Abstract
The currently prevalent concept of the generation of photosynthetic reducing power in oxygen-evolving cells envisions a linear (noncyclic) electron flow from water to ferredoxin (and thence to NADP+) that requires the collaboration of photosystems I and II (PSI and PSII) joined by plastoquinone and other electron carriers (the Z scheme). The essence of the Z scheme is that only PSI can reduce ferredoxin—i.e., that, after being energized to an intermediate reducing potential by PSII, electrons from water are transported via plastoquinone to PSI which energizes the electrons to their ultimate reducing potential adequate for the reduction of ferredoxin. Basic to the Z scheme is the function of plastoquinone as the obligatory link in electron transport from PSII to PSI. However, we have found that, when plastoquinone function was inhibited, ferredoxin was photoreduced by water without the collaboration of PSI. We now report evidence for an important function of plastoquinone in the translocation of protons liberated inside the thylakoid membrane by photooxidation of water. When the oxygenic photoreduction (i.e., by water) of ferredoxin was blocked by plastoquinone inhibitors, dibromothymoquinone or dinitrophenol ether of iodonitrothymol, the photoreduction of ferredoxin was restored by each of four chemically diverse uncouplers, similar only in their ability to facilitate proton movement across membranes. Similar results were obtained for the oxygenic reduction of NADP+. Our results suggest that the light-induced electron flow from water cannot be maintained unless the simultaneously liberated protons are removed from inside the membrane via plastoquinone. The new evidence is embodied in a concept of an oxygenic photosystem for photosynthetic electron and proton transport, which we propose as an alternative to the Z scheme, to account for photoreduction of ferredoxin-NADP+ by water and the coupled oxygenic (formerly noncyclic) ATP formation without involving PSI. The role of the anoxygenic photosystem (formerly called PSI) is ATP formation by cyclic photophosphorylation.
Keywords: uncouplers, plastoquinone, photosystems I and II, oxygenic and anoxygenic photosystems
Full text
PDF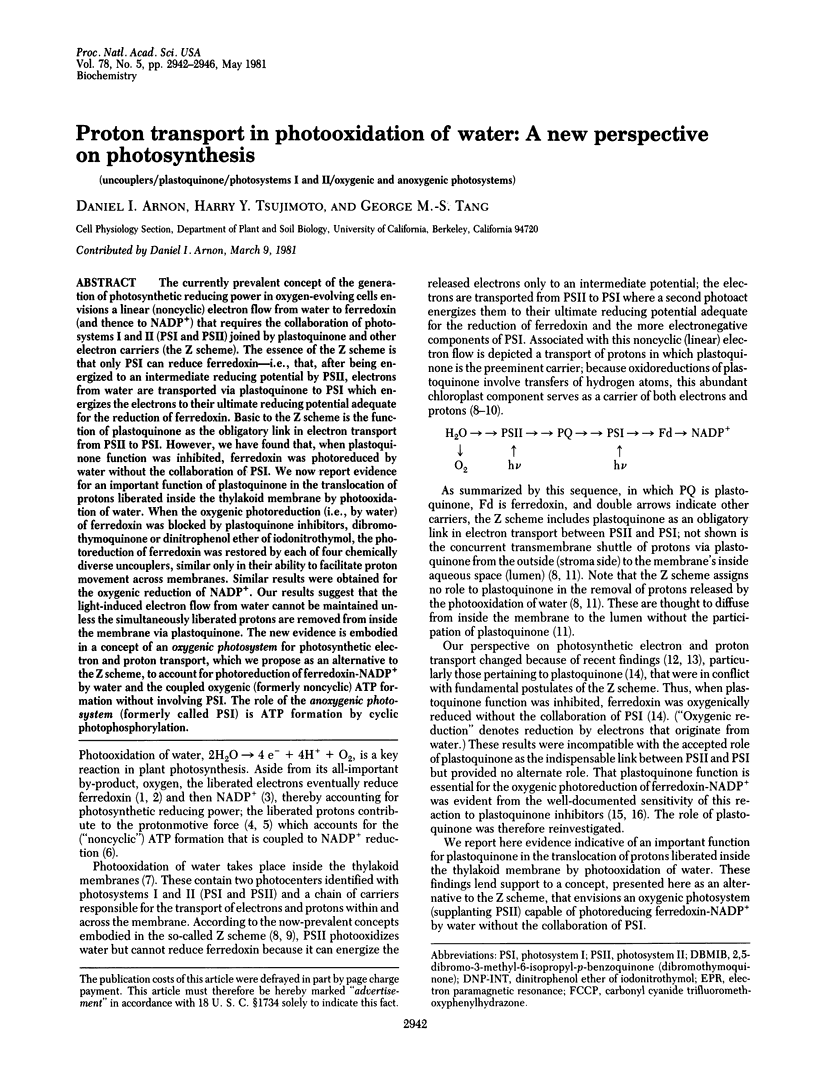
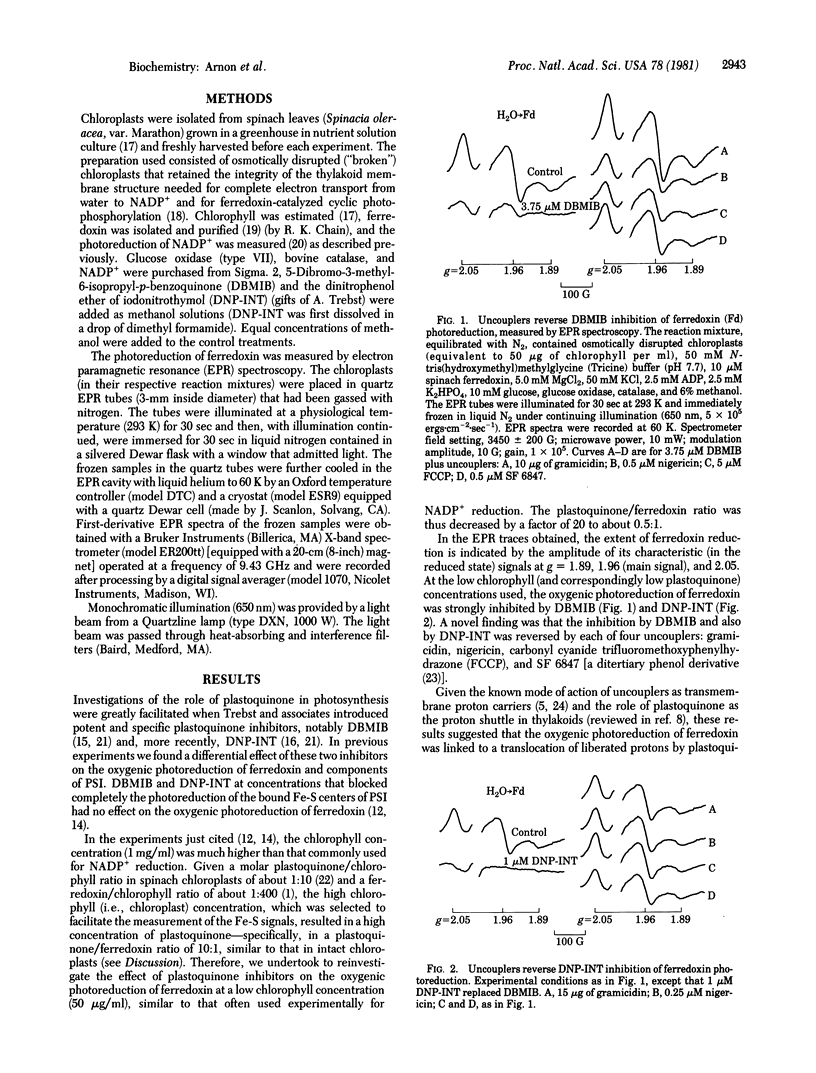
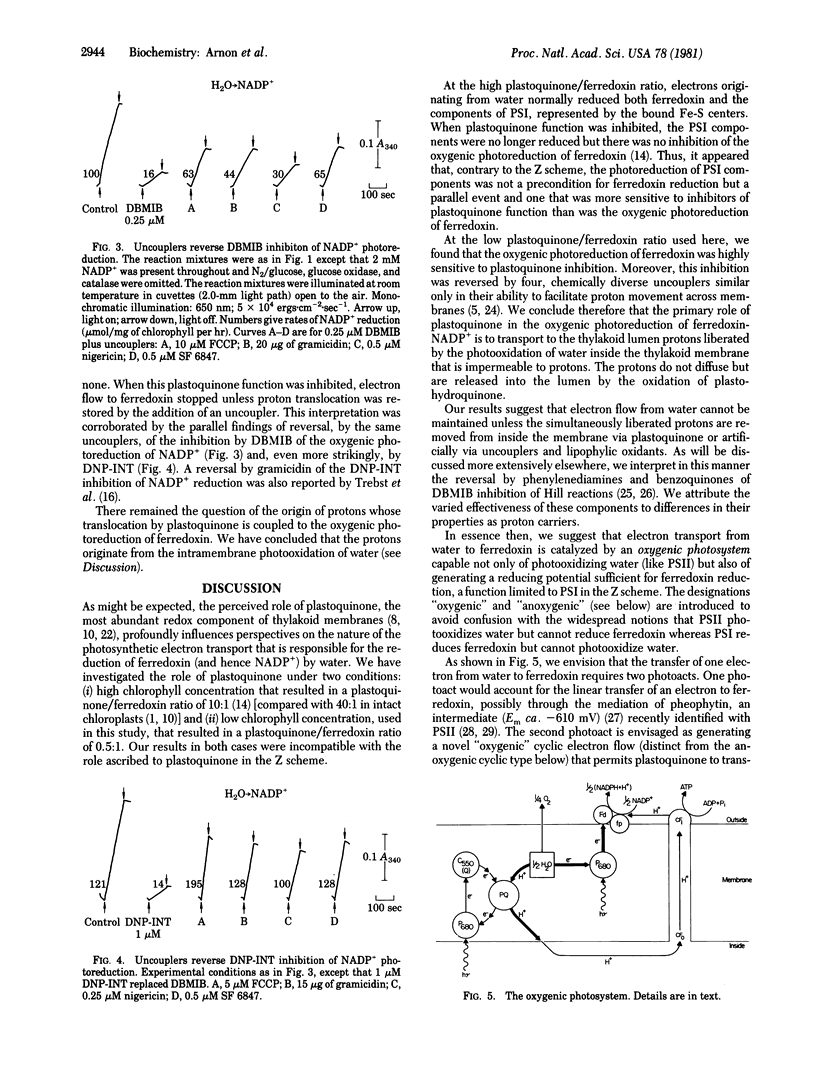
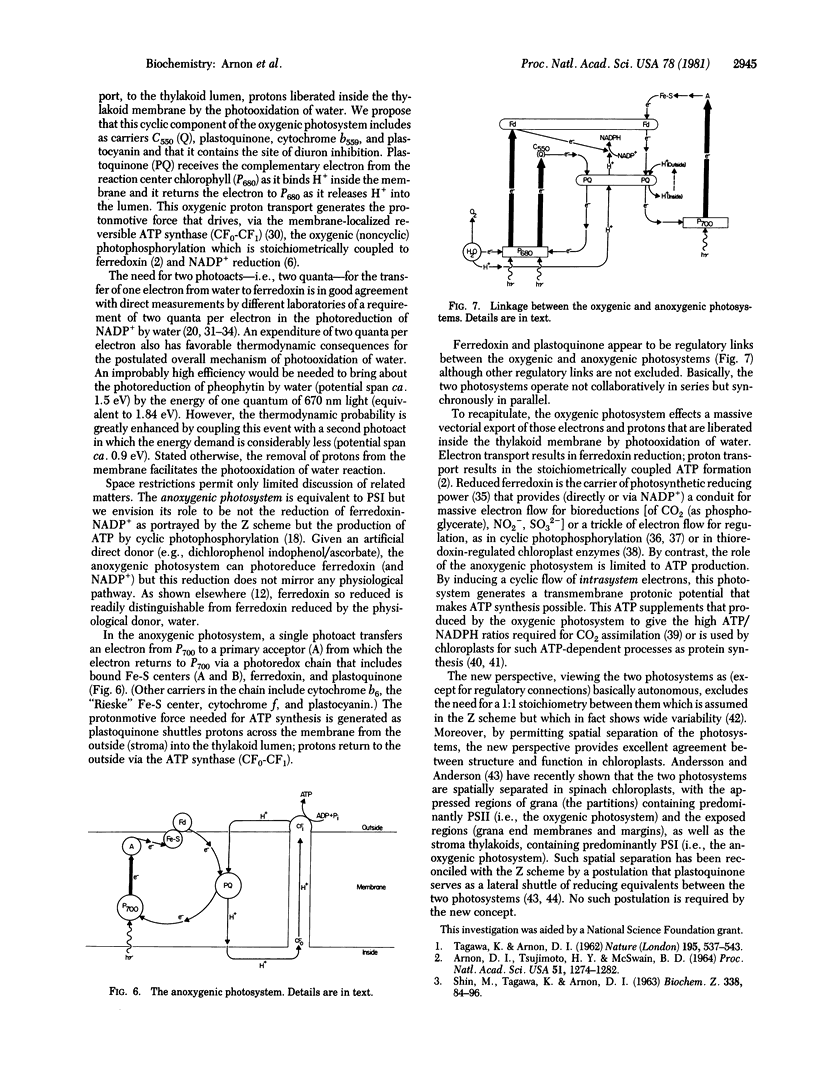
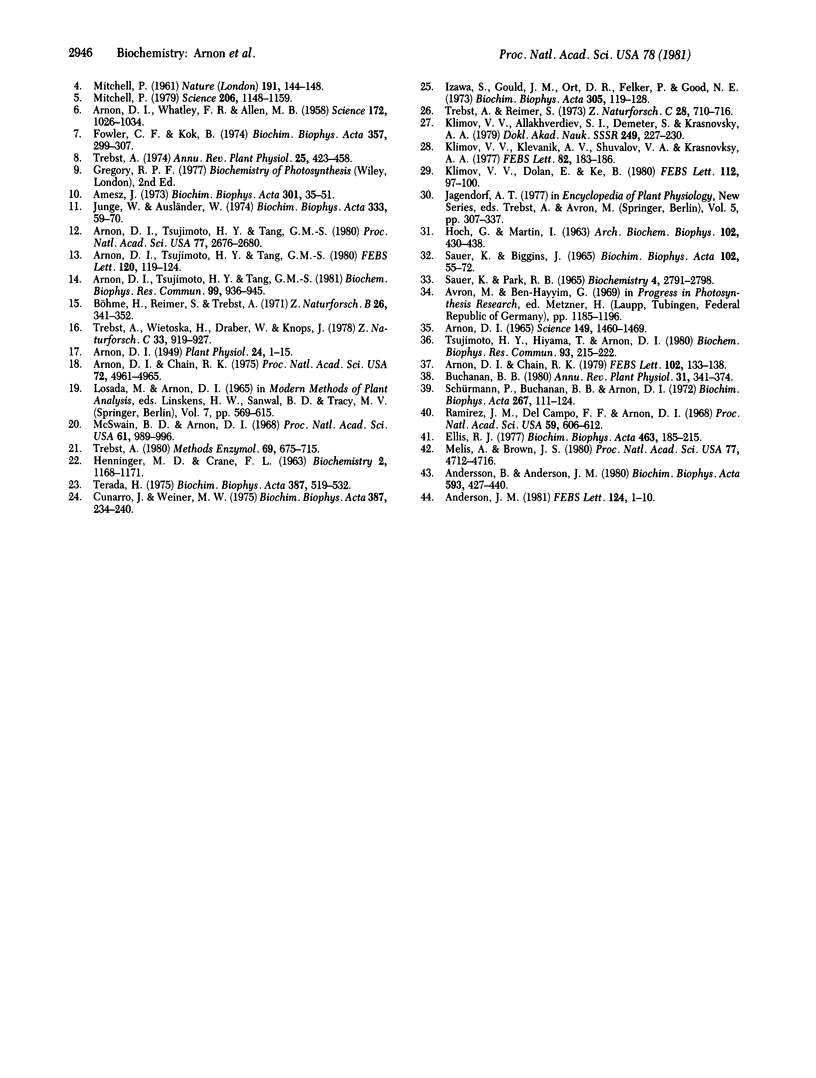
Selected References
These references are in PubMed. This may not be the complete list of references from this article.
