Abstract
A new set of energy values to predict the secondary structures in RNA molecules has been derived through a multiple-step refinement procedure. It achieves more than 80% success in predicting the cloverleaf pattern in tRNA (200 sequences tested) and more than 60% success in predicting the consensus folding of 5S RNA (100 sequences). Improvements in our initial program for predicting secondary structures, based on the principle of the "incompatibility islets" made possible the work on 5S RNA. The program was speeded up by introducing a dynamic grouping of the islets into three disjoint blocks. The novel features in the energy model include i) an evaluation of the contribution of odd pairs according to their position within a segment ii) a penalty for internal loops related to their dissymmetry iii) a bonus for bulge loops when the two terminal paired bases at the junction point are both pyrimidines.
Full text
PDF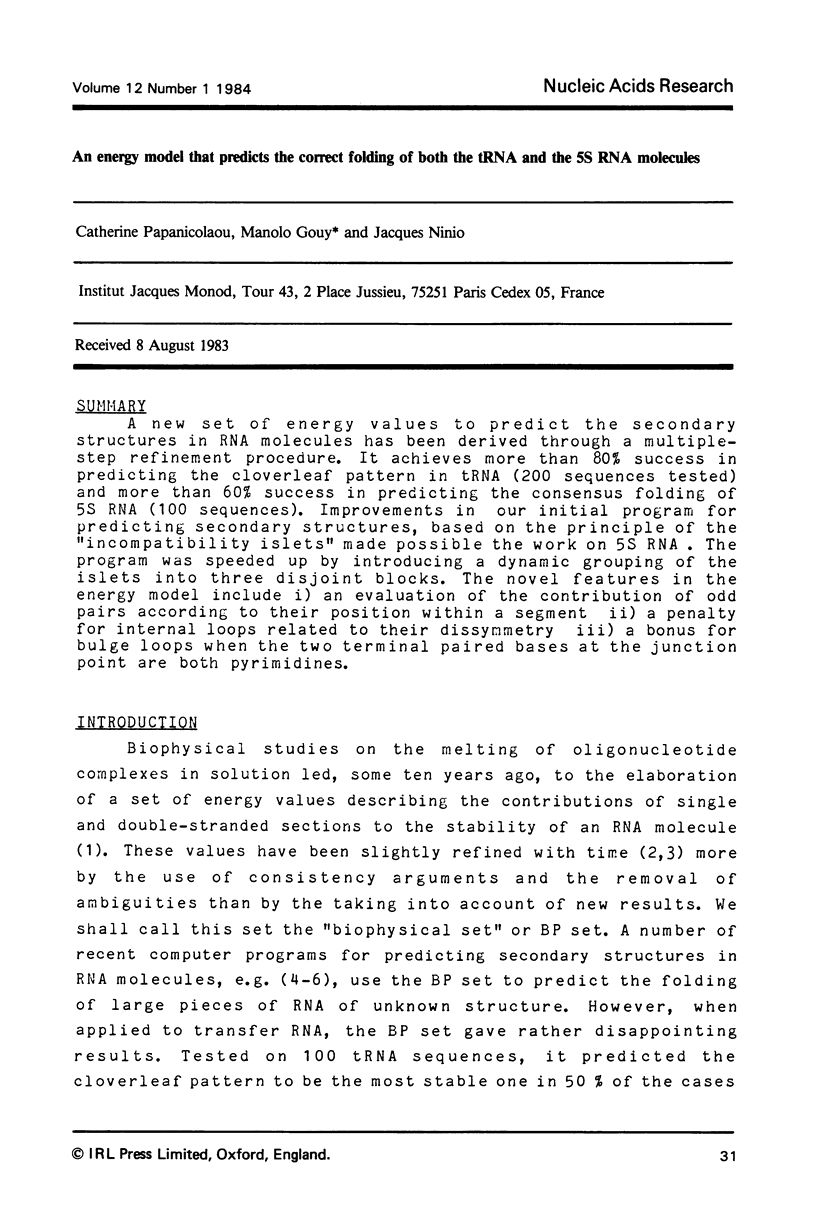
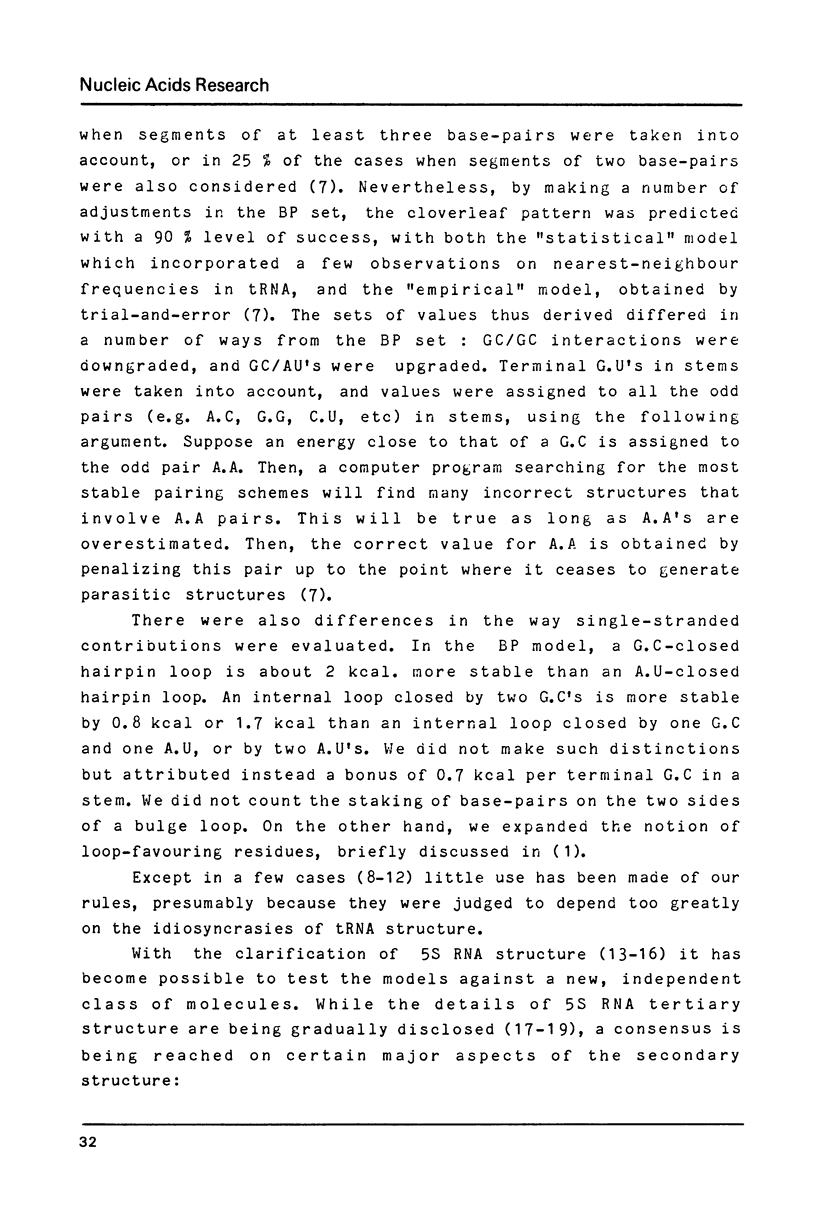
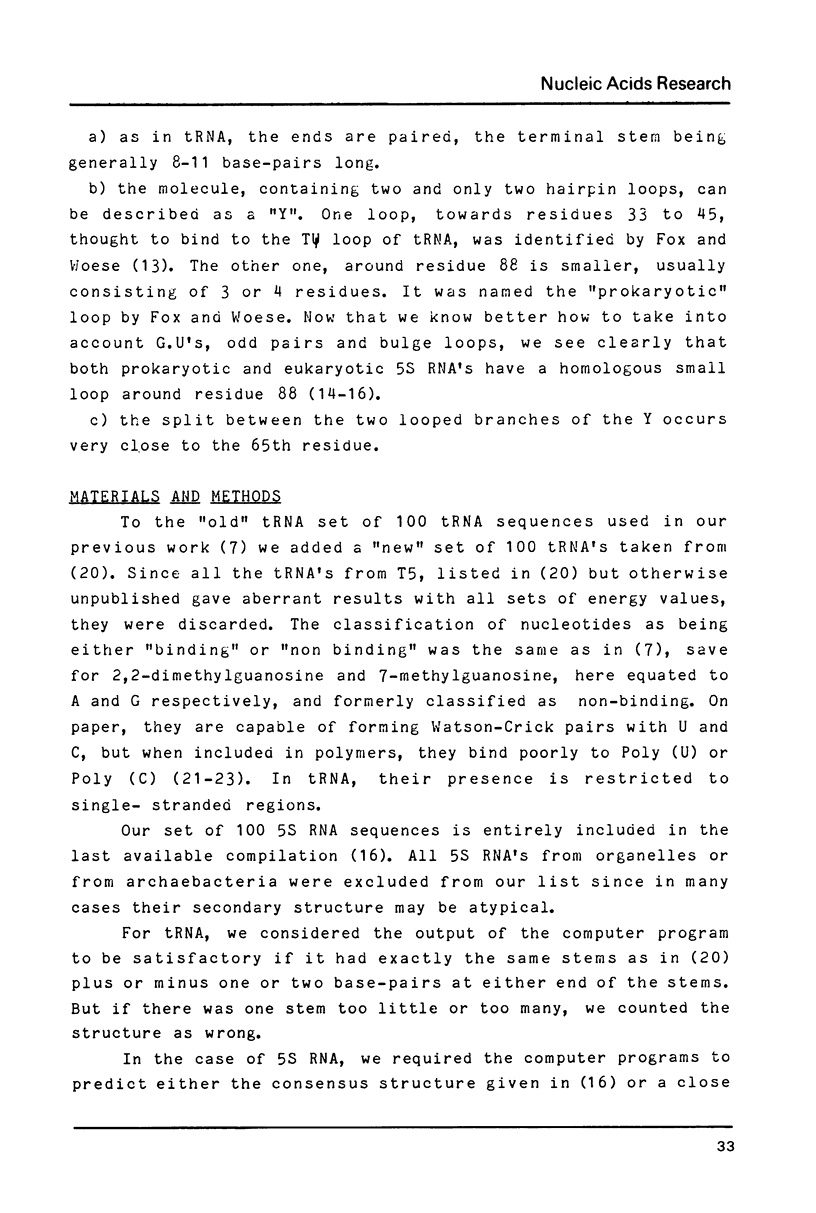
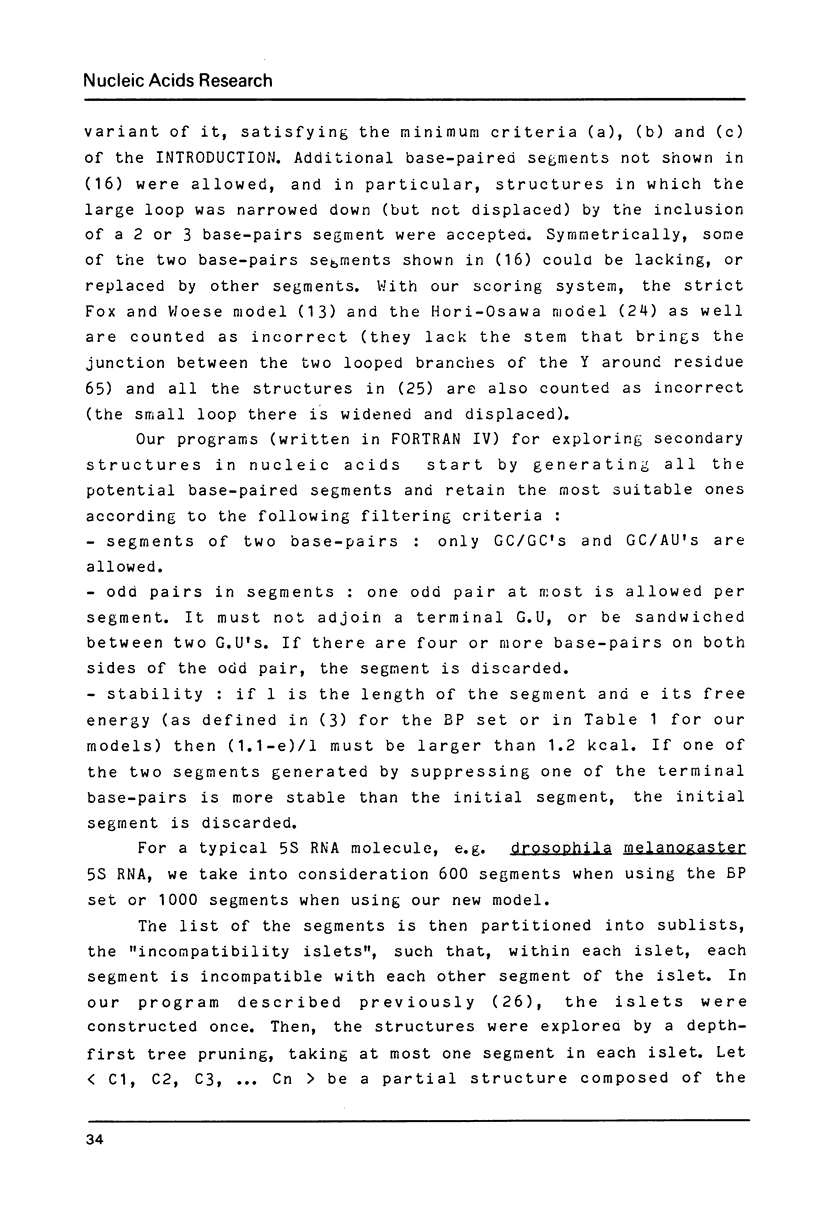
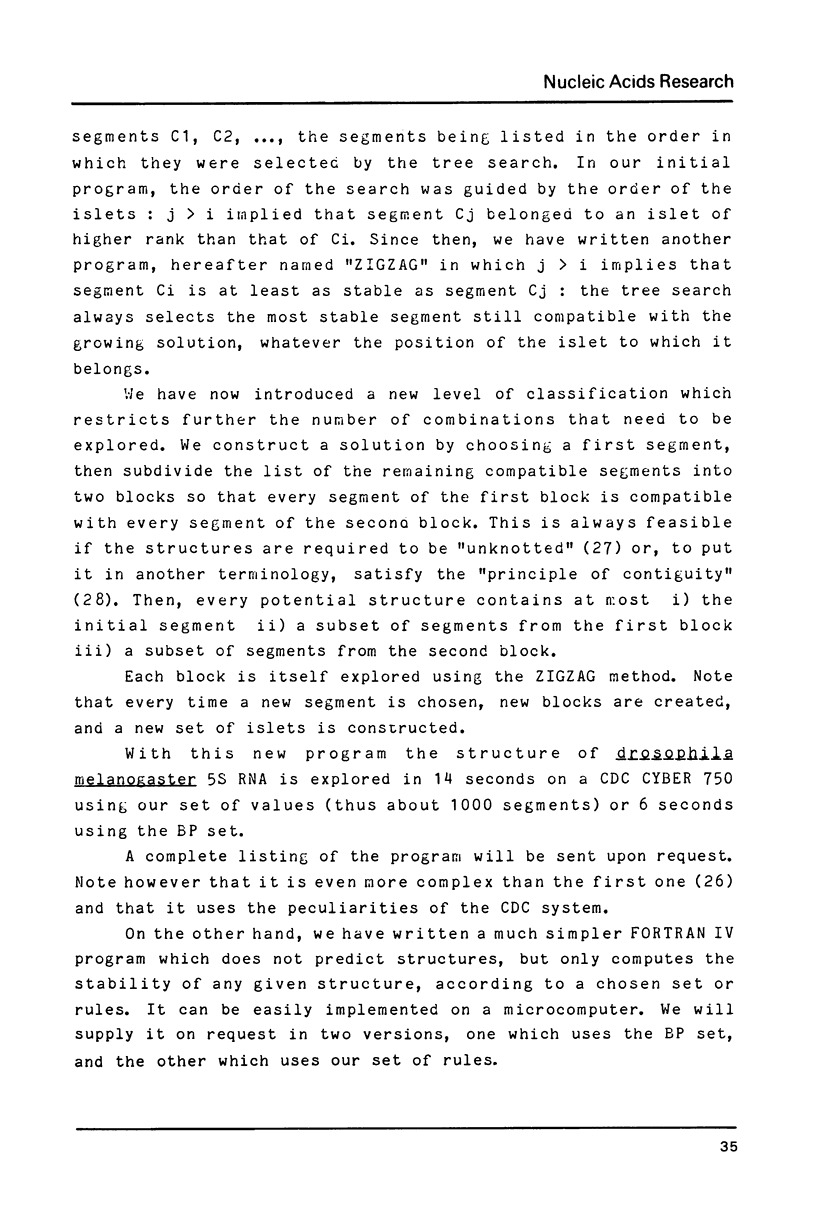
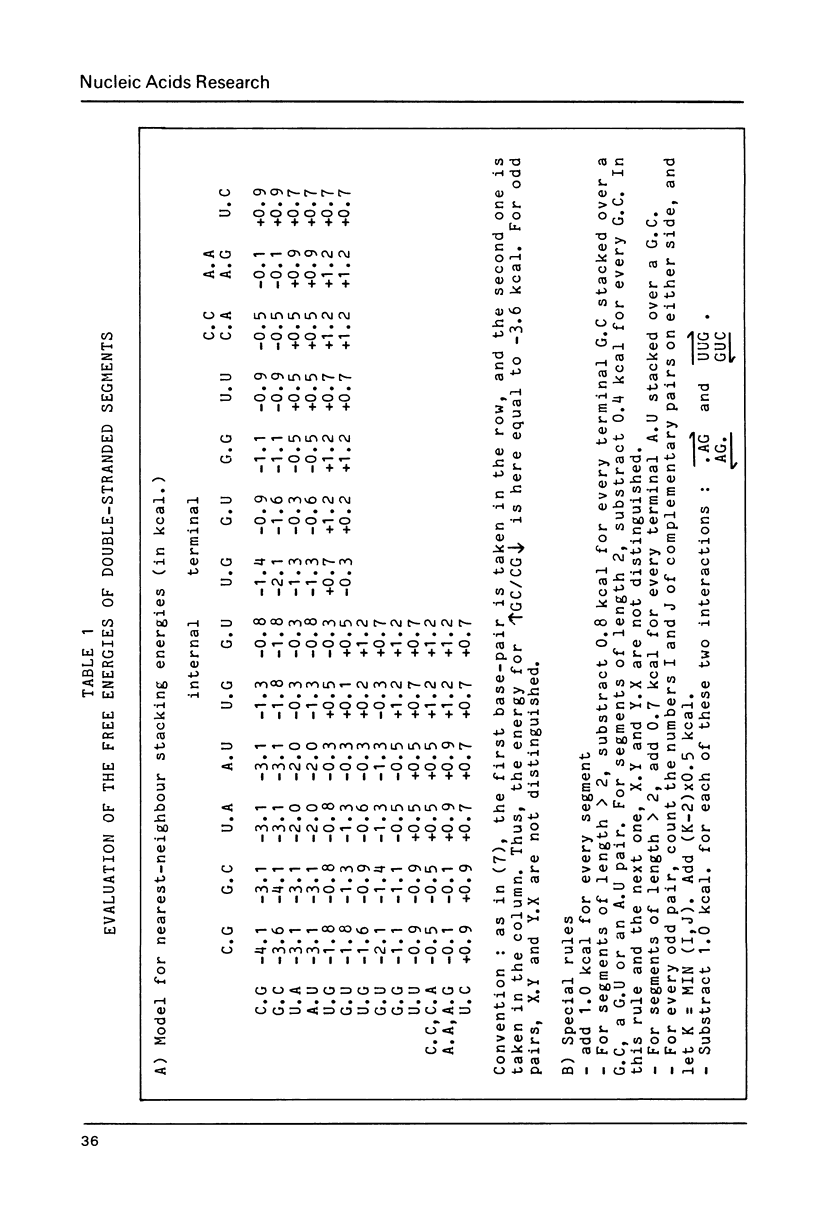
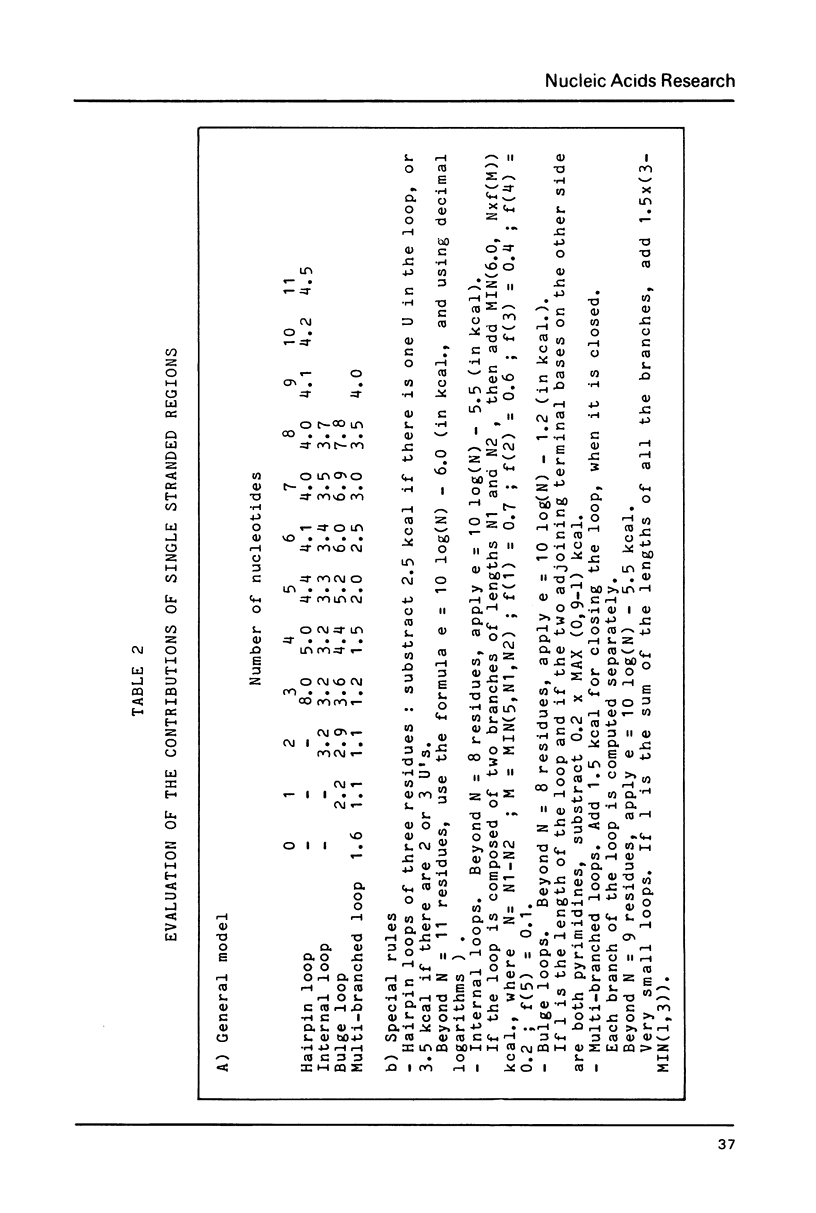
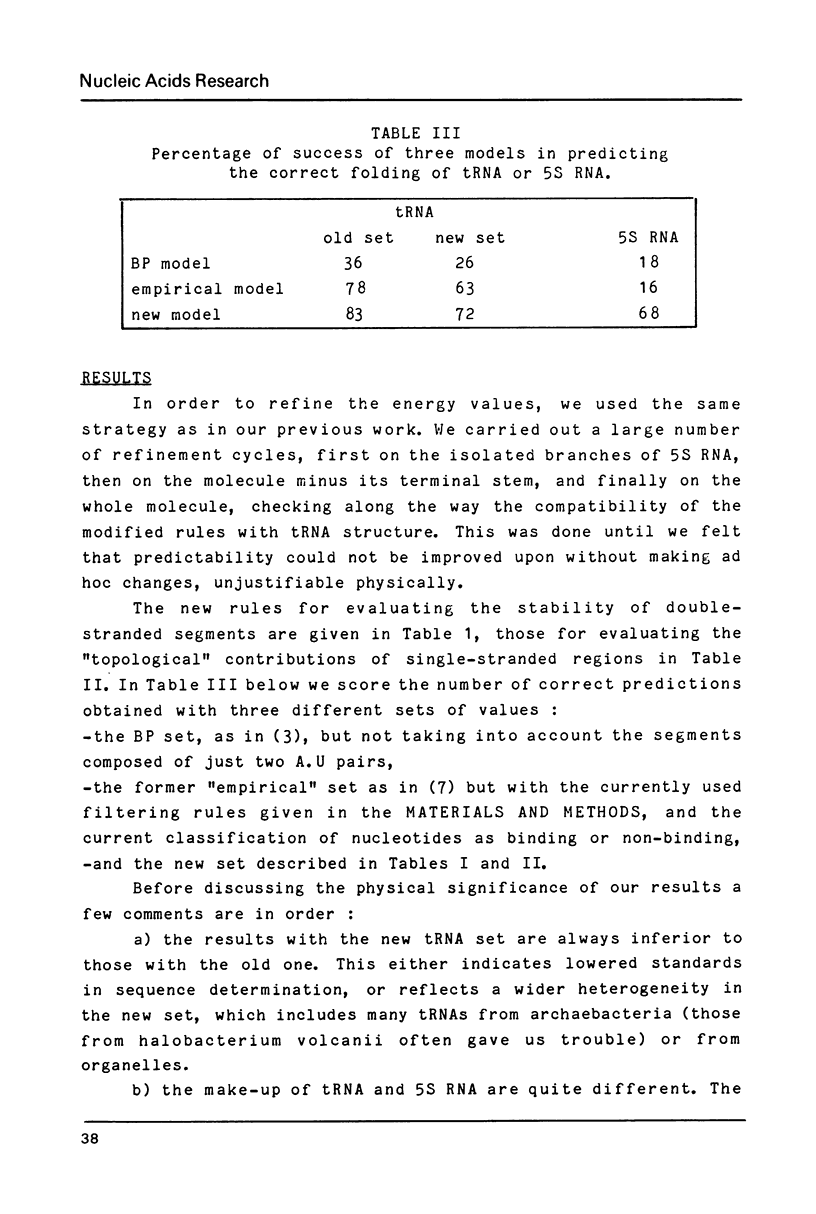
