Abstract
The chemosmotic model provides a framework for visualizing energy-coupled reactions (vectorial reaction sequences, membrane-dependent gradient formation, and charge separation of reacting species) and a mechanism for energy coupling (indirect coupling between the driving and driven reaction sequences mediated by a membrane potential or a protonmotive force). The mechanistic parameters of this model have been examined from four standpoints: compatibility with the experimental realities, supporting evidence that is unambiguous, compatibility with the enzymic nature of energy coupling, and the capability for generating verifiable predictions. Recent developments that have clarified the mechanism of ion transport, the nature of the protonic changes that accompany energy coupling, and the enzymic nature of energy coupling systems have made such an examination both timely and necessary. After weighing the available evidence, it has been concluded that the chemosmotic principle of indirect coupling has no basis in fact and that it is physically unsound in respect to the mechanism of energy coupling and enzymic catalysis.
Full text
PDF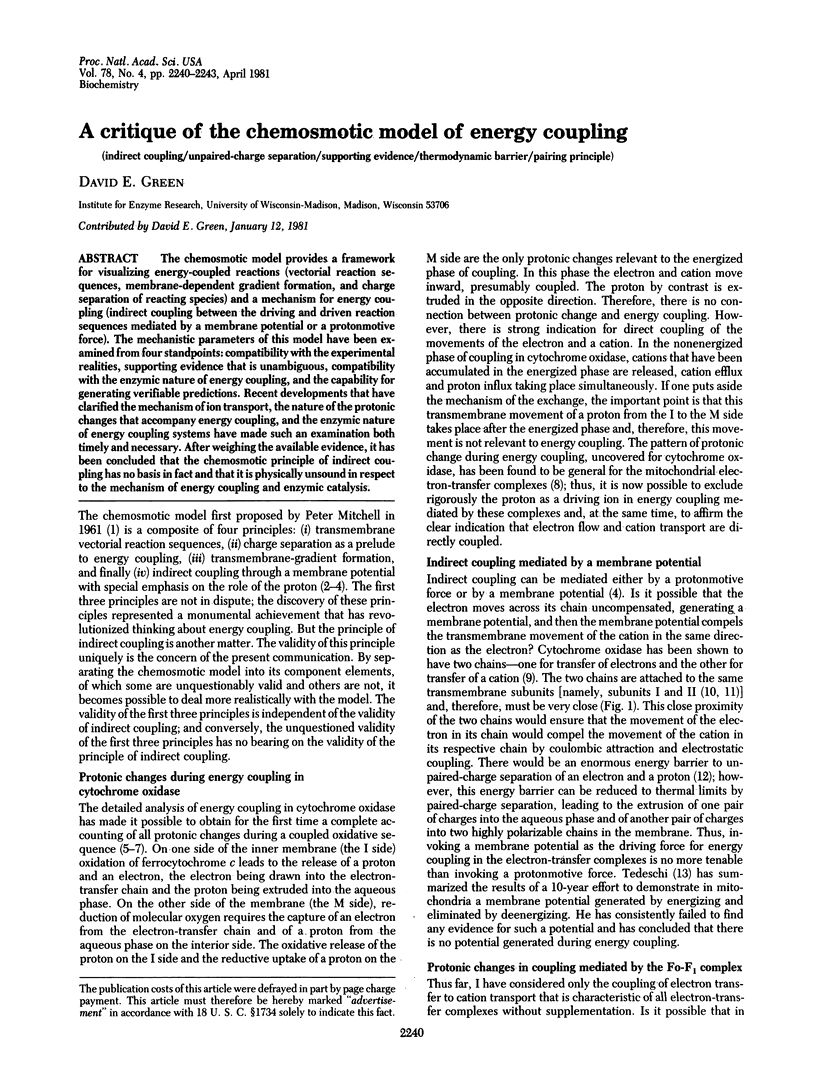
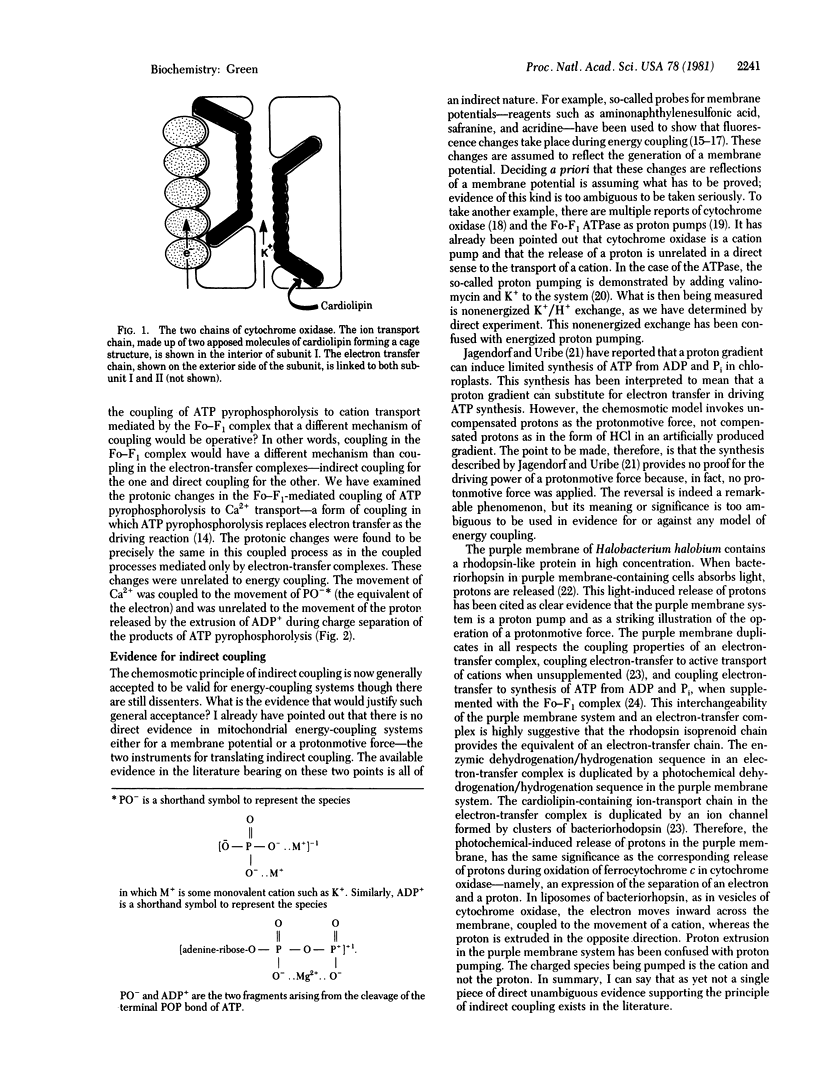
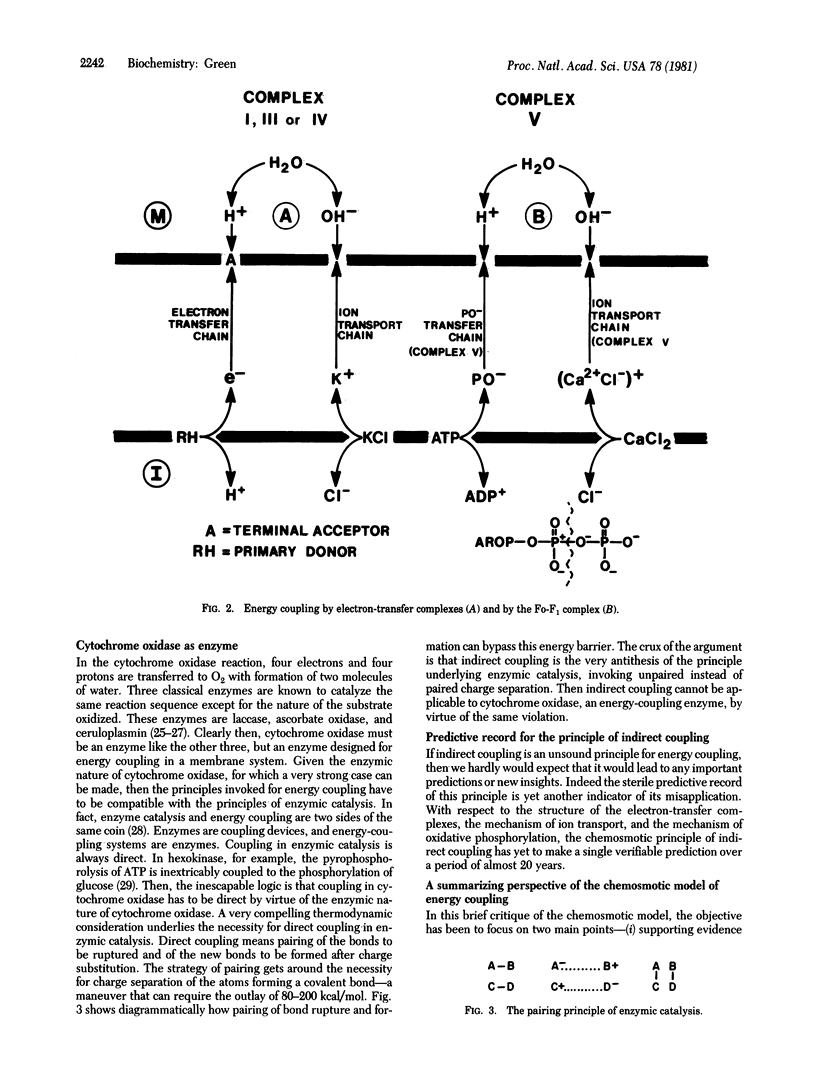
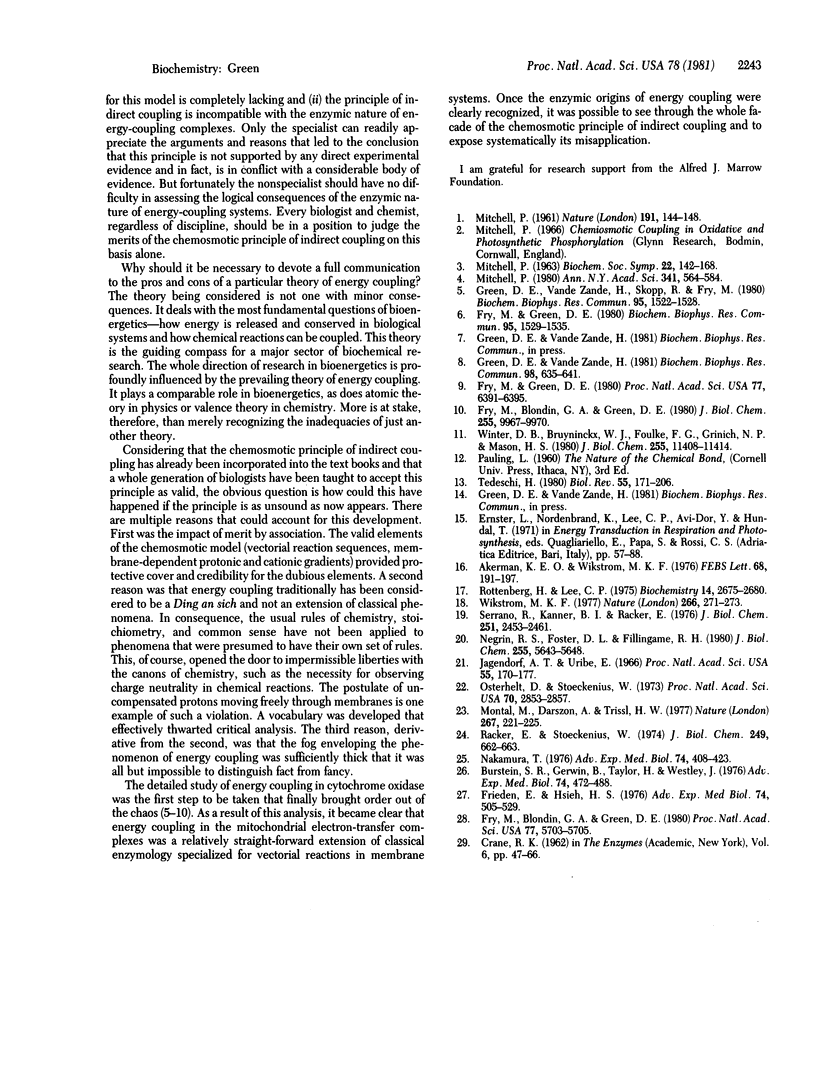
Selected References
These references are in PubMed. This may not be the complete list of references from this article.
