Abstract
Striatal neurons were cultured from the fetal mouse brain and maintained in serum-free medium for 14-21 days in vitro (DIV). Pretreatment of the culture dishes successively with a polycation followed by fetal calf serum resulted in rapid neuron attachment and neurite proliferation. After 9-10 DIV, electron microscope observations revealed the presence of vesicles in axon terminals forming mature synapses with axons and perikarya of adjacent neurons and in varicosities along extended axons. Synapsin I, a synaptic vesicle-specific protein, was present only in neuronal perikarya after 3 DIV, in perikarya and in varicosities along extended axons after 6 DIV, and in varicosities and contact points between axon terminals and adjacent axons or perikarya after 11-14 DIV. Neurotransmitter-stimulated intracellular formation of cAMP decreased markedly during neuronal differentiation. Inositol phosphate formation in response to neurotransmitters, however, increased significantly throughout the period of striatal neuronal development. K+ (56 mM) depolarization resulted in a 2-fold increase in endogenous gamma-aminobutyric acid (GABA) release from striatal neurons, 50% of which was Ca2+-dependent, between 3 and 11 DIV. Between 11 and 14 DIV, subsequent to synapse formation (as revealed by electron microscope observations), GABA release evoked by 56 mM K+ increased up to 5-fold, 75% of which was Ca2+-dependent. It appears that the complete differentiation of striatal neurons in