Abstract
The Na+/H+ antiporter of the obligate alkalophile Bacillus alcalophilus facilitates growth at alkaline pH and precludes growth below pH 8.5. Thus, nonalkalophilic mutant strains do not exhibit Na+/H+ antiport activity and, interestingly, such strains concomitantly lose the ability to catalyze Na+-dependent accumulation of alpha-aminoisobutyrate [Krulwich, T. A., Mandel, D. G. Bornstein, R. F. & Guffanti, A. A. (1979) Biochem. Biophys. Res. Commun. 91, 58-62]. Several other Na+-dependent transport systems are now documented in vesicles from the wild-type strain, and it is demonstrated that these systems are defective in vesicles from the nonalkalophilic mutant KM23. Surprisingly, the defect seems to result not from the loss of Na+/H+ antiport activity per se but from a pleiotropic defect in the Na+/substrate symporters themselves. Monensin, an ionophore that catalyzes Na+/H+ exchange, does not restore respiration-driven Na+/substrate symport in KM23 vesicles. Moreover, with KM23 vesicles, efflux of alpha-aminoisobutyrate, L-malate, and L-aspartate down their respective concentration gradients is not stimulated by Na+, in contrast to the observations with wild-type vesicles. Because monensin should ameliorate a simple defect in Na+/H+ antiport activity and the antiporter should not be required for Na+/substrate symport down a concentration gradient, the results suggest that there may be a direct relationship between the antiporter and various Na+/substrate symporters. One possibility is that the systems share a Na+-translocating subunit.
Full text
PDF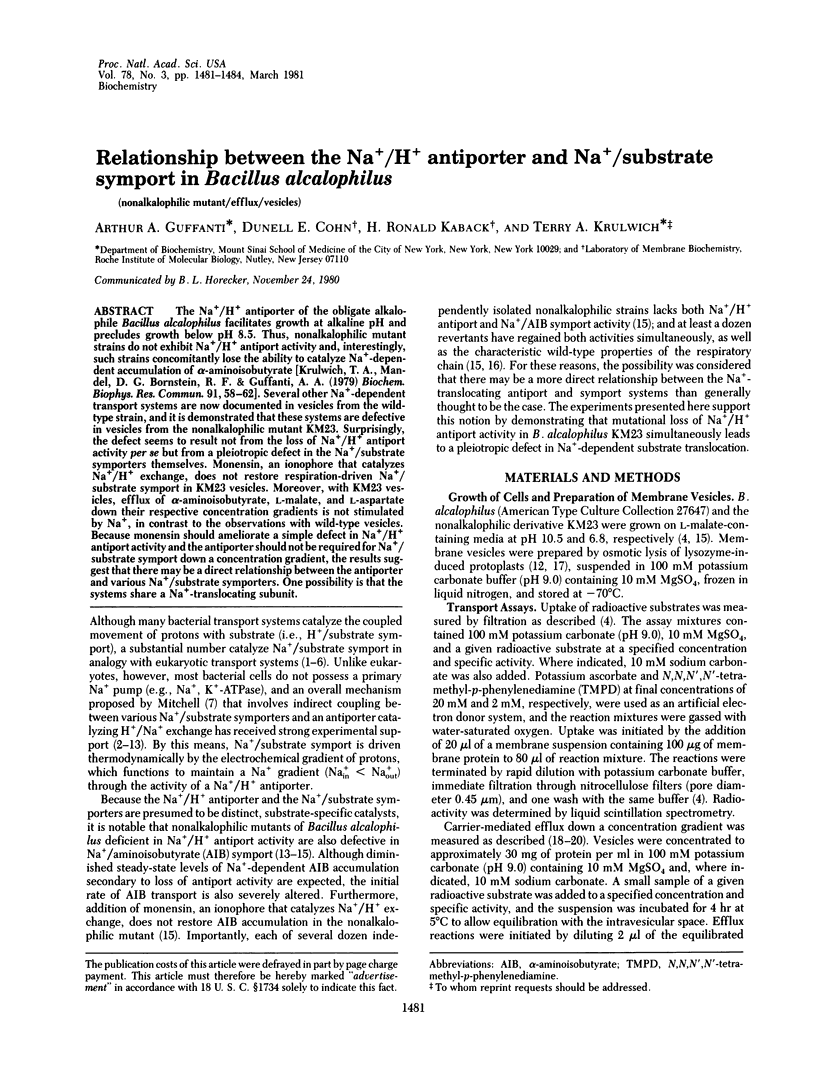
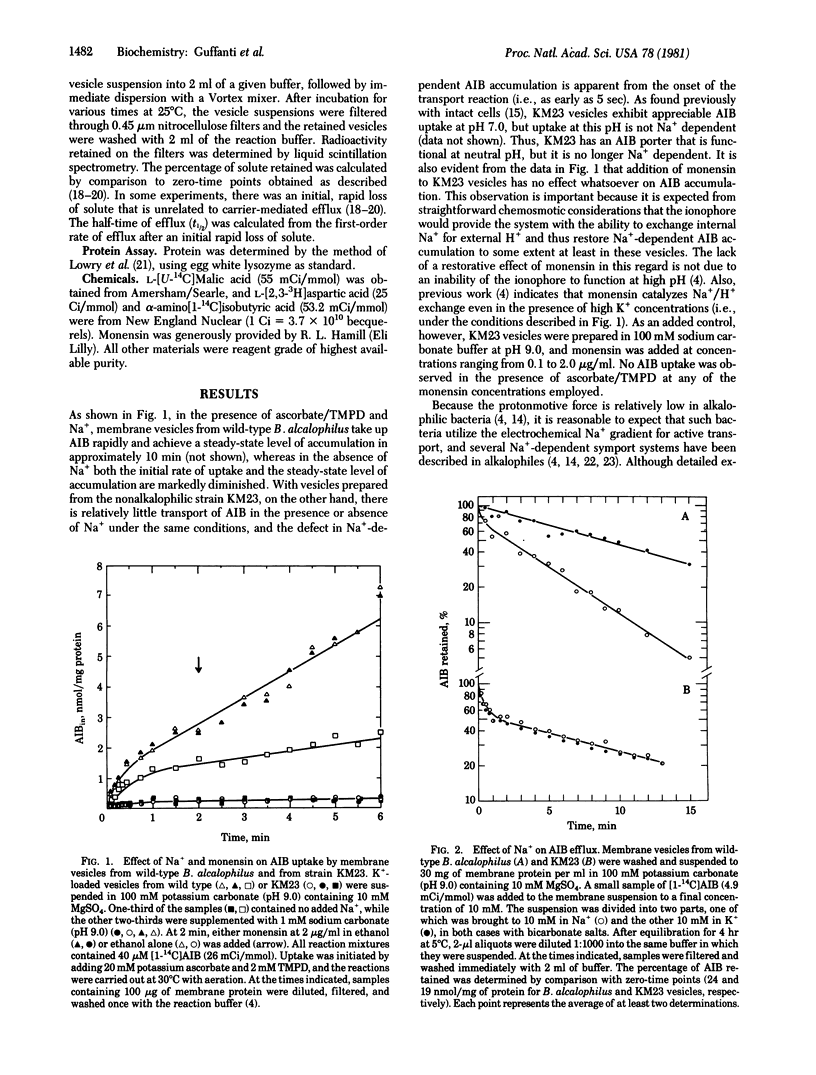
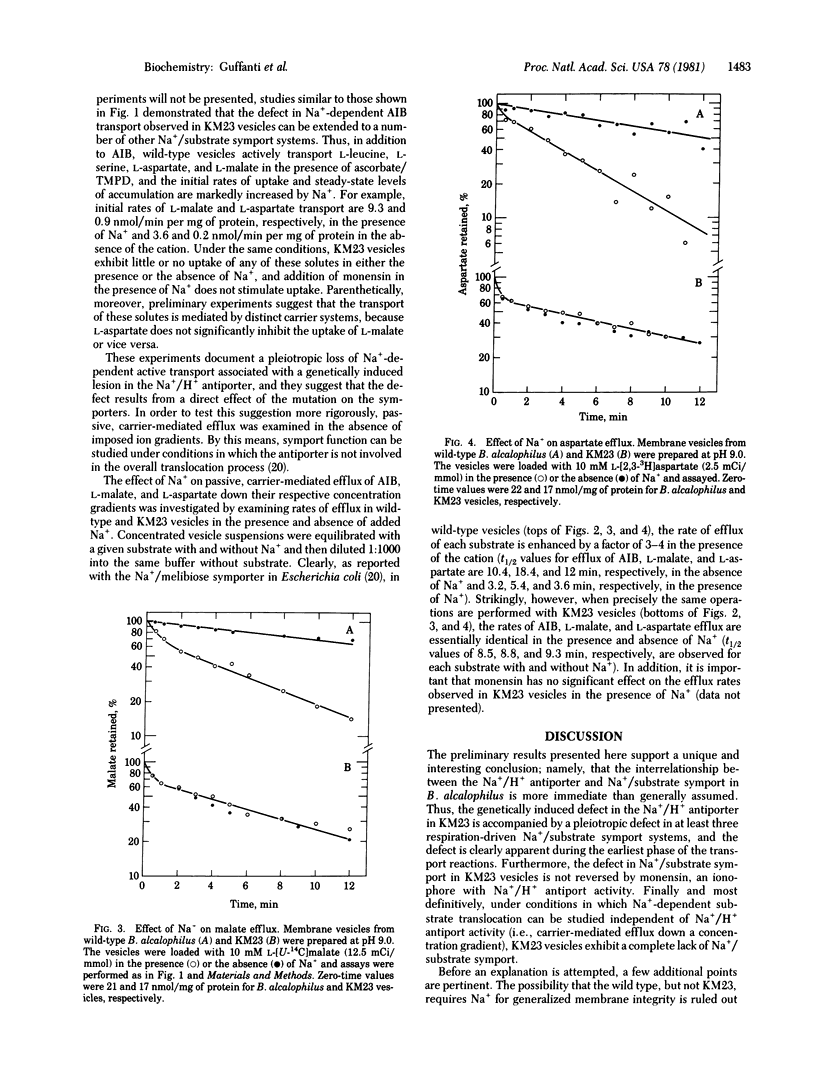
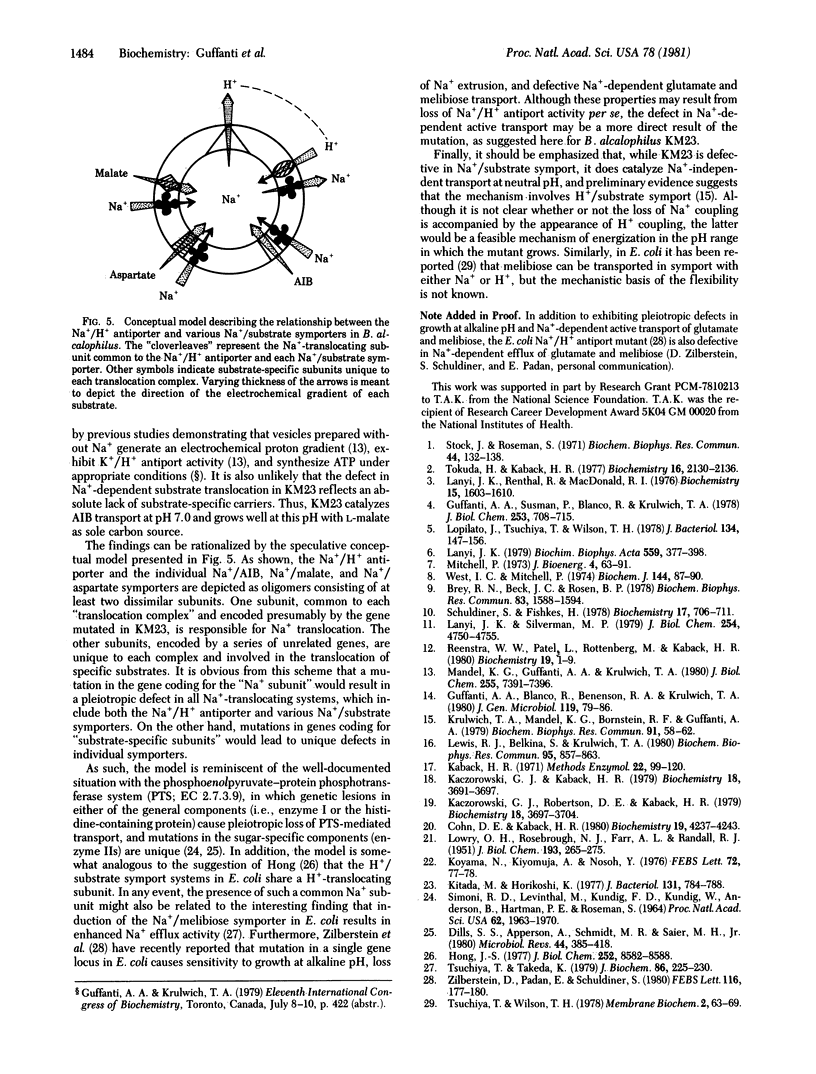
Selected References
These references are in PubMed. This may not be the complete list of references from this article.