- ARNON D. I., TSUJIMOTO H. Y., MCSWAIN B. D. ROLE OF FERREDOXIN IN PHOTOSYNTHETIC PRODUCTION OF OXYGEN AND PHOSPHORYLATION BY CHLOROPLASTS. Proc Natl Acad Sci U S A. 1964 Jun;51:1274–1282. doi: 10.1073/pnas.51.6.1274. [DOI] [PMC free article] [PubMed] [Google Scholar]
- Amesz J. The function of plastoquinone in photosynthetic electron transport. Biochim Biophys Acta. 1973 Feb 12;301(1):35–51. doi: 10.1016/0304-4173(73)90011-6. [DOI] [PubMed] [Google Scholar]
- Andersson B., Anderson J. M. Lateral heterogeneity in the distribution of chlorophyll-protein complexes of the thylakoid membranes of spinach chloroplasts. Biochim Biophys Acta. 1980 Dec 3;593(2):427–440. doi: 10.1016/0005-2728(80)90078-x. [DOI] [PubMed] [Google Scholar]
- Arnon D. I. COPPER ENZYMES IN ISOLATED CHLOROPLASTS. POLYPHENOLOXIDASE IN BETA VULGARIS. Plant Physiol. 1949 Jan;24(1):1–15. doi: 10.1104/pp.24.1.1. [DOI] [PMC free article] [PubMed] [Google Scholar]
- Arnon D. I., Chain R. K. Regulation of ferredoxin-catalyzed photosynthetic phosphorylations. Proc Natl Acad Sci U S A. 1975 Dec;72(12):4961–4965. doi: 10.1073/pnas.72.12.4961. [DOI] [PMC free article] [PubMed] [Google Scholar]
- Arnon D. I., Chain R. K. Regulatory electron transport pathways in cyclic photophosphorylation: reduction in C-550 and cytochrome b6 by ferrodoxin in the dark. FEBS Lett. 1979 Jun 1;102(1):133–138. doi: 10.1016/0014-5793(79)80944-8. [DOI] [PubMed] [Google Scholar]
- Arnon D. I. Ferredoxin and photosynthesis. Science. 1965 Sep 24;149(3691):1460–1470. doi: 10.1126/science.149.3691.1460. [DOI] [PubMed] [Google Scholar]
- Arnon D. I., Tsujimoto H. Y., Tang G. M. Contrasts between oxygenic and anoxygenic photoreduction of ferredoxin: Incompatibilities with prevailing concepts of photosynthetic electron transport. Proc Natl Acad Sci U S A. 1980 May;77(5):2676–2680. doi: 10.1073/pnas.77.5.2676. [DOI] [PMC free article] [PubMed] [Google Scholar]
- Arnon D. I., Tsujimoto H. Y., Tang G. M. Oxygenic photoreduction of ferredoxin independently of the membrane-bound iron-sulfur centers of photosystem I. Biochem Biophys Res Commun. 1981 Apr 15;99(3):936–945. doi: 10.1016/0006-291x(81)91253-5. [DOI] [PubMed] [Google Scholar]
- Arnon D. I., Whatley F. R., Allen M. B. Assimilatory Power in Photosynthesis: Photosynthetic phosphorylation by isolated chloroplasts is coupled with TPN reduction. Science. 1958 May 2;127(3305):1026–1034. doi: 10.1126/science.127.3305.1026. [DOI] [PubMed] [Google Scholar]
- Cunarro J., Weiner M. W. Mechanism of action of agents which uncouple oxidative phosphorylation: direct correlation between proton-carrying and respiratory-releasing properties using rat liver mitochondria. Biochim Biophys Acta. 1975 May 15;387(2):234–240. doi: 10.1016/0005-2728(75)90106-1. [DOI] [PubMed] [Google Scholar]
- Fowler C. F., Kok B. Proton evolution associated with the photooxidation of water in photosynthesis. Biochim Biophys Acta. 1974 Aug 23;357(2):299–307. doi: 10.1016/0005-2728(74)90068-1. [DOI] [PubMed] [Google Scholar]
- HOCH G., MARTIN I. TWO LIGHT REACTIONS IN TPN REDUCTION BY SPINACH CHLOROPLASTS. Arch Biochem Biophys. 1963 Sep;102:430–438. doi: 10.1016/0003-9861(63)90251-0. [DOI] [PubMed] [Google Scholar]