serum-free medium may provide a suitable model for the study of the physiological and regulatory mechanisms involved in nerve cell development.
Full text
PDF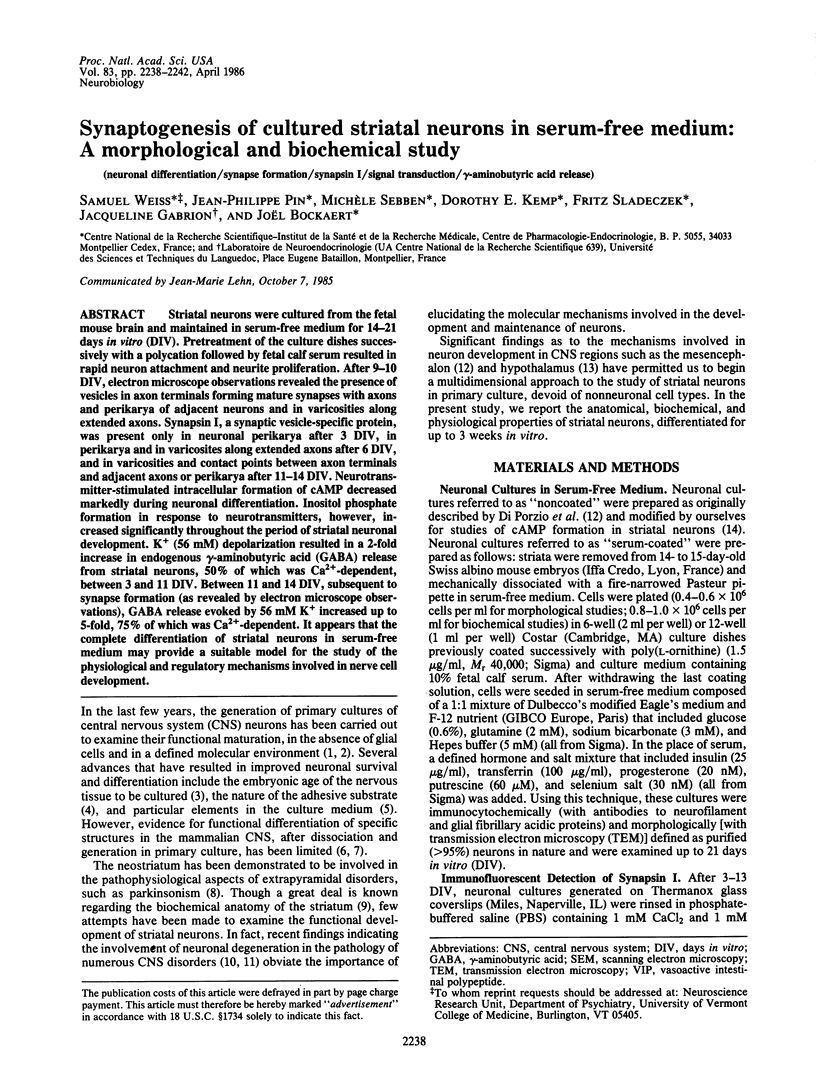
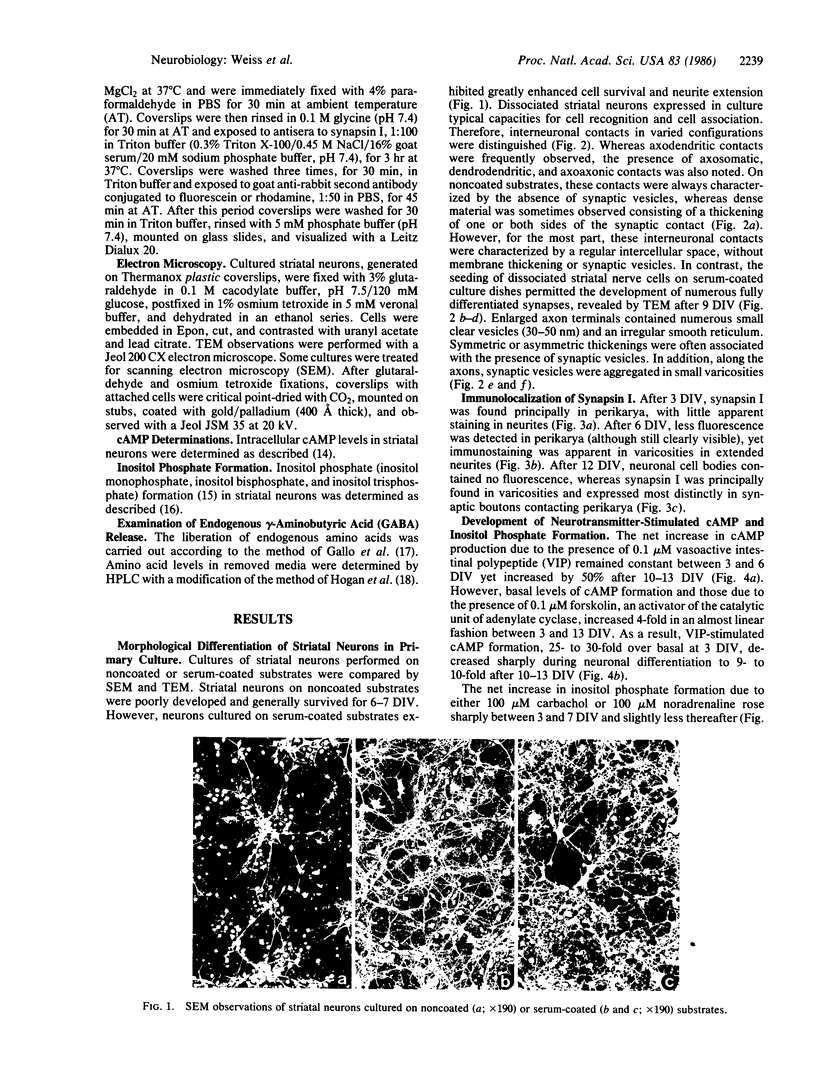
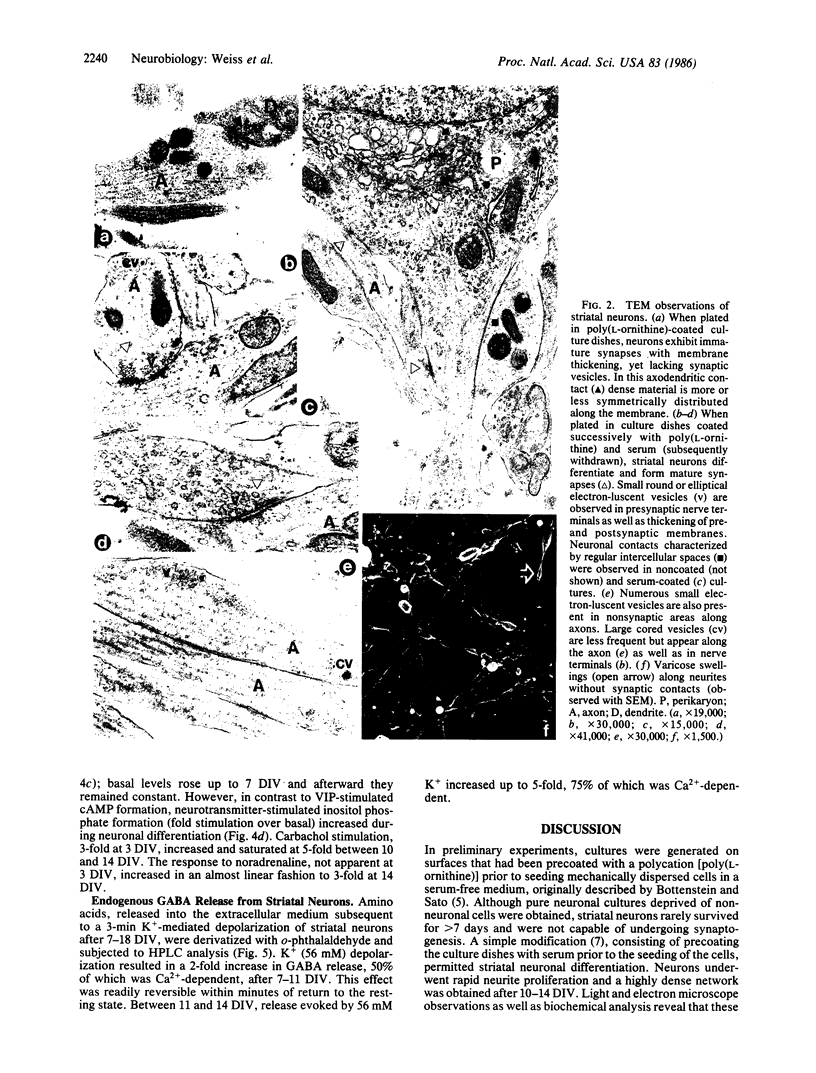
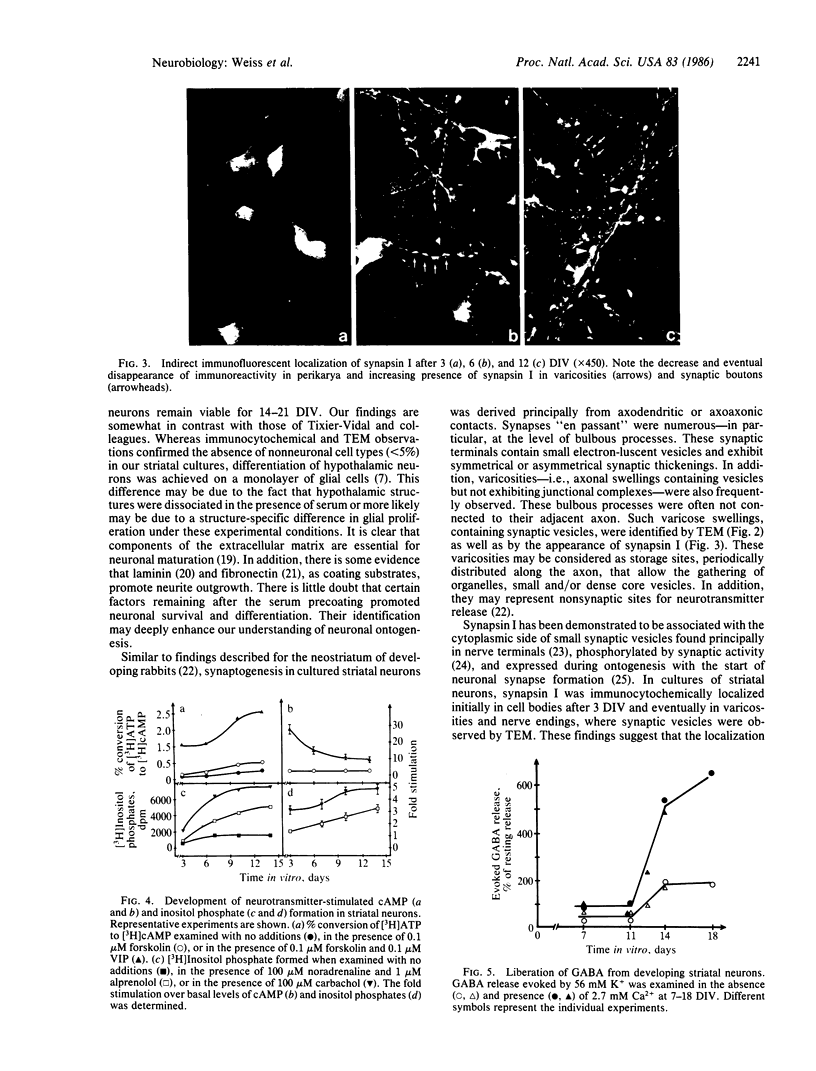
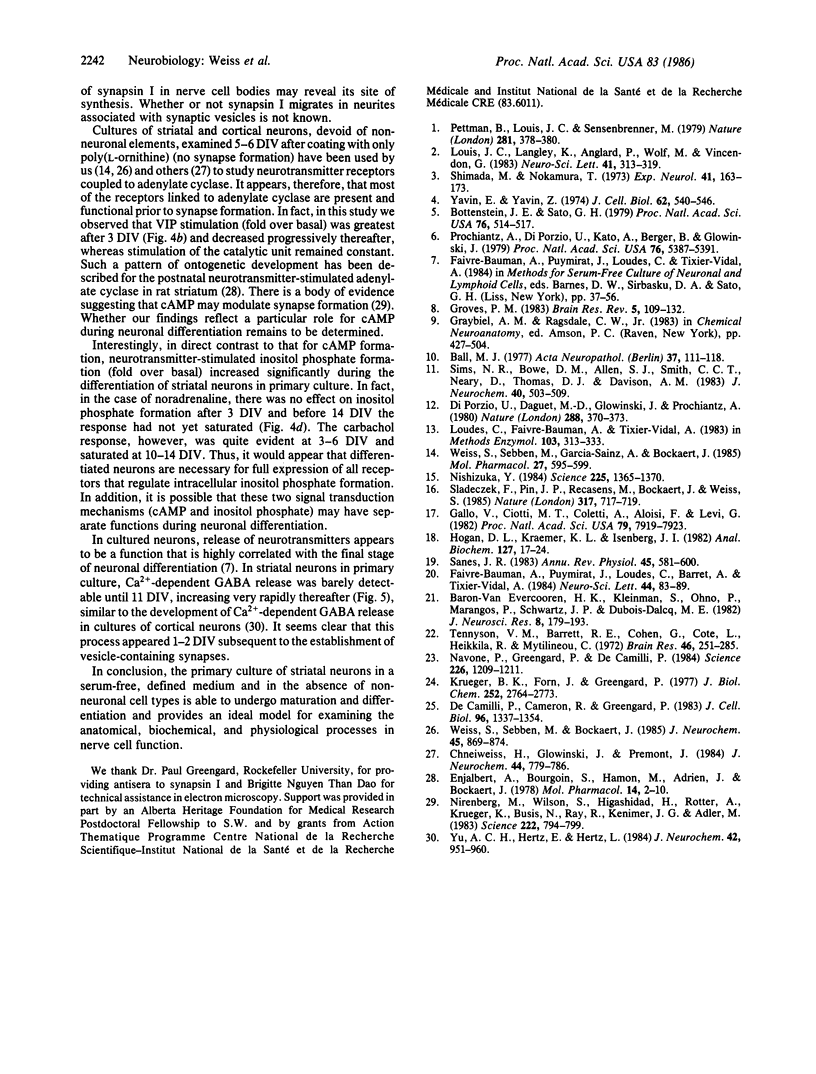
Images in this article
Selected References
These references are in PubMed. This may not be the complete list of references from this article.