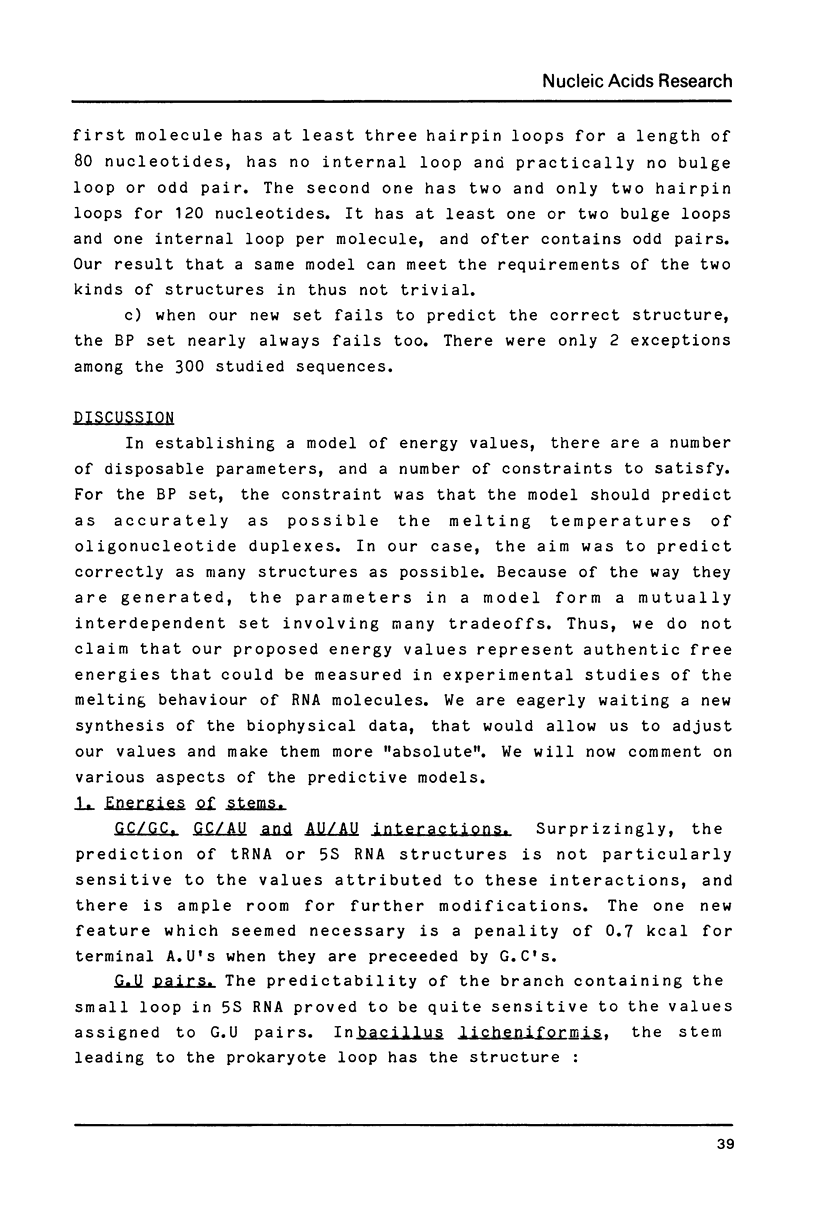
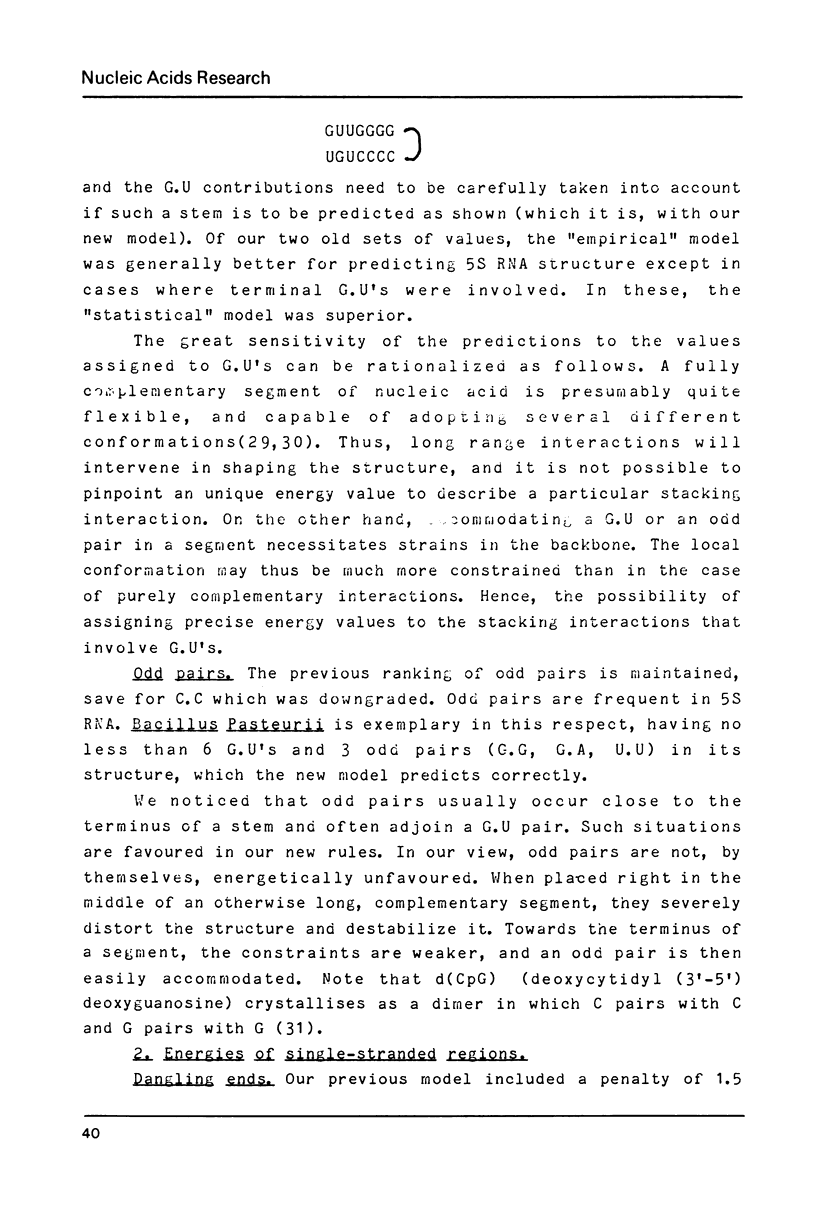
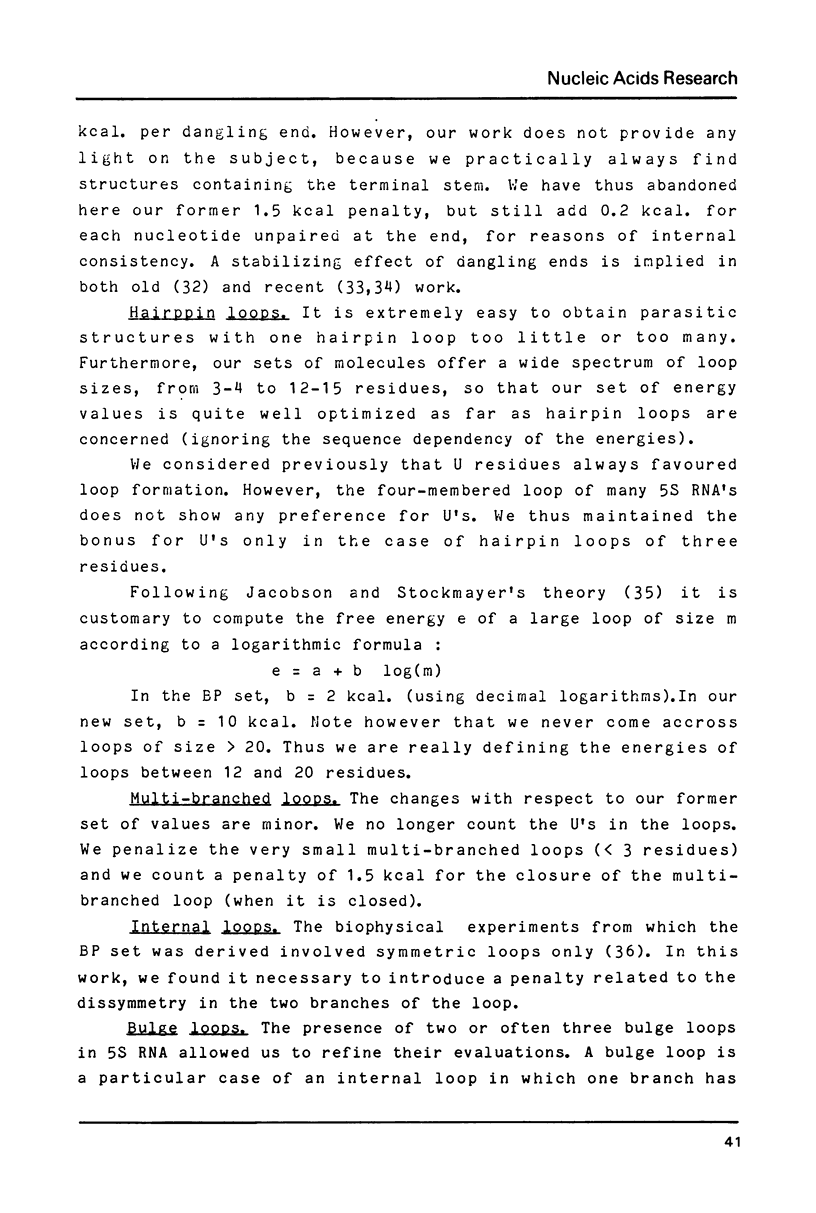
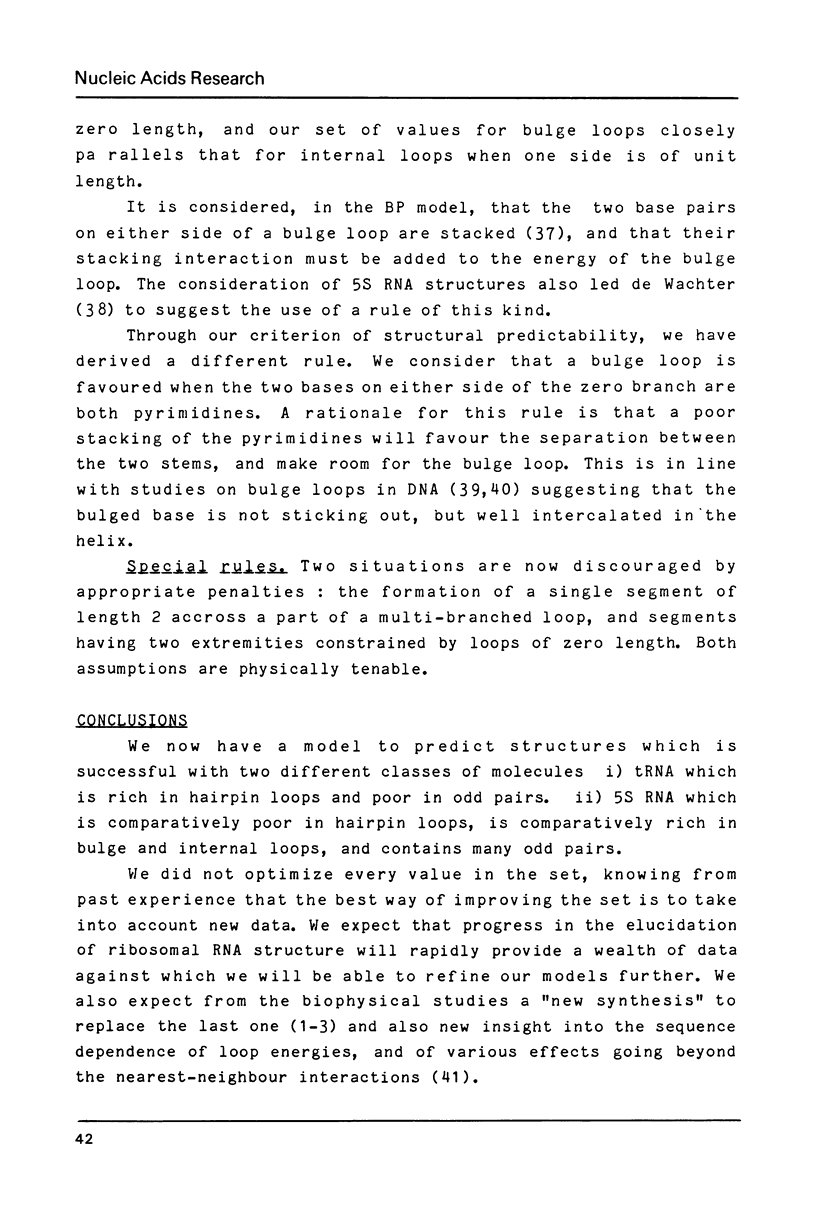
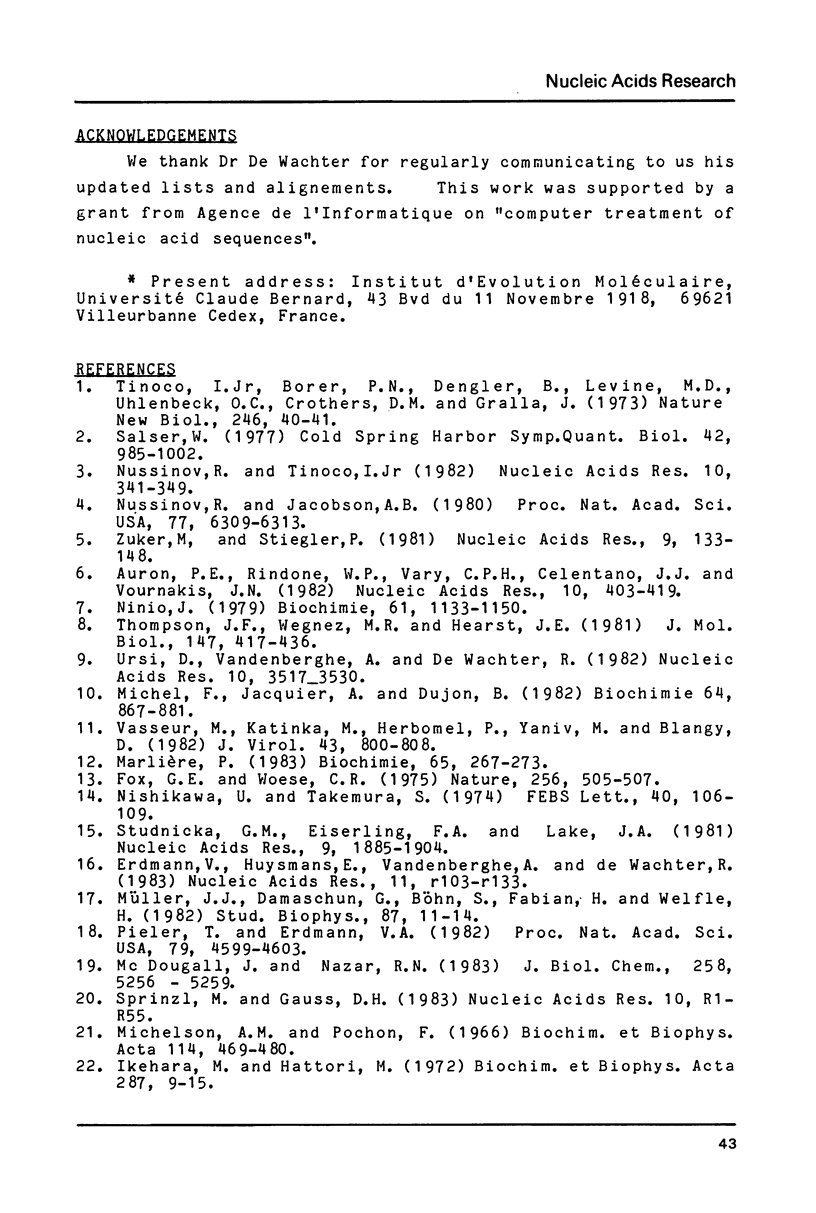
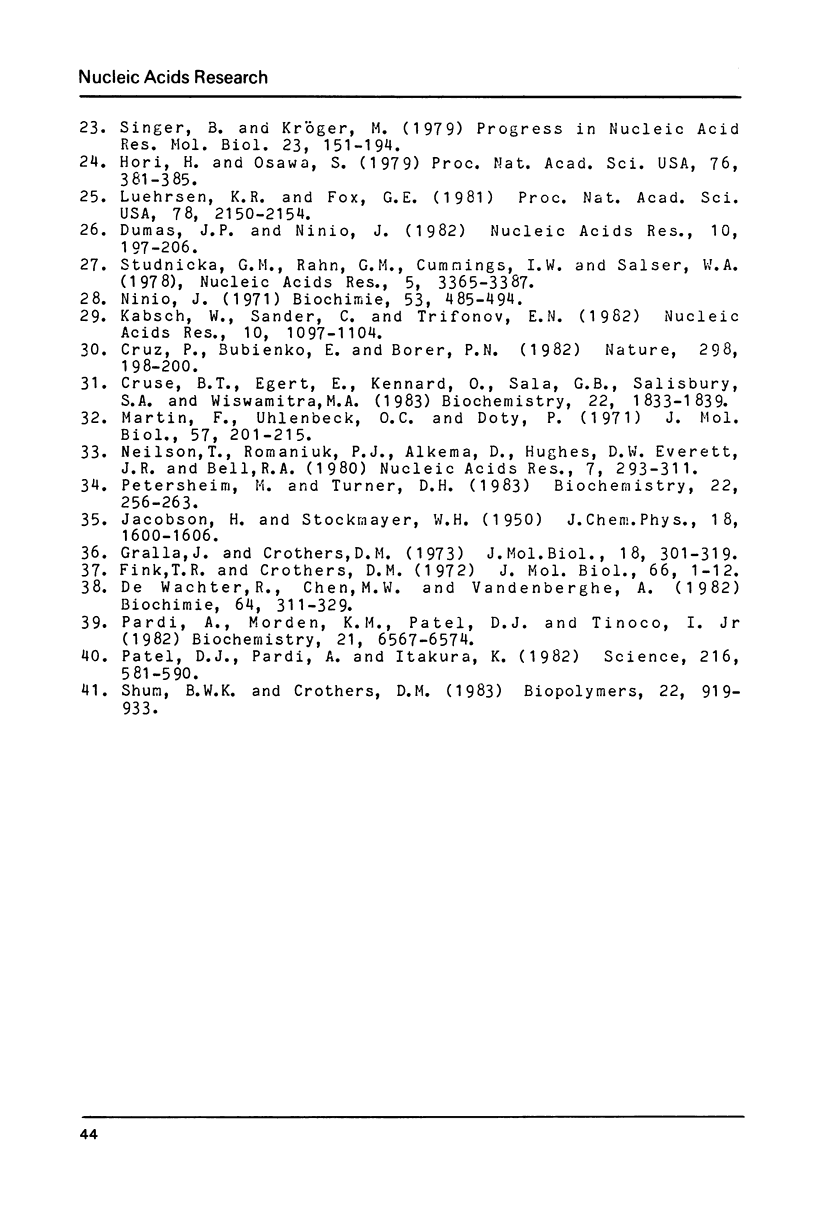
Selected References
These references are in PubMed. This may not be the complete list of references from this article.
- Auron P. E., Rindone W. P., Vary C. P., Celentano J. J., Vournakis J. N. Computer-aided prediction of RNA secondary structures. Nucleic Acids Res. 1982 Jan 11;10(1):403–419. doi: 10.1093/nar/10.1.403. [DOI] [PMC free article] [PubMed] [Google Scholar]
- Cruse W. B., Egert E., Kennard O., Sala G. B., Salisbury S. A., Viswamitra M. A. Self base pairing in a complementary deoxydinucleoside monophosphate duplex: crystal and molecular structure of deoxycytidylyl-(3'-5')-deoxyguanosine. Biochemistry. 1983 Apr 12;22(8):1833–1839. doi: 10.1021/bi00277a014. [DOI] [PubMed] [Google Scholar]
- Cruz P., Bubienko E., Borer P. N. A model for base overlap in RNA. Nature. 1982 Jul 8;298(5870):198–200. doi: 10.1038/298198a0. [DOI] [PubMed] [Google Scholar]
- Dumas J. P., Ninio J. Efficient algorithms for folding and comparing nucleic acid sequences. Nucleic Acids Res. 1982 Jan 11;10(1):197–206. doi: 10.1093/nar/10.1.197. [DOI] [PMC free article] [PubMed] [Google Scholar]