- Izawa S., Gould J. M., Ort D. R., Felker P., Good N. E. Electron transport and photophosphorylation in chloroplasts as a function of the electron acceptor. 3. A dibromothymoquinone-insensitive phosphorylation reaction associated with photosystem II. Biochim Biophys Acta. 1973 Apr 27;305(1):119–128. doi: 10.1016/0005-2728(73)90237-5. [DOI] [PubMed] [Google Scholar]
- Klimov V. V., Klevanik A. V., Shuvalov V. A., Kransnovsky A. A. Reduction of pheophytin in the primary light reaction of photosystem II. FEBS Lett. 1977 Oct 15;82(2):183–186. doi: 10.1016/0014-5793(77)80580-2. [DOI] [PubMed] [Google Scholar]
- MITCHELL P. Coupling of phosphorylation to electron and hydrogen transfer by a chemi-osmotic type of mechanism. Nature. 1961 Jul 8;191:144–148. doi: 10.1038/191144a0. [DOI] [PubMed] [Google Scholar]
- McSwain B. D., Arnon D. I. Enhancement effects and the identity of the two photochemical reactions of photosynthesis. Proc Natl Acad Sci U S A. 1968 Nov;61(3):989–996. doi: 10.1073/pnas.61.3.989. [DOI] [PMC free article] [PubMed] [Google Scholar]
- Melis A., Brown J. S. Stoichiometry of system I and system II reaction centers and of plastoquinone in different photosynthetic membranes. Proc Natl Acad Sci U S A. 1980 Aug;77(8):4712–4716. doi: 10.1073/pnas.77.8.4712. [DOI] [PMC free article] [PubMed] [Google Scholar]
- Mitchell P. Keilin's respiratory chain concept and its chemiosmotic consequences. Science. 1979 Dec 7;206(4423):1148–1159. doi: 10.1126/science.388618. [DOI] [PubMed] [Google Scholar]
- Ramírez J. M., Campo F. F., Arnon D. I. Photosynthetic phosphorylation as energy source for protein synthesis and carbon dioxide assimilation by chloroplasts. Proc Natl Acad Sci U S A. 1968 Feb;59(2):606–612. doi: 10.1073/pnas.59.2.606. [DOI] [PMC free article] [PubMed] [Google Scholar]
- SHIN M., TAGAWA K., ARNON D. I. CRYSTALLIZATION OF FERREDOXIN-TPN REDUCTASE AND ITS ROLE IN THE PHOTOSYNTHETIC APPARATUS OF CHLOROPLASTS. Biochem Z. 1963;338:84–96. [PubMed] [Google Scholar]
- Sauer K., Biggins J. Action spectra and quantum yields for nicotinamide--adenine dinucleotide phosphate reduction by chloroplasts. Biochim Biophys Acta. 1965 May 25;102(1):55–72. doi: 10.1016/0926-6585(65)90202-5. [DOI] [PubMed] [Google Scholar]
- Sauer K., Park R. B. The Hill reaction of chloroplasts. Action spectra and quantum requirements. Biochemistry. 1965 Dec;4(12):2791–2798. doi: 10.1021/bi00888a032. [DOI] [PubMed] [Google Scholar]
- Schürmann P., Buchanan B. B., Arnon D. I. Role of cyclic photophosphorylation in photosynthetic carbon dioxide assimilation by isolated chloroplasts. Biochim Biophys Acta. 1972 Apr 20;267(1):111–124. doi: 10.1016/0005-2728(72)90143-0. [DOI] [PubMed] [Google Scholar]
- TAGAWA K., ARNON D. I. Ferredoxins as electron carriers in photosynthesis and in the biological production and consumption of hydrogen gas. Nature. 1962 Aug 11;195:537–543. doi: 10.1038/195537a0. [DOI] [PubMed] [Google Scholar]
- Terada H. Some biochemical and physiochemical properties of the potent uncoupler SF 6847 (3,5-di-tert-butyl-4-hydroxybenzylidenemalononitrile). Biochim Biophys Acta. 1975 Jun 17;387(3):519–532. doi: 10.1016/0005-2728(75)90090-0. [DOI] [PubMed] [Google Scholar]
- Tsujimoto H. Y., Hiyama T., Arnon D. I. Affinity of ferredoxin for electrons from water and the regulation of cyclic photophosphorylation. Biochem Biophys Res Commun. 1980 Mar 13;93(1):215–222. doi: 10.1016/s0006-291x(80)80268-3. [DOI] [PubMed] [Google Scholar]