- Akerman K. E., Wikström M. K. Safranine as a probe of the mitochondrial membrane potential. FEBS Lett. 1976 Oct 1;68(2):191–197. doi: 10.1016/0014-5793(76)80434-6. [DOI] [PubMed] [Google Scholar]
- Burstein S. R., Gerwin B., Taylor H., Westley J. Forman catalytic mechanism of ascorbate oxidase. Adv Exp Med Biol. 1976;74:472–488. doi: 10.1007/978-1-4684-3270-1_41. [DOI] [PubMed] [Google Scholar]
- Frieden E., Hsieh H. S. The biological role of ceruloplasmin and its oxidase activity. Adv Exp Med Biol. 1976;74:505–529. doi: 10.1007/978-1-4684-3270-1_43. [DOI] [PubMed] [Google Scholar]
- Fry M., Blondin G. A., Green D. E. Relation between enzymic catalysis and energy coupling. Proc Natl Acad Sci U S A. 1980 Oct;77(10):5703–5705. doi: 10.1073/pnas.77.10.5703. [DOI] [PMC free article] [PubMed] [Google Scholar]
- Fry M., Blondin G. A., Green D. E. The localization of tightly bound cardiolipin in cytochrome oxidase. J Biol Chem. 1980 Oct 25;255(20):9967–9970. [PubMed] [Google Scholar]
- Fry M., Green D. E. Energized transport of cations by cytochrome oxidase. Biochem Biophys Res Commun. 1980 Aug 29;95(4):1529–1535. doi: 10.1016/s0006-291x(80)80071-4. [DOI] [PubMed] [Google Scholar]
- Fry M., Green D. E. Ion-transport chain of cytochrome oxidase: the two chain-direct coupling principle of energy coupling. Proc Natl Acad Sci U S A. 1980 Nov;77(11):6391–6395. doi: 10.1073/pnas.77.11.6391. [DOI] [PMC free article] [PubMed] [Google Scholar]
- Green D. E., Vande Zande H. Bound cytochrome C as proton donor and acceptor during enzymic oxidoreduction. Biochem Biophys Res Commun. 1981 Feb 12;98(3):635–641. doi: 10.1016/0006-291x(81)91161-x. [DOI] [PubMed] [Google Scholar]
- Jagendorf A. T., Uribe E. ATP formation caused by acid-base transition of spinach chloroplasts. Proc Natl Acad Sci U S A. 1966 Jan;55(1):170–177. doi: 10.1073/pnas.55.1.170. [DOI] [PMC free article] [PubMed] [Google Scholar]
- MITCHELL P. Coupling of phosphorylation to electron and hydrogen transfer by a chemi-osmotic type of mechanism. Nature. 1961 Jul 8;191:144–148. doi: 10.1038/191144a0. [DOI] [PubMed] [Google Scholar]
- Mitchell P. Protonmotive cytochrome system of mitochondria. Ann N Y Acad Sci. 1980;341:564–584. doi: 10.1111/j.1749-6632.1980.tb47199.x. [DOI] [PubMed] [Google Scholar]
- Montal M., Darszon A., Trissl H. W. Transmembrane channel formation in rhodopsin-containing bilayer membranes. Nature. 1977 May 19;267(5608):221–225. doi: 10.1038/267221a0. [DOI] [PubMed] [Google Scholar]
- Nakamura T. Oxidation and reduction of copper ions in catalytic reactions of Rhus laccase. Adv Exp Med Biol. 1976;74:408–423. doi: 10.1007/978-1-4684-3270-1_35. [DOI] [PubMed] [Google Scholar]
- Oesterhelt D., Stoeckenius W. Functions of a new photoreceptor membrane. Proc Natl Acad Sci U S A. 1973 Oct;70(10):2853–2857. doi: 10.1073/pnas.70.10.2853. [DOI] [PMC free article] [PubMed] [Google Scholar]
- Racker E., Stoeckenius W. Reconstitution of purple membrane vesicles catalyzing light-driven proton uptake and adenosine triphosphate formation. J Biol Chem. 1974 Jan 25;249(2):662–663. [PubMed] [Google Scholar]
- Rottenberg H., Lee C. P. Energy dependent hydrogen ion accumulation in submitochondrial particles. Biochemistry. 1975 Jun 17;14(12):2675–2680. doi: 10.1021/bi00683a017. [DOI] [PubMed] [Google Scholar]
- Serrano R., Kanner B. I., Racker E. Purification and properties of the proton-translocating adenosine triphosphatase complex of bovine heart mitochondria. J Biol Chem. 1976 Apr 25;251(8):2453–2461. [PubMed] [Google Scholar]
- Tedeschi H. The mitochondrial membrane potential. Biol Rev Camb Philos Soc. 1980 May;55(2):171–206. doi: 10.1111/j.1469-185x.1980.tb00692.x. [DOI] [PubMed] [Google Scholar]
- Vande Zande H. U., Skopp R., Fry M. Intrinsic coupling in cytochrome oxidase: nature and stoichiometry of the coupling reactions. Biochem Biophys Res Commun. 1980 Aug 29;95(4):1522–1528. doi: 10.1016/s0006-291x(80)80070-2. [DOI] [PubMed] [Google Scholar]
- Wikstrom M. K. Proton pump coupled to cytochrome c oxidase in mitochondria. Nature. 1977 Mar 17;266(5599):271–273. doi: 10.1038/266271a0. [DOI] [PubMed] [Google Scholar]
- Winter D. B., Bruyninckx W. J., Foulke F. G., Grinich N. P., Mason H. S. Location of heme a on subunits I and II and copper on subunit II of cytochrome c oxidase. J Biol Chem. 1980 Dec 10;255(23):11408–11414. [PubMed] [Google Scholar]