- Erdmann V. A., Huysmans E., Vandenberghe A., De Wachter R. Collection of published 5S and 5.8S ribosomal RNA sequences. Nucleic Acids Res. 1983 Jan 11;11(1):r105–r133. [PMC free article] [PubMed] [Google Scholar]
- Fink T. R., Crothers D. M. Free energy of imperfect nucleic acid helices. I. The bulge defect. J Mol Biol. 1972 Apr 28;66(1):1–12. doi: 10.1016/s0022-2836(72)80002-0. [DOI] [PubMed] [Google Scholar]
- Fox G. E., Woese C. R. 5S RNA secondary structure. Nature. 1975 Aug 7;256(5517):505–507. doi: 10.1038/256505a0. [DOI] [PubMed] [Google Scholar]
- Gralla J., Crothers D. M. Free energy of imperfect nucleic acid helices. 3. Small internal loops resulting from mismatches. J Mol Biol. 1973 Aug 5;78(2):301–319. doi: 10.1016/0022-2836(73)90118-6. [DOI] [PubMed] [Google Scholar]
- Hori H., Osawa S. Evolutionary change in 5S RNA secondary structure and a phylogenic tree of 54 5S RNA species. Proc Natl Acad Sci U S A. 1979 Jan;76(1):381–385. doi: 10.1073/pnas.76.1.381. [DOI] [PMC free article] [PubMed] [Google Scholar]