- Ball M. J. Neuronal loss, neurofibrillary tangles and granulovacuolar degeneration in the hippocampus with ageing and dementia. A quantitative study. Acta Neuropathol. 1977 Feb 28;37(2):111–118. doi: 10.1007/BF00692056. [DOI] [PubMed] [Google Scholar]
- Baron-Van Evercooren A., Kleinman H. K., Ohno S., Marangos P., Schwartz J. P., Dubois-Dalcq M. E. Nerve growth factor, laminin, and fibronectin promote neurite growth in human fetal sensory ganglia cultures. J Neurosci Res. 1982;8(2-3):179–193. doi: 10.1002/jnr.490080208. [DOI] [PubMed] [Google Scholar]
- Bottenstein J. E., Sato G. H. Growth of a rat neuroblastoma cell line in serum-free supplemented medium. Proc Natl Acad Sci U S A. 1979 Jan;76(1):514–517. doi: 10.1073/pnas.76.1.514. [DOI] [PMC free article] [PubMed] [Google Scholar]
- Chneiweiss H., Glowinski J., Prémont J. Vasoactive intestinal polypeptide receptors linked to an adenylate cyclase, and their relationship with biogenic amine- and somatostatin-sensitive adenylate cyclases on central neuronal and glial cells in primary cultures. J Neurochem. 1985 Mar;44(3):779–786. doi: 10.1111/j.1471-4159.1985.tb12883.x. [DOI] [PubMed] [Google Scholar]
- De Camilli P., Cameron R., Greengard P. Synapsin I (protein I), a nerve terminal-specific phosphoprotein. I. Its general distribution in synapses of the central and peripheral nervous system demonstrated by immunofluorescence in frozen and plastic sections. J Cell Biol. 1983 May;96(5):1337–1354. doi: 10.1083/jcb.96.5.1337. [DOI] [PMC free article] [PubMed] [Google Scholar]
- Enjalbert A., Bourgoin S., Hamon M., Adrien J., Bockaert J. Postsynaptic serotonin-sensitive adenylate cyclase in the central nervous system. I. Development and distribution of serotonin and dopamine-sensitive adenylate cyclases in rat and guinea pig brain. Mol Pharmacol. 1978 Jan;14(1):2–10. [PubMed] [Google Scholar]
- Faivre-Bauman A., Puymirat J., Loudes C., Barret A., Tixier-Vidal A. Laminin promotes attachment and neurite elongation of fetal hypothalamic neurons grown in serum-free medium. Neurosci Lett. 1984 Jan 27;44(1):83–89. doi: 10.1016/0304-3940(84)90225-8. [DOI] [PubMed] [Google Scholar]
- Gallo V., Ciotti M. T., Coletti A., Aloisi F., Levi G. Selective release of glutamate from cerebellar granule cells differentiating in culture. Proc Natl Acad Sci U S A. 1982 Dec;79(24):7919–7923. doi: 10.1073/pnas.79.24.7919. [DOI] [PMC free article] [PubMed] [Google Scholar]
- Groves P. M. A theory of the functional organization of the neostriatum and the neostriatal control of voluntary movement. Brain Res. 1983 Mar;286(2):109–132. doi: 10.1016/0165-0173(83)90011-5. [DOI] [PubMed] [Google Scholar]
- Hogan D. L., Kraemer K. L., Isenberg J. I. The use of high-performance liquid chromatography for quantitation of plasma amino acids in man. Anal Biochem. 1982 Nov 15;127(1):17–24. doi: 10.1016/0003-2697(82)90138-5. [DOI] [PubMed] [Google Scholar]
- Krueger B. K., Forn J., Greengard P. Depolarization-induced phosphorylation of specific proteins, mediated by calcium ion influx, in rat brain synaptosomes. J Biol Chem. 1977 Apr 25;252(8):2764–2773. [PubMed] [Google Scholar]
- Loudes C., Faivre-Bauman A., Tixier-Vidal A. Techniques for culture of hypothalamic neurons. Methods Enzymol. 1983;103:313–334. doi: 10.1016/s0076-6879(83)03021-9. [DOI] [PubMed] [Google Scholar]
- Louis J. C., Langley K., Anglard P., Wolf M., Vincendon G. Long-term culture of neurones from human cerebral cortex in serum-free medium. Neurosci Lett. 1983 Nov 11;41(3):313–319. doi: 10.1016/0304-3940(83)90469-x. [DOI] [PubMed] [Google Scholar]
