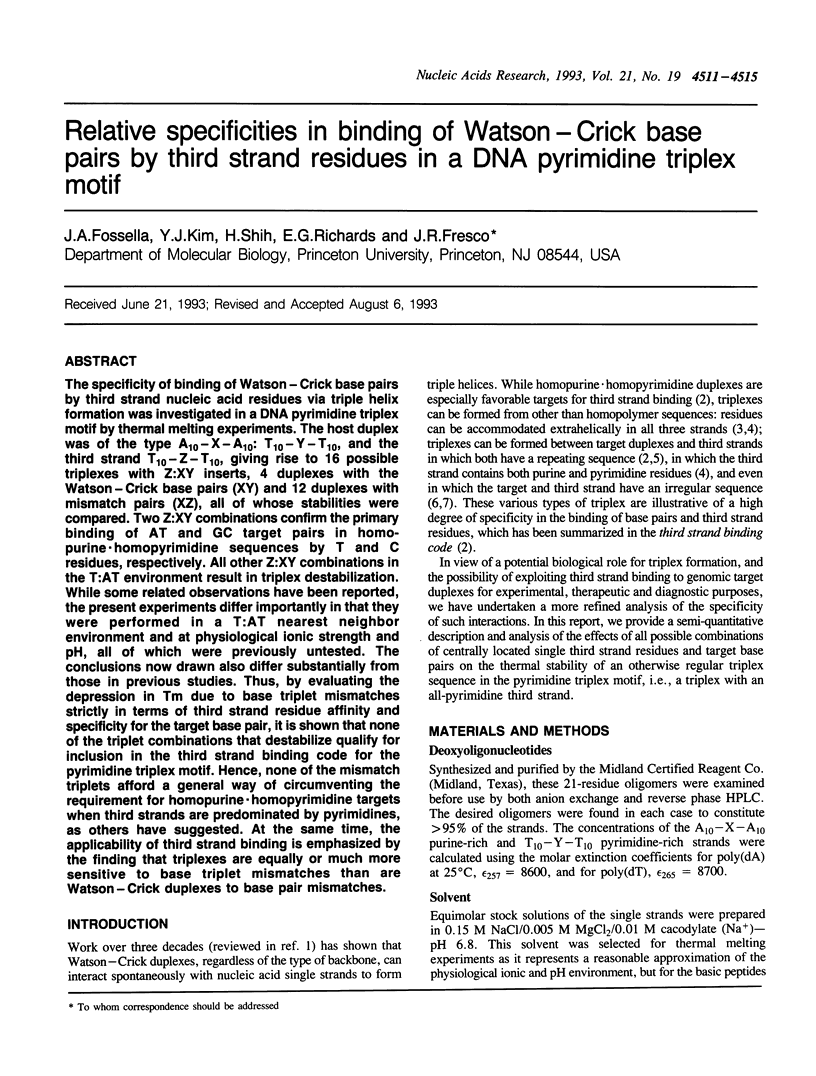
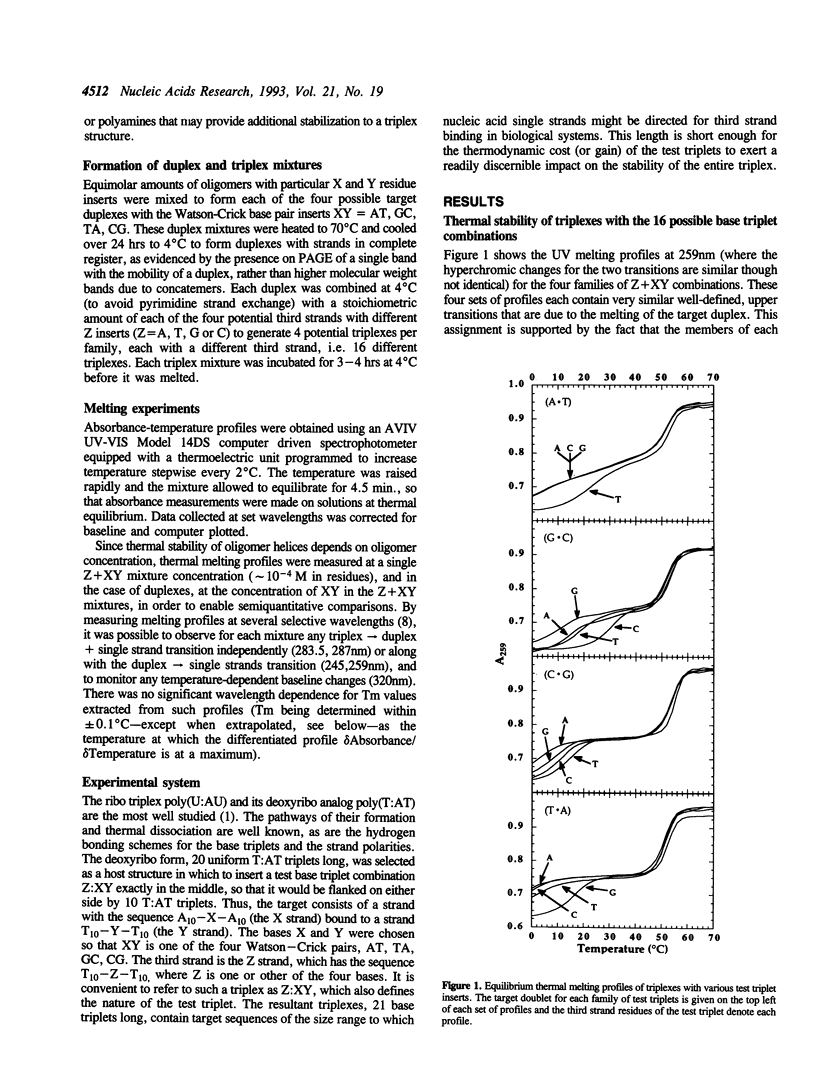
