Abstract
Microtubule proteins isolated from calf brain will undergo gelation-contraction in the presence of ATP. We have now examined this process by video-enhanced contrast microscopy. After ATP addition to steady-state microtubules, slow (1-5 micron/min), linear movements of particles and microtubules toward aggregation centers occur. The resulting structures resemble mitotic spindle asters. During the time when gel contraction occurs, asters move (at 1-5 micron/min) toward other nearby asters. This is accompanied by the apparent shortening of the microtubules running between the asters. This is the first example of isolated microtubules undergoing a process that has similarities to half-spindle shortening during anaphase A. Formation of aster-like structures without preformed microtubule organizing centers raises the possibility that a similar process may contribute to microtubule organization in vivo.
Full text
PDF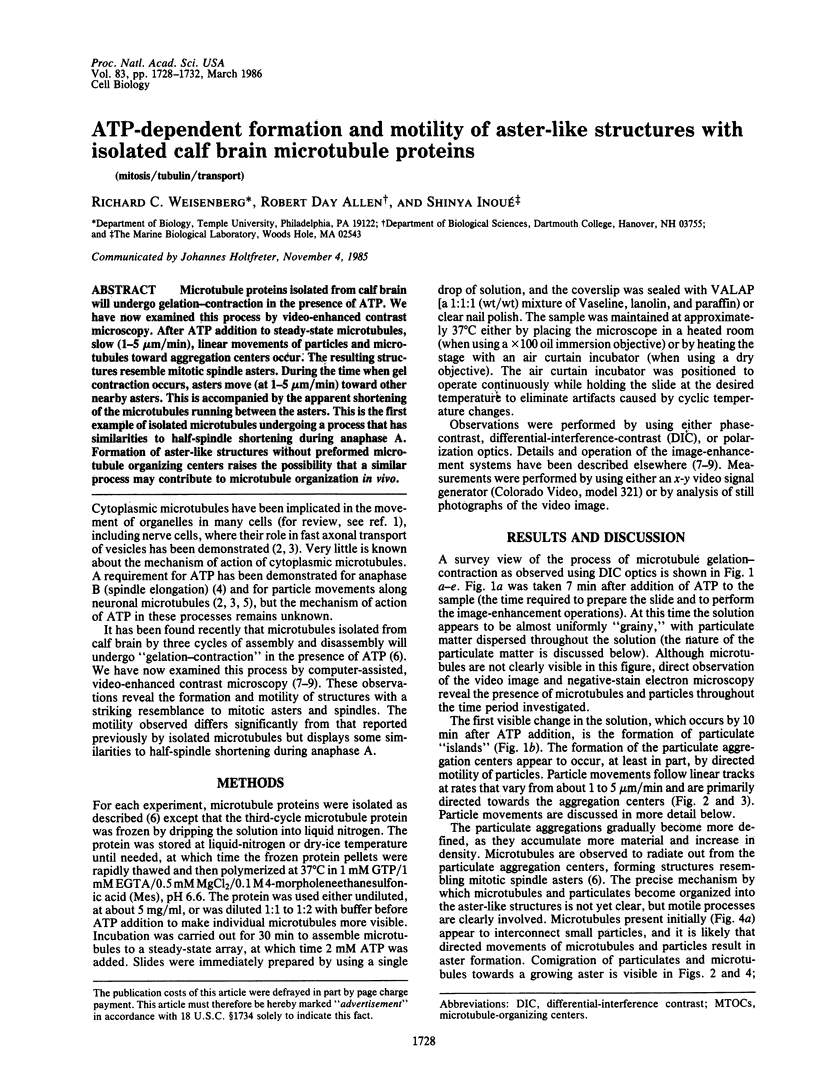