- Navone F., Greengard P., De Camilli P. Synapsin I in nerve terminals: selective association with small synaptic vesicles. Science. 1984 Dec 7;226(4679):1209–1211. doi: 10.1126/science.6438799. [DOI] [PubMed] [Google Scholar]
- Nirenberg M., Wilson S., Higashida H., Rotter A., Krueger K., Busis N., Ray R., Kenimer J. G., Adler M. Modulation of synapse formation by cyclic adenosine monophosphate. Science. 1983 Nov 18;222(4625):794–799. doi: 10.1126/science.6314503. [DOI] [PubMed] [Google Scholar]
- Nishizuka Y. Turnover of inositol phospholipids and signal transduction. Science. 1984 Sep 21;225(4668):1365–1370. doi: 10.1126/science.6147898. [DOI] [PubMed] [Google Scholar]
- Pettmann B., Louis J. C., Sensenbrenner M. Morphological and biochemical maturation of neurones cultured in the absence of glial cells. Nature. 1979 Oct 4;281(5730):378–380. doi: 10.1038/281378a0. [DOI] [PubMed] [Google Scholar]
- Prochiantz A., di Porzio U., Kato A., Berger B., Glowinski J. In vitro maturation of mesencephalic dopaminergic neurons from mouse embryos is enhanced in presence of their striatal target cells. Proc Natl Acad Sci U S A. 1979 Oct;76(10):5387–5391. doi: 10.1073/pnas.76.10.5387. [DOI] [PMC free article] [PubMed] [Google Scholar]
- Sanes J. R. Roles of extracellular matrix in neural development. Annu Rev Physiol. 1983;45:581–600. doi: 10.1146/annurev.ph.45.030183.003053. [DOI] [PubMed] [Google Scholar]
- Shimada M., Nakamura T. Time of neuron origin in mouse hypothalamic nuclei. Exp Neurol. 1973 Oct;41(1):163–173. doi: 10.1016/0014-4886(73)90187-8. [DOI] [PubMed] [Google Scholar]
- Sims N. R., Bowen D. M., Allen S. J., Smith C. C., Neary D., Thomas D. J., Davison A. N. Presynaptic cholinergic dysfunction in patients with dementia. J Neurochem. 1983 Feb;40(2):503–509. doi: 10.1111/j.1471-4159.1983.tb11311.x. [DOI] [PubMed] [Google Scholar]
- Sladeczek F., Pin J. P., Récasens M., Bockaert J., Weiss S. Glutamate stimulates inositol phosphate formation in striatal neurones. Nature. 1985 Oct 24;317(6039):717–719. doi: 10.1038/317717a0. [DOI] [PubMed] [Google Scholar]
- Tennyson V. M., Barrett R. E., Cohen G., Côté L., Heikkila R., Mytilineou C. The developing neostriatum of the rabbit: correlation of fluorescence histochemistry, electron microscopy, endogenous dopamine levels, and ( 3 H)dopamine uptake. Brain Res. 1972 Nov 13;46:251–285. doi: 10.1016/0006-8993(72)90019-4. [DOI] [PubMed] [Google Scholar]
- Weiss S., Sebben M., Bockaert J. Corticotropin-peptide regulation of intracellular cyclic AMP production in cortical neurons in primary culture. J Neurochem. 1985 Sep;45(3):869–874. doi: 10.1111/j.1471-4159.1985.tb04074.x. [DOI] [PubMed] [Google Scholar]
- Weiss S., Sebben M., Garcia-Sainz J. A., Bockaert J. D2-dopamine receptor-mediated inhibition of cyclic AMP formation in striatal neurons in primary culture. Mol Pharmacol. 1985 Jun;27(6):595–599. [PubMed] [Google Scholar]
- Yavin E., Yavin Z. Attachment and culture of dissociated cells from rat embryo cerebral hemispheres on polylysine-coated surface. J Cell Biol. 1974 Aug;62(2):540–546. doi: 10.1083/jcb.62.2.540. [DOI] [PMC free article] [PubMed] [Google Scholar]
- Yu A. C., Hertz E., Hertz L. Alterations in uptake and release rates for GABA, glutamate, and glutamine during biochemical maturation of highly purified cultures of cerebral cortical neurons, a GABAergic preparation. J Neurochem. 1984 Apr;42(4):951–960. doi: 10.1111/j.1471-4159.1984.tb12696.x. [DOI] [PubMed] [Google Scholar]
- di Porzio U., Daguet M. C., Glowinski J., Prochiantz A. Effect of striatal cells on in vitro maturation of mesencephalic dopaminergic neurones grown in serum-free conditions. Nature. 1980 Nov 27;288(5789):370–373. doi: 10.1038/288370a0. [DOI] [PubMed] [Google Scholar]