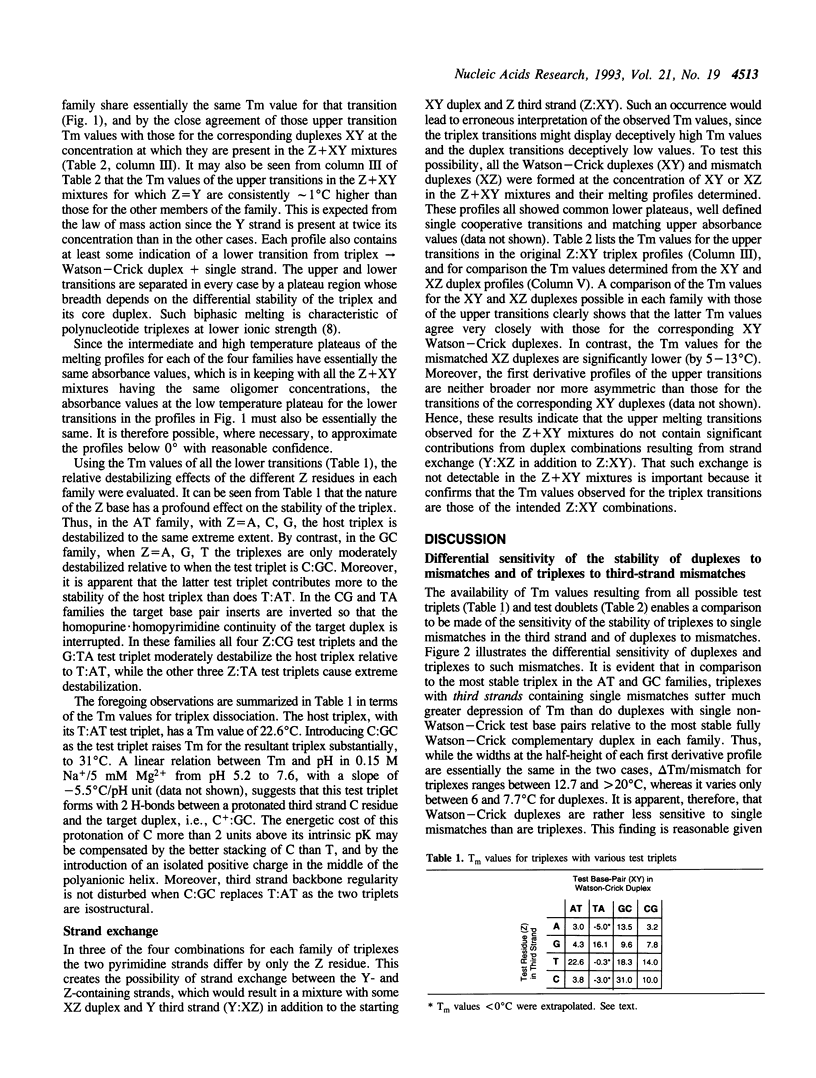
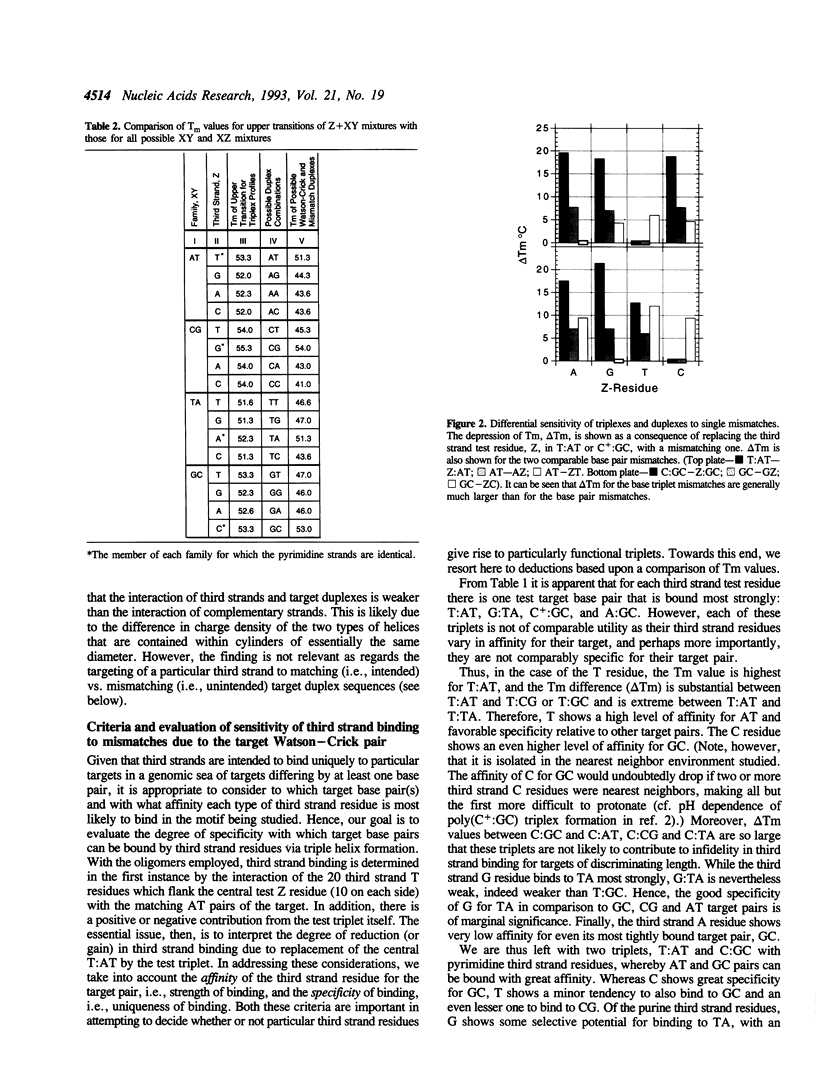