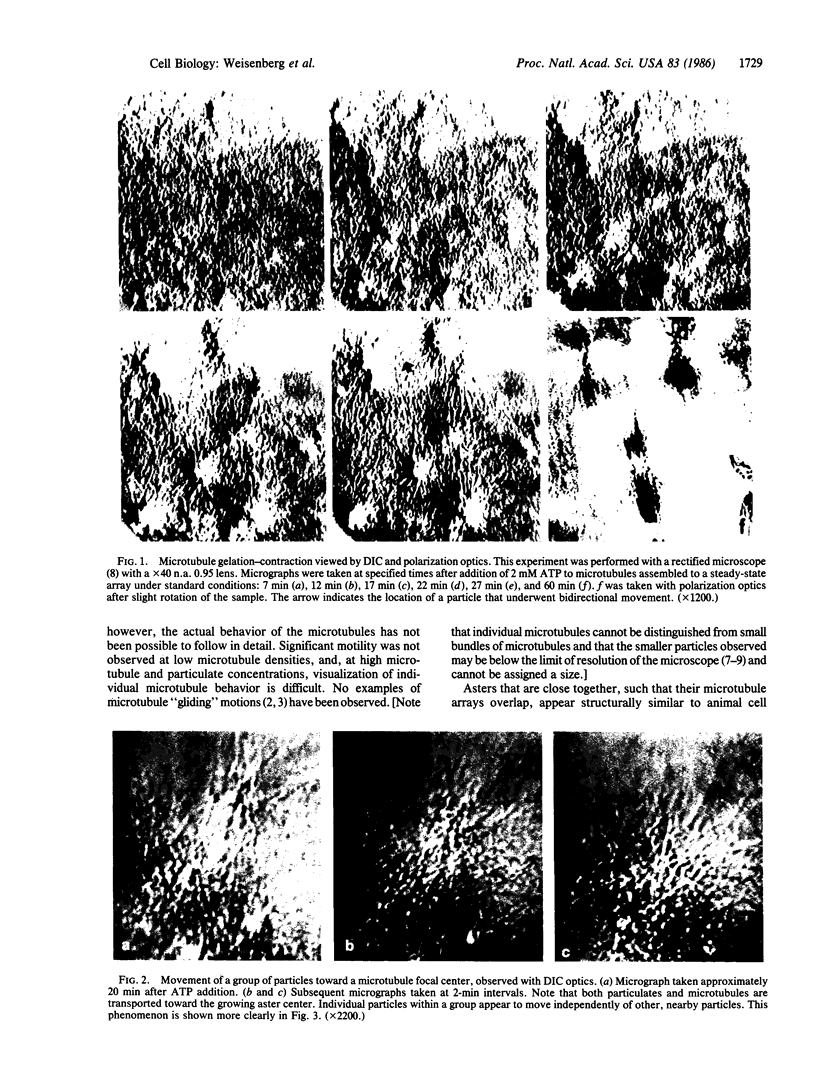
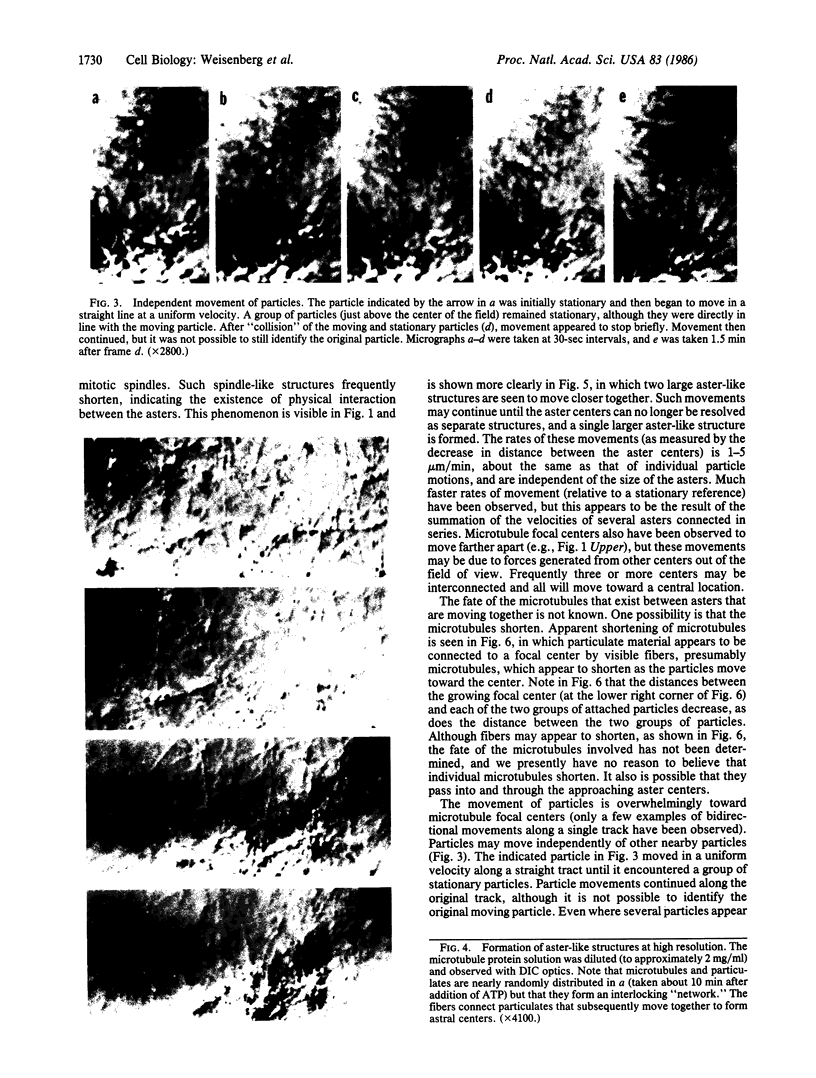
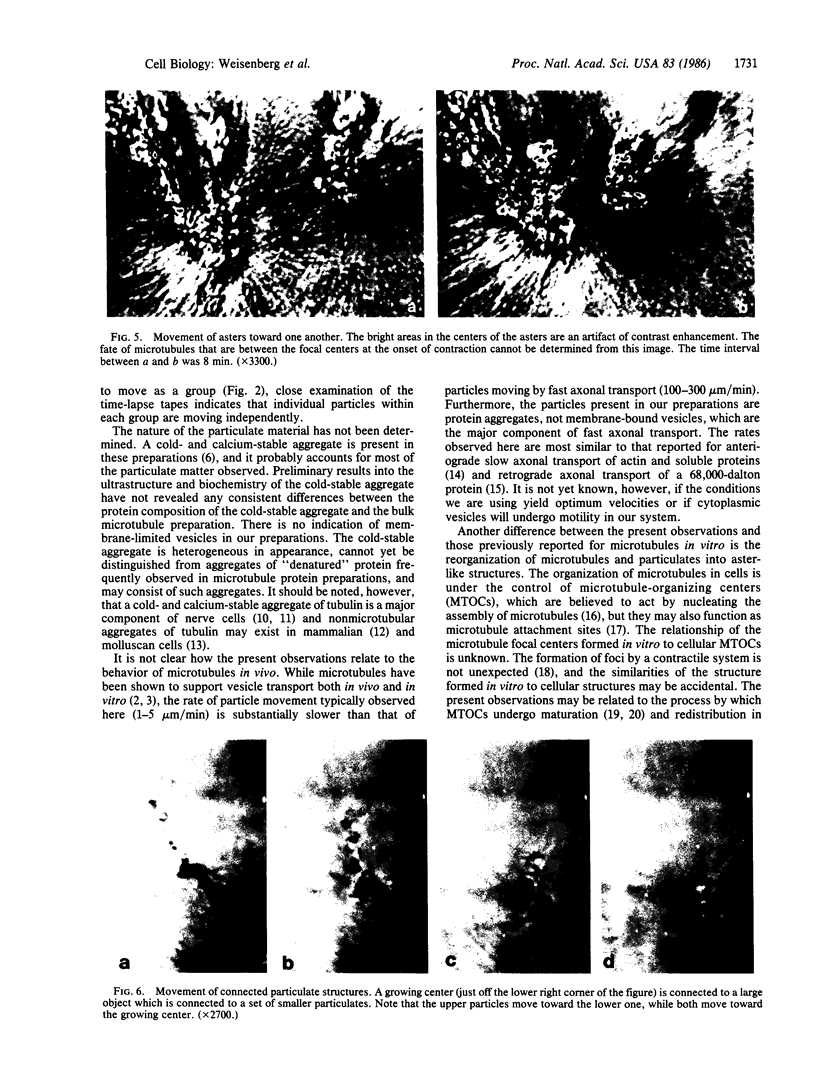
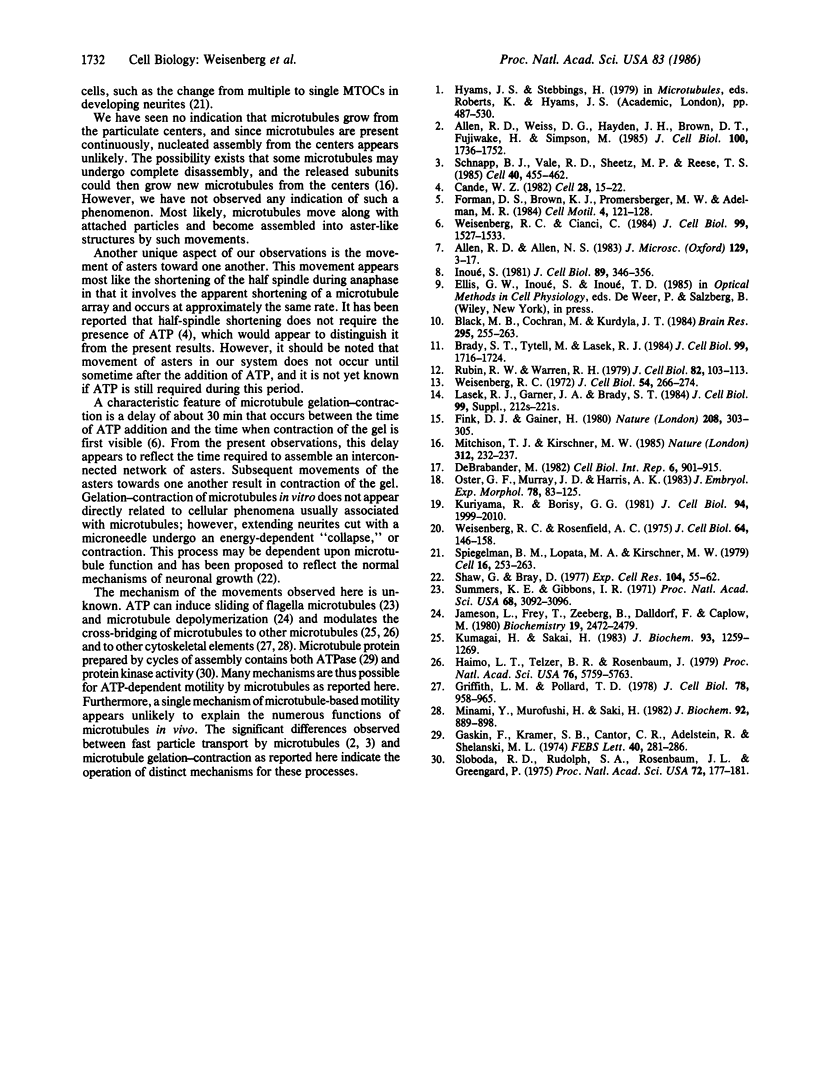
Images in this article
Selected References
These references are in PubMed. This may not be the complete list of references from this article.