- Brey R. N., Beck J. C., Rosen B. P. Cation/proton antiport systems in Escherichia coli. Biochem Biophys Res Commun. 1978 Aug 29;83(4):1588–1594. doi: 10.1016/0006-291x(78)91403-1. [DOI] [PubMed] [Google Scholar]
- Cohn D. E., Kaback H. R. Mechanism of the melibiose porter in membrane vesicles of Escherichia coli. Biochemistry. 1980 Sep 2;19(18):4237–4243. doi: 10.1021/bi00559a015. [DOI] [PubMed] [Google Scholar]
- Dills S. S., Apperson A., Schmidt M. R., Saier M. H., Jr Carbohydrate transport in bacteria. Microbiol Rev. 1980 Sep;44(3):385–418. doi: 10.1128/mr.44.3.385-418.1980. [DOI] [PMC free article] [PubMed] [Google Scholar]
- Guffanti A. A., Susman P., Blanco R., Krulwich T. A. The protonmotive force and alpha-aminoisobutyric acid transport in an obligately alkalophilic bacterium. J Biol Chem. 1978 Feb 10;253(3):708–715. [PubMed] [Google Scholar]
- Hong J. S. An ecf mutation in Escherichia coli pleiotropically affecting energy coupling in active transport but not generation or maintenance of membrane potential. J Biol Chem. 1977 Dec 10;252(23):8582–8588. [PubMed] [Google Scholar]
- Kaczorowski G. J., Kaback H. R. Mechanism of lactose translocation in membrane vesicles from Escherichia coli. 1. Effect of pH on efflux, exchange, and counterflow. Biochemistry. 1979 Aug 21;18(17):3691–3697. doi: 10.1021/bi00584a009. [DOI] [PubMed] [Google Scholar]
- Kaczorowski G. J., Robertson D. E., Kaback H. R. Mechanism of lactose translocation in membrane vesicles from Escherichia coli. 2. Effect of imposed delata psi, delta pH, and Delta mu H+. Biochemistry. 1979 Aug 21;18(17):3697–3704. doi: 10.1021/bi00584a010. [DOI] [PubMed] [Google Scholar]
- Kitada M., Horikoshi K. Sodium ion-stimulated alpha-[1-14C]aminoisobutyric acid uptake in alkalophilic Bacillus species. J Bacteriol. 1977 Sep;131(3):784–788. doi: 10.1128/jb.131.3.784-788.1977. [DOI] [PMC free article] [PubMed] [Google Scholar]
- Koyama N., Kiyomiya A., Nosoh Y. Na+-dependent uptake of amino acids by an alkalophilic Bacillus. FEBS Lett. 1976 Dec 15;72(1):77–78. doi: 10.1016/0014-5793(76)80816-2. [DOI] [PubMed] [Google Scholar]
- Krulwich T. A., Mandel K. G., Bornstein R. F., Guffanti A. A. A non-alkalophilic mutant of Bacillus alcalophilus lacks the Na+/H+ antiporter. Biochem Biophys Res Commun. 1979 Nov 14;91(1):58–62. doi: 10.1016/0006-291x(79)90582-5. [DOI] [PubMed] [Google Scholar]
- LOWRY O. H., ROSEBROUGH N. J., FARR A. L., RANDALL R. J. Protein measurement with the Folin phenol reagent. J Biol Chem. 1951 Nov;193(1):265–275. [PubMed] [Google Scholar]
- Lanyi J. K., Renthal R., MacDonald R. E. Light-induced glutamate transport in Halobacterium halobium envelope vesicles. II. Evidence that the driving force is a light-dependent sodium gradient. Biochemistry. 1976 Apr 20;15(8):1603–1610. doi: 10.1021/bi00653a002. [DOI] [PubMed] [Google Scholar]