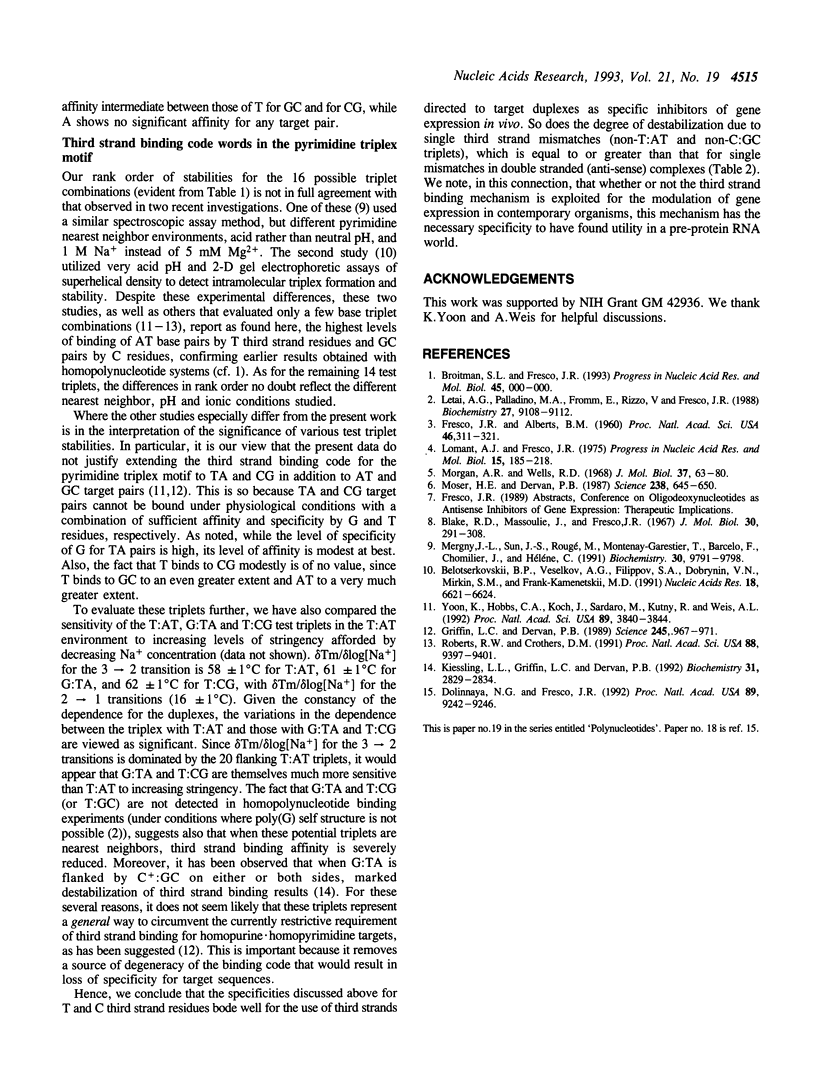
Selected References
These references are in PubMed. This may not be the complete list of references from this article.
