Abstract
We tested the role of different intracellular proteolytic pathways in sepsis-induced muscle proteolysis. Sepsis was induced in rats by cecal ligation and puncture; controls were sham operated. Total and myofibrillar proteolysis was determined in incubated extensor digitorum longus muscles as release of tyrosine and 3-methylhistidine, respectively. Lysosomal proteolysis was assessed by using the lysosomotropic agents NH4Cl, chloroquine, leupeptin, and methylamine. Ca(2+)-dependent proteolysis was determined in the absence or presence of Ca2+ or by blocking the Ca(2+)-dependent proteases calpain I and II. Energy-dependent proteolysis was determined in muscles depleted of ATP by 2-deoxyglucose and 2.4-dinitrophenol. Muscle ubiquitin mRNA and the concentrations of free and conjugated ubiquitin were determined by Northern and Western blots, respectively, to assess the role of the ATP-ubiquitin-dependent proteolytic pathway. Total and myofibrillar protein breakdown was increased during sepsis by 50 and 440%, respectively. Lysosomal and Ca(2+)-dependent proteolysis was similar in control and septic rats. In contrast, energy-dependent total and myofibrillar protein breakdown was increased by 172% and more than fourfold, respectively, in septic muscle. Ubiquitin mRNA was increased severalfold in septic muscle. The