- Lanyi J. K., Silverman M. P. Gating effects in Halobacterium halobium membrane transport. J Biol Chem. 1979 Jun 10;254(11):4750–4755. [PubMed] [Google Scholar]
- Lanyi J. K. The role of Na+ in transport processes of bacterial membranes. Biochim Biophys Acta. 1979 Dec 20;559(4):377–397. doi: 10.1016/0304-4157(79)90011-x. [DOI] [PubMed] [Google Scholar]
- Lewis R. J., Belkina S., Krulwich T. A. Alkalophiles have much higher cytochrome contents than conventional bacteria and than their own non-alkalophilic mutant derivatives. Biochem Biophys Res Commun. 1980 Jul 31;95(2):857–863. doi: 10.1016/0006-291x(80)90866-9. [DOI] [PubMed] [Google Scholar]
- Lopilato J., Tsuchiya T., Wilson T. H. Role of Na+ and Li+ in thiomethylgalactoside transport by the melibiose transport system of Escherichia coli. J Bacteriol. 1978 Apr;134(1):147–156. doi: 10.1128/jb.134.1.147-156.1978. [DOI] [PMC free article] [PubMed] [Google Scholar]
- Mandel K. G., Guffanti A. A., Krulwich T. A. Monovalent cation/proton antiporters in membrane vesicles from Bacillus alcalophilus. J Biol Chem. 1980 Aug 10;255(15):7391–7396. [PubMed] [Google Scholar]
- Mitchell P. Performance and conservation of osmotic work by proton-coupled solute porter systems. J Bioenerg. 1973 Jan;4(1):63–91. doi: 10.1007/BF01516051. [DOI] [PubMed] [Google Scholar]
- Schuldiner S., Fishkes H. Sodium-proton antiport in isolated membrane vesicles of Escherichia coli. Biochemistry. 1978 Feb 21;17(4):706–711. doi: 10.1021/bi00597a023. [DOI] [PubMed] [Google Scholar]
- Stock J., Roseman S. A sodium-dependent sugar co-transport system in bacteria. Biochem Biophys Res Commun. 1971 Jul 2;44(1):132–138. doi: 10.1016/s0006-291x(71)80168-7. [DOI] [PubMed] [Google Scholar]
- Tokuda H., Kaback H. R. Sodium-dependent methyl 1-thio-beta-D-galactopyranoside transport in membrane vesicles isolated from Salmonella typhimurium. Biochemistry. 1977 May 17;16(10):2130–2136. doi: 10.1021/bi00629a013. [DOI] [PubMed] [Google Scholar]
- Tsuchiya T., Takeda K. Extrusion of sodium ions energized by respiration and glycolysis in Escherichia coli. J Biochem. 1979 Jul;86(1):225–230. [PubMed] [Google Scholar]
- West I. C., Mitchell P. Proton/sodium ion antiport in Escherichia coli. Biochem J. 1974 Oct;144(1):87–90. doi: 10.1042/bj1440087. [DOI] [PMC free article] [PubMed] [Google Scholar]
- Zilberstein D., Padan E., Schuldiner S. A single locus in Escherichia coli governs growth in alkaline pH and on carbon sources whose transport is sodium dependent. FEBS Lett. 1980 Jul 28;116(2):177–180. doi: 10.1016/0014-5793(80)80637-5. [DOI] [PubMed] [Google Scholar]