- Belotserkovskii B. P., Veselkov A. G., Filippov S. A., Dobrynin V. N., Mirkin S. M., Frank-Kamenetskii M. D. Formation of intramolecular triplex in homopurine-homopyrimidine mirror repeats with point substitutions. Nucleic Acids Res. 1990 Nov 25;18(22):6621–6624. doi: 10.1093/nar/18.22.6621. [DOI] [PMC free article] [PubMed] [Google Scholar]
- Blake R. D., Massoulié J., Fresco J. R. Polynucleotides. 8. A spectral approach to the equilibria between polyriboadenylate and polyribouridylate and their complexes. J Mol Biol. 1967 Dec 14;30(2):291–308. [PubMed] [Google Scholar]
- Dolinnaya N. G., Fresco J. R. Single-stranded nucleic acid helical secondary structure stabilized by ionic bonds: d(A(+)-G)10. Proc Natl Acad Sci U S A. 1992 Oct 1;89(19):9242–9246. doi: 10.1073/pnas.89.19.9242. [DOI] [PMC free article] [PubMed] [Google Scholar]
- Fresco J. R., Alberts B. M. THE ACCOMMODATION OF NONCOMPLEMENTARY BASES IN HELICAL POLYRIBONUCLEOTIDES AND DEOXYRIBONUCLEIC ACIDS. Proc Natl Acad Sci U S A. 1960 Mar;46(3):311–321. doi: 10.1073/pnas.46.3.311. [DOI] [PMC free article] [PubMed] [Google Scholar]
- Griffin L. C., Dervan P. B. Recognition of thymine adenine.base pairs by guanine in a pyrimidine triple helix motif. Science. 1989 Sep 1;245(4921):967–971. doi: 10.1126/science.2549639. [DOI] [PubMed] [Google Scholar]
- Kiessling L. L., Griffin L. C., Dervan P. B. Flanking sequence effects within the pyrimidine triple-helix motif characterized by affinity cleaving. Biochemistry. 1992 Mar 17;31(10):2829–2834. doi: 10.1021/bi00125a026. [DOI] [PubMed] [Google Scholar]
- Letai A. G., Palladino M. A., Fromm E., Rizzo V., Fresco J. R. Specificity in formation of triple-stranded nucleic acid helical complexes: studies with agarose-linked polyribonucleotide affinity columns. Biochemistry. 1988 Dec 27;27(26):9108–9112. doi: 10.1021/bi00426a007. [DOI] [PubMed] [Google Scholar]
- Lomant A. J., Fresco J. R. Structural and energetic consequences of noncomplementary base oppositions in nucleic acid helices. Prog Nucleic Acid Res Mol Biol. 1975;15(0):185–218. doi: 10.1016/s0079-6603(08)60120-8. [DOI] [PubMed] [Google Scholar]
- Mergny J. L., Sun J. S., Rougée M., Montenay-Garestier T., Barcelo F., Chomilier J., Hélène C. Sequence specificity in triple-helix formation: experimental and theoretical studies of the effect of mismatches on triplex stability. Biochemistry. 1991 Oct 8;30(40):9791–9798. doi: 10.1021/bi00104a031. [DOI] [PubMed] [Google Scholar]
- Morgan A. R., Wells R. D. Specificity of the three-stranded complex formation between double-stranded DNA and single-stranded RNA containing repeating nucleotide sequences. J Mol Biol. 1968 Oct 14;37(1):63–80. doi: 10.1016/0022-2836(68)90073-9. [DOI] [PubMed] [Google Scholar]
- Moser H. E., Dervan P. B. Sequence-specific cleavage of double helical DNA by triple helix formation. Science. 1987 Oct 30;238(4827):645–650. doi: 10.1126/science.3118463. [DOI] [PubMed] [Google Scholar]
- Roberts R. W., Crothers D. M. Specificity and stringency in DNA triplex formation. Proc Natl Acad Sci U S A. 1991 Nov 1;88(21):9397–9401. doi: 10.1073/pnas.88.21.9397. [DOI] [PMC free article] [PubMed] [Google Scholar]
- Yoon K., Hobbs C. A., Koch J., Sardaro M., Kutny R., Weis A. L. Elucidation of the sequence-specific third-strand recognition of four Watson-Crick base pairs in a pyrimidine triple-helix motif: T.AT, C.GC, T.CG, and G.TA. Proc Natl Acad Sci U S A. 1992 May 1;89(9):3840–3844. doi: 10.1073/pnas.89.9.3840. [DOI] [PMC free article] [PubMed] [Google Scholar]