- Ikehara M., Hattori M. Polynucleotides. XV. Synthesis and properties of polynucleotides containing N 2 -dimethylguanylic acid residues in polyinosinate and polyadenylate chains. Biochim Biophys Acta. 1972 Nov 16;287(1):9–15. doi: 10.1016/0005-2787(72)90325-5. [DOI] [PubMed] [Google Scholar]
- Kabsch W., Sander C., Trifonov E. N. The ten helical twist angles of B-DNA. Nucleic Acids Res. 1982 Feb 11;10(3):1097–1104. doi: 10.1093/nar/10.3.1097. [DOI] [PMC free article] [PubMed] [Google Scholar]
- Luehrsen K. R., Fox G. E. Secondary structure of eukaryotic cytoplasmic 5S ribosomal RNA. Proc Natl Acad Sci U S A. 1981 Apr;78(4):2150–2154. doi: 10.1073/pnas.78.4.2150. [DOI] [PMC free article] [PubMed] [Google Scholar]
- Marlière P. Computer building and folding of fictitious transfer-RNA sequences. Biochimie. 1983 Apr-May;65(4-5):267–273. doi: 10.1016/s0300-9084(83)80278-8. [DOI] [PubMed] [Google Scholar]
- Martin F. H., Uhlenbeck O. C., Doty P. Self-complementary oligoribonucleotides: adenylic acid-uridylic acid block copolymers. J Mol Biol. 1971 Apr 28;57(2):201–215. doi: 10.1016/0022-2836(71)90341-x. [DOI] [PubMed] [Google Scholar]
- McDougall J., Nazar R. N. Tertiary structure of the eukaryotic ribosomal 5 S RNA. Accessibility of phosphodiester bonds to ethylnitrosourea modification. J Biol Chem. 1983 Apr 25;258(8):5256–5259. [PubMed] [Google Scholar]