- Allen R. D., Allen N. S. Video-enhanced microscopy with a computer frame memory. J Microsc. 1983 Jan;129(Pt 1):3–17. doi: 10.1111/j.1365-2818.1983.tb04157.x. [DOI] [PubMed] [Google Scholar]
- Allen R. D., Weiss D. G., Hayden J. H., Brown D. T., Fujiwake H., Simpson M. Gliding movement of and bidirectional transport along single native microtubules from squid axoplasm: evidence for an active role of microtubules in cytoplasmic transport. J Cell Biol. 1985 May;100(5):1736–1752. doi: 10.1083/jcb.100.5.1736. [DOI] [PMC free article] [PubMed] [Google Scholar]
- Black M. M., Cochran J. M., Kurdyla J. T. Solubility properties of neuronal tubulin: evidence for labile and stable microtubules. Brain Res. 1984 Mar 19;295(2):255–263. doi: 10.1016/0006-8993(84)90974-0. [DOI] [PubMed] [Google Scholar]
- Brady S. T., Tytell M., Lasek R. J. Axonal tubulin and axonal microtubules: biochemical evidence for cold stability. J Cell Biol. 1984 Nov;99(5):1716–1724. doi: 10.1083/jcb.99.5.1716. [DOI] [PMC free article] [PubMed] [Google Scholar]
- Cande W. Z. Nucleotide requirements for anaphase chromosome movements in permeabilized mitotic cells: anaphase B but not anaphase A requires ATP. Cell. 1982 Jan;28(1):15–22. doi: 10.1016/0092-8674(82)90370-1. [DOI] [PubMed] [Google Scholar]
- De Brabander M. A model for the microtubule organizing activity of the centrosomes and kinetochores in mammalian cells. Cell Biol Int Rep. 1982 Oct;6(10):901–915. doi: 10.1016/0309-1651(82)90001-7. [DOI] [PubMed] [Google Scholar]
- Fink D. J., Gainer H. Retrograde axonal transport of endogenous proteins in sciatic nerve demonstrated by covalent labeling in vivo. Science. 1980 Apr 18;208(4441):303–305. doi: 10.1126/science.6154312. [DOI] [PubMed] [Google Scholar]
- Forman D. S., Brown K. J., Promersberger M. W., Adelman M. R. Nucleotide specificity for reactivation of organelle movements in permeabilized axons. Cell Motil. 1984;4(2):121–128. doi: 10.1002/cm.970040205. [DOI] [PubMed] [Google Scholar]
- Gaskin F., Kramer S. B., Cantor C. R., Adelstein R., Shelanski M. L. A dynein-like protein associated with neurotubules. FEBS Lett. 1974 Apr 1;40(2):281–286. doi: 10.1016/0014-5793(74)80244-9. [DOI] [PubMed] [Google Scholar]
- Griffith L. M., Pollard T. D. Evidence for actin filament-microtubule interaction mediated by microtubule-associated proteins. J Cell Biol. 1978 Sep;78(3):958–965. doi: 10.1083/jcb.78.3.958. [DOI] [PMC free article] [PubMed] [Google Scholar]
- Haimo L. T., Telzer B. R., Rosenbaum J. L. Dynein binds to and crossbridges cytoplasmic microtubules. Proc Natl Acad Sci U S A. 1979 Nov;76(11):5759–5763. doi: 10.1073/pnas.76.11.5759. [DOI] [PMC free article] [PubMed] [Google Scholar]
- Inoué S. Video image processing greatly enhances contrast, quality, and speed in polarization-based microscopy. J Cell Biol. 1981 May;89(2):346–356. doi: 10.1083/jcb.89.2.346. [DOI] [PMC free article] [PubMed] [Google Scholar]
- Jameson L., Frey T., Zeeberg B., Dalldorf F., Caplow M. Inhibition of microtubule assembly by phosphorylation of microtubule-associated proteins. Biochemistry. 1980 May 27;19(11):2472–2479. doi: 10.1021/bi00552a027. [DOI] [PubMed] [Google Scholar]