results suggest that the increase in muscle proteolysis during sepsis is due to an increase in nonlysosomal energy-dependent protein breakdown, which may involve the ubiquitin system.
Full text
PDF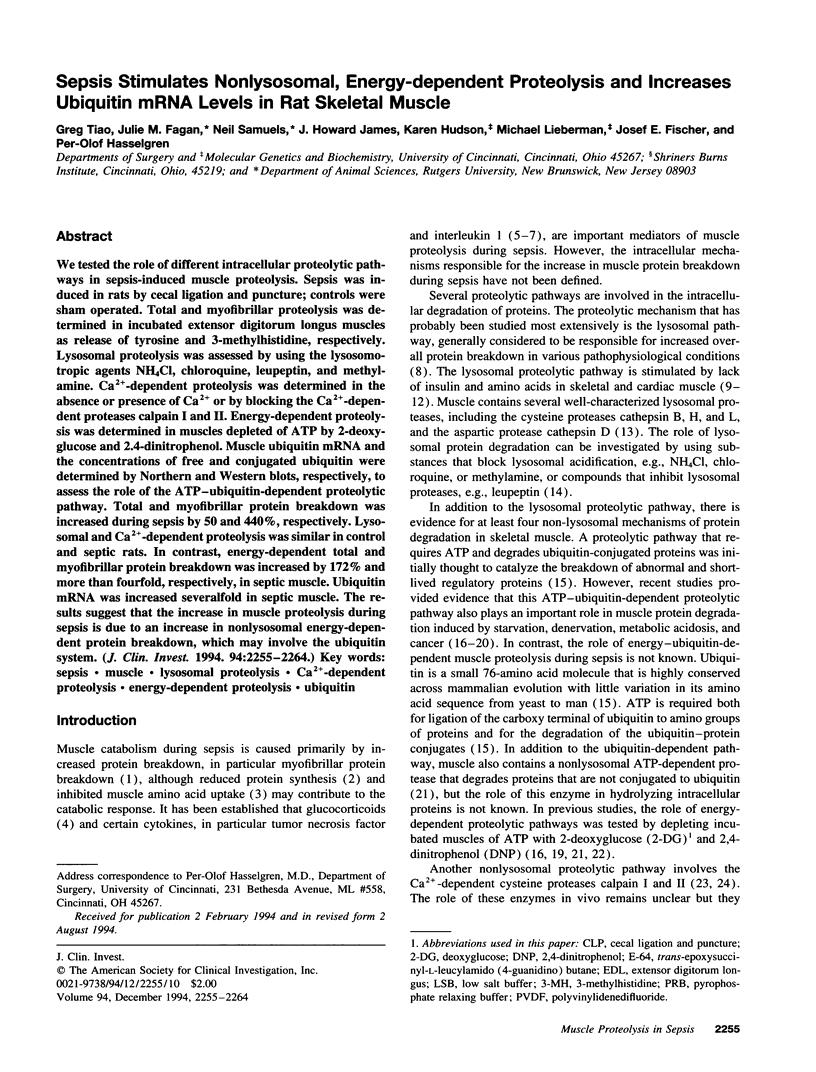
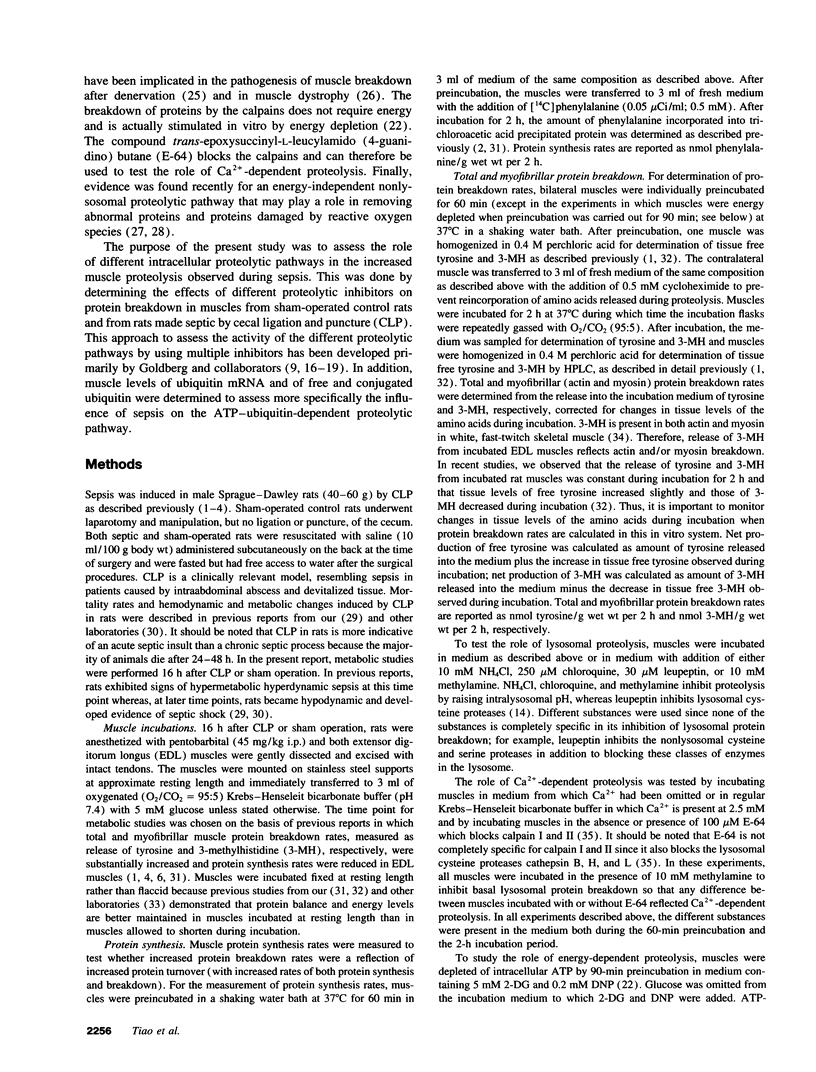
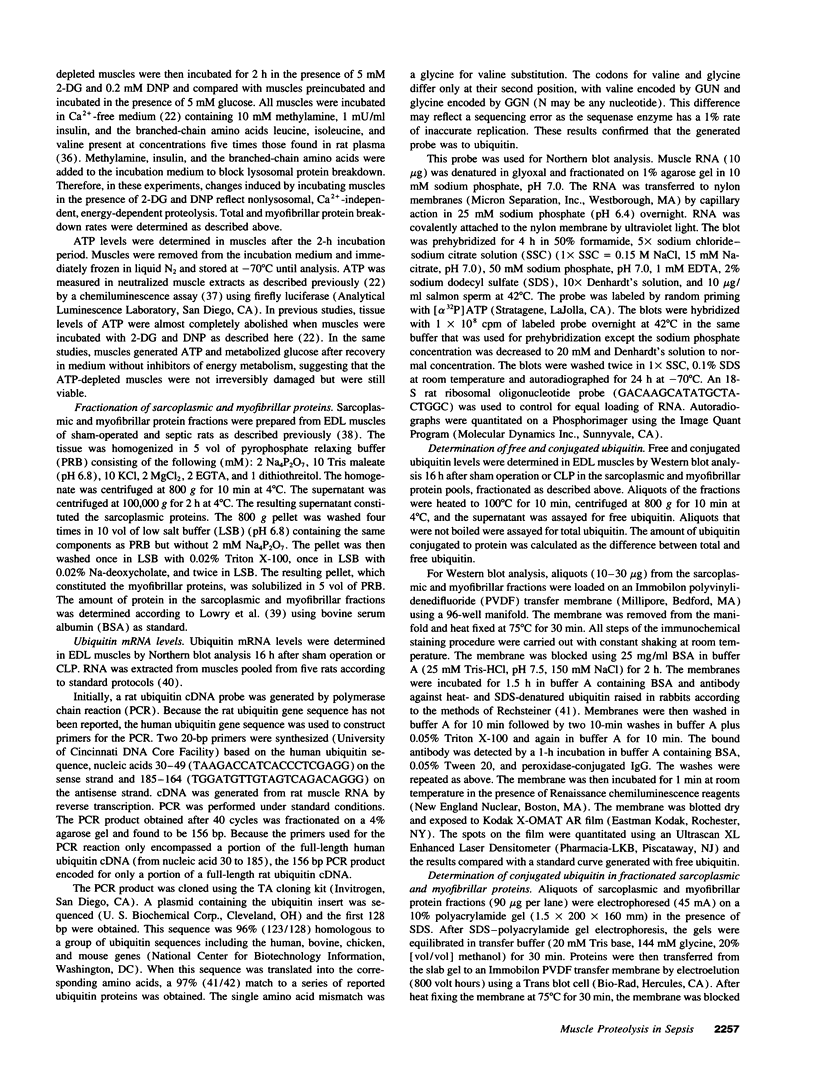
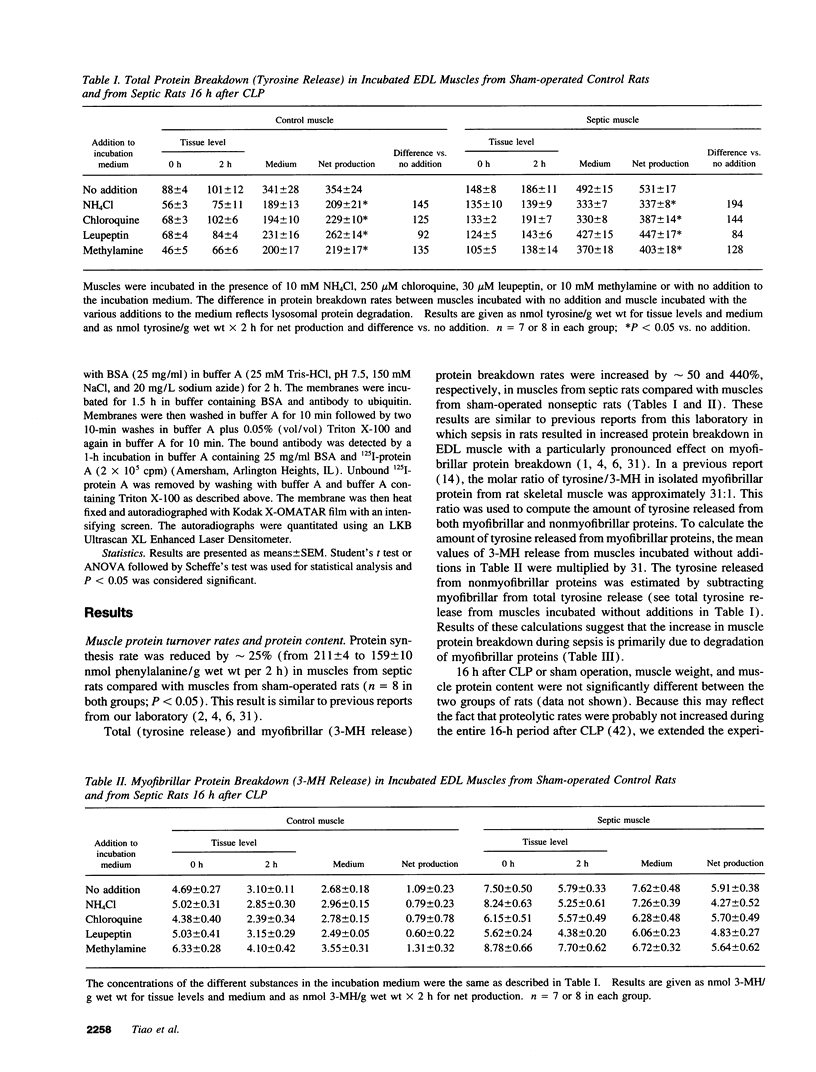
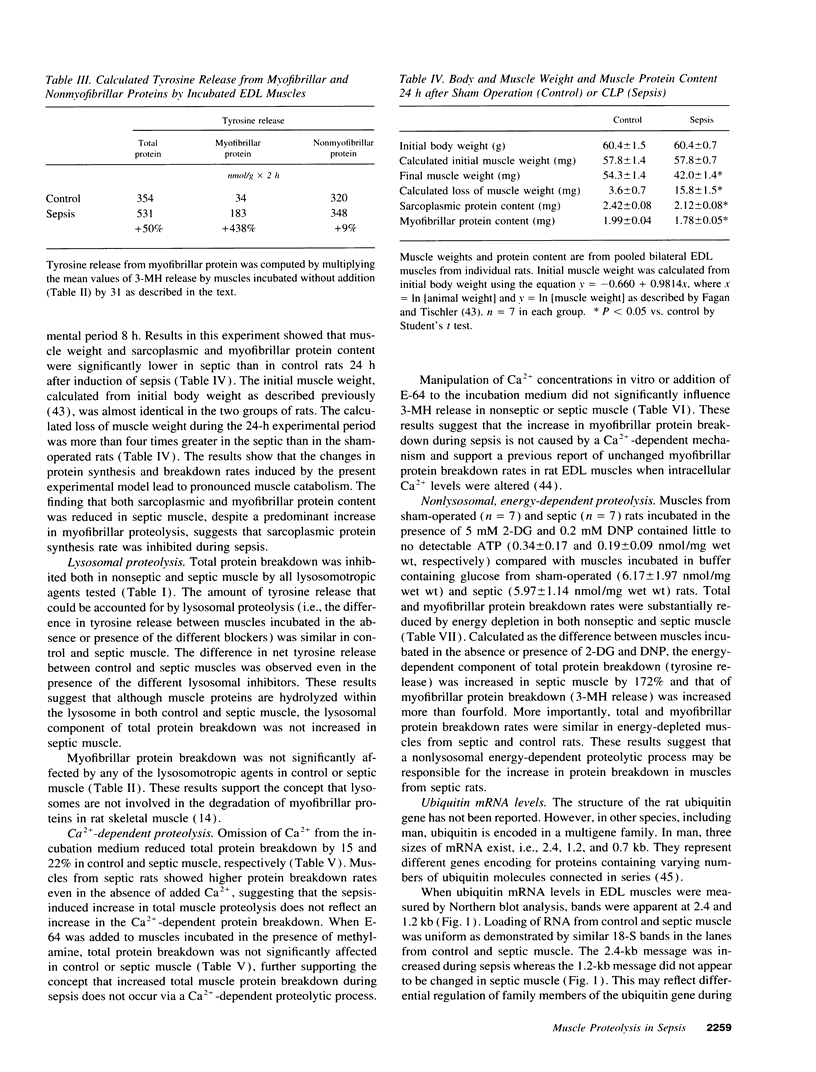
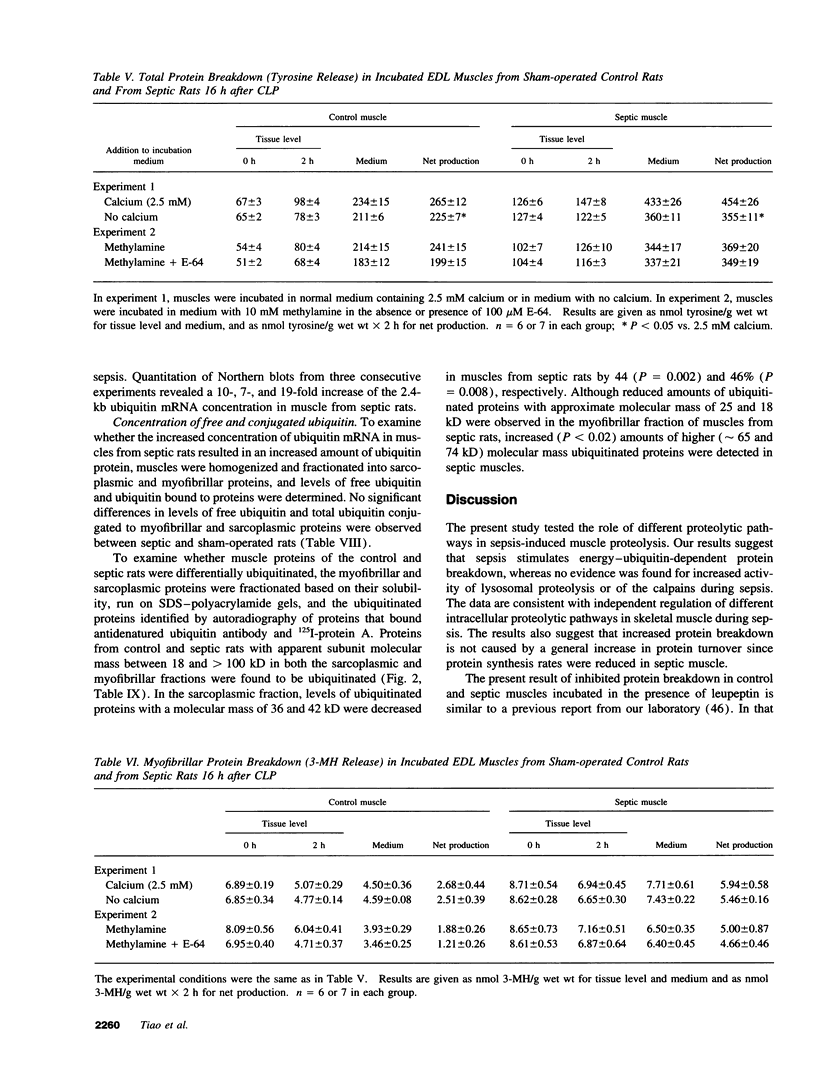