- Michel F., Jacquier A., Dujon B. Comparison of fungal mitochondrial introns reveals extensive homologies in RNA secondary structure. Biochimie. 1982 Oct;64(10):867–881. doi: 10.1016/s0300-9084(82)80349-0. [DOI] [PubMed] [Google Scholar]
- Michelson A. M., Pochon F. Polynucleotide analogues. VII. Methylation of polynucleotides. Biochim Biophys Acta. 1966 Mar 21;114(3):469–480. [PubMed] [Google Scholar]
- Neilson T., Romaniuk P. J., Alkema D., Hughes D. W., Everett J. R., Bell R. A. The effects of base sequence and dangling bases on the stability of short ribonucleic acid duplexes. Nucleic Acids Symp Ser. 1980;(7):293–311. [PubMed] [Google Scholar]
- Ninio J. Prediction of pairing schemes in RNA molecules-loop contributions and energy of wobble and non-wobble pairs. Biochimie. 1979;61(10):1133–1150. doi: 10.1016/s0300-9084(80)80227-6. [DOI] [PubMed] [Google Scholar]
- Ninio J. Properties of nucleic acid representations. I. Topology. Biochimie. 1971;53(4):485–494. [PubMed] [Google Scholar]
- Nishikawa K., Takemura S. Nucleotide sequence of 5 S RNA from Torulopsis utilis. FEBS Lett. 1974 Mar 15;40(1):106–109. doi: 10.1016/0014-5793(74)80904-x. [DOI] [PubMed] [Google Scholar]
- Nussinov R., Jacobson A. B. Fast algorithm for predicting the secondary structure of single-stranded RNA. Proc Natl Acad Sci U S A. 1980 Nov;77(11):6309–6313. doi: 10.1073/pnas.77.11.6309. [DOI] [PMC free article] [PubMed] [Google Scholar]
- Nussinov R., Tinoco I., Jr, Jacobson A. B. Small changes in free energy assignments for unpaired bases do not affect predicted secondary structures in single stranded RNA. Nucleic Acids Res. 1982 Jan 11;10(1):341–349. doi: 10.1093/nar/10.1.341. [DOI] [PMC free article] [PubMed] [Google Scholar]
- Pardi A., Morden K. M., Patel D. J., Tinoco I., Jr Kinetics for exchange of imino protons in the d(C-G-C-G-A-A-T-T-C-G-C-G) double helix and in two similar helices that contain a G . T base pair, d(C-G-T-G-A-A-T-T-C-G-C-G), and an extra adenine, d(C-G-C-A-G-A-A-T-T-C-G-C-G). Biochemistry. 1982 Dec 7;21(25):6567–6574. doi: 10.1021/bi00268a038. [DOI] [PubMed] [Google Scholar]
- Pieler T., Erdmann V. A. Three-dimensional structural model of eubacterial 5S RNA that has functional implications. Proc Natl Acad Sci U S A. 1982 Aug;79(15):4599–4603. doi: 10.1073/pnas.79.15.4599. [DOI] [PMC free article] [PubMed] [Google Scholar]