- Kumagai H., Sakai H. A porcine brain protein (35 K protein) which bundles microtubules and its identification as glyceraldehyde 3-phosphate dehydrogenase. J Biochem. 1983 May;93(5):1259–1269. doi: 10.1093/oxfordjournals.jbchem.a134260. [DOI] [PubMed] [Google Scholar]
- Lasek R. J., Garner J. A., Brady S. T. Axonal transport of the cytoplasmic matrix. J Cell Biol. 1984 Jul;99(1 Pt 2):212s–221s. doi: 10.1083/jcb.99.1.212s. [DOI] [PMC free article] [PubMed] [Google Scholar]
- Minami Y., Murofushi H., Sakai H. Interaction of tubulin with neurofilaments: formation of networks by neurofilament-dependent tubulin polymerization. J Biochem. 1982 Sep;92(3):889–898. doi: 10.1093/oxfordjournals.jbchem.a134003. [DOI] [PubMed] [Google Scholar]
- Mitchison T., Kirschner M. Microtubule assembly nucleated by isolated centrosomes. Nature. 1984 Nov 15;312(5991):232–237. doi: 10.1038/312232a0. [DOI] [PubMed] [Google Scholar]
- Oster G. F., Murray J. D., Harris A. K. Mechanical aspects of mesenchymal morphogenesis. J Embryol Exp Morphol. 1983 Dec;78:83–125. [PubMed] [Google Scholar]
- Rubin R. W., Warren R. H. Organization of tubulin in normal and transformed rat kidney cells. J Cell Biol. 1979 Jul;82(1):103–113. doi: 10.1083/jcb.82.1.103. [DOI] [PMC free article] [PubMed] [Google Scholar]
- Schnapp B. J., Vale R. D., Sheetz M. P., Reese T. S. Single microtubules from squid axoplasm support bidirectional movement of organelles. Cell. 1985 Feb;40(2):455–462. doi: 10.1016/0092-8674(85)90160-6. [DOI] [PubMed] [Google Scholar]
- Shaw G., Bray D. Movement and extension of isolated growth cones. Exp Cell Res. 1977 Jan;104(1):55–62. doi: 10.1016/0014-4827(77)90068-4. [DOI] [PubMed] [Google Scholar]
- Sloboda R. D., Rudolph S. A., Rosenbaum J. L., Greengard P. Cyclic AMP-dependent endogenous phosphorylation of a microtubule-associated protein. Proc Natl Acad Sci U S A. 1975 Jan;72(1):177–181. doi: 10.1073/pnas.72.1.177. [DOI] [PMC free article] [PubMed] [Google Scholar]
- Spiegelman B. M., Lopata M. A., Kirschner M. W. Aggregation of microtubule initiation sites preceding neurite outgrowth in mouse neuroblastoma cells. Cell. 1979 Feb;16(2):253–263. doi: 10.1016/0092-8674(79)90003-5. [DOI] [PubMed] [Google Scholar]
- Summers K. E., Gibbons I. R. Adenosine triphosphate-induced sliding of tubules in trypsin-treated flagella of sea-urchin sperm. Proc Natl Acad Sci U S A. 1971 Dec;68(12):3092–3096. doi: 10.1073/pnas.68.12.3092. [DOI] [PMC free article] [PubMed] [Google Scholar]
- Weisenberg R. C. Changes in the organization of tubulin during meiosis in the eggs of the surf clam, Spisula solidissima. J Cell Biol. 1972 Aug;54(2):266–278. doi: 10.1083/jcb.54.2.266. [DOI] [PMC free article] [PubMed] [Google Scholar]
- Weisenberg R. C., Cianci C. ATP-induced gelation--contraction of microtubules assembled in vitro. J Cell Biol. 1984 Oct;99(4 Pt 1):1527–1533. doi: 10.1083/jcb.99.4.1527. [DOI] [PMC free article] [PubMed] [Google Scholar]
- Weisenberg R. C., Rosenfeld A. C. In vitro polymerization of microtubules into asters and spindles in homogenates of surf clam eggs. J Cell Biol. 1975 Jan;64(1):146–158. doi: 10.1083/jcb.64.1.146. [DOI] [PMC free article] [PubMed] [Google Scholar]