- Salser W. Globin mRNA sequences: analysis of base pairing and evolutionary implications. Cold Spring Harb Symp Quant Biol. 1978;42(Pt 2):985–1002. doi: 10.1101/sqb.1978.042.01.099. [DOI] [PubMed] [Google Scholar]
- Shum B. W., Crothers D. M. High-resolution NMR studies of A- and G-containing oligonucleotides. Biopolymers. 1983 Mar;22(3):919–933. doi: 10.1002/bip.360220313. [DOI] [PubMed] [Google Scholar]
- Singer B., Kröger M. Participation of modified nucleosides in translation and transcription. Prog Nucleic Acid Res Mol Biol. 1979;23:151–194. doi: 10.1016/s0079-6603(08)60133-6. [DOI] [PubMed] [Google Scholar]
- Studnicka G. M., Eiserling F. A., Lake J. A. A unique secondary folding pattern for 5S RNA corresponds to the lowest energy homologous secondary structure in 17 different prokaryotes. Nucleic Acids Res. 1981 Apr 24;9(8):1885–1904. doi: 10.1093/nar/9.8.1885. [DOI] [PMC free article] [PubMed] [Google Scholar]
- Studnicka G. M., Rahn G. M., Cummings I. W., Salser W. A. Computer method for predicting the secondary structure of single-stranded RNA. Nucleic Acids Res. 1978 Sep;5(9):3365–3387. doi: 10.1093/nar/5.9.3365. [DOI] [PMC free article] [PubMed] [Google Scholar]
- Thompson J. F., Wegnez M. R., Hearst J. E. Determination of the secondary structure of Drosophila melanogaster 5 S RNA by hydroxymethyltrimethylpsoralen crosslinking. J Mol Biol. 1981 Apr 15;147(3):417–436. doi: 10.1016/0022-2836(81)90493-9. [DOI] [PubMed] [Google Scholar]
- Tinoco I., Jr, Borer P. N., Dengler B., Levin M. D., Uhlenbeck O. C., Crothers D. M., Bralla J. Improved estimation of secondary structure in ribonucleic acids. Nat New Biol. 1973 Nov 14;246(150):40–41. doi: 10.1038/newbio246040a0. [DOI] [PubMed] [Google Scholar]
- Ursi D., Vandenberghe A., De Wachter R. The sequence of the 5.8 S ribosomal RNA of the crustacean Artemia salina. With a proposal for a general secondary structure model for 5.8 S ribosomal RNA. Nucleic Acids Res. 1982 Jun 11;10(11):3517–3530. doi: 10.1093/nar/10.11.3517. [DOI] [PMC free article] [PubMed] [Google Scholar]
- Vasseur M., Katinka M., Herbomel P., Yaniv M., Blangy D. Physical and biological features of polyoma virus mutants able to infect embryonal carcinoma cell lines. J Virol. 1982 Sep;43(3):800–808. doi: 10.1128/jvi.43.3.800-808.1982. [DOI] [PMC free article] [PubMed] [Google Scholar]
- Zuker M., Stiegler P. Optimal computer folding of large RNA sequences using thermodynamics and auxiliary information. Nucleic Acids Res. 1981 Jan 10;9(1):133–148. doi: 10.1093/nar/9.1.133. [DOI] [PMC free article] [PubMed] [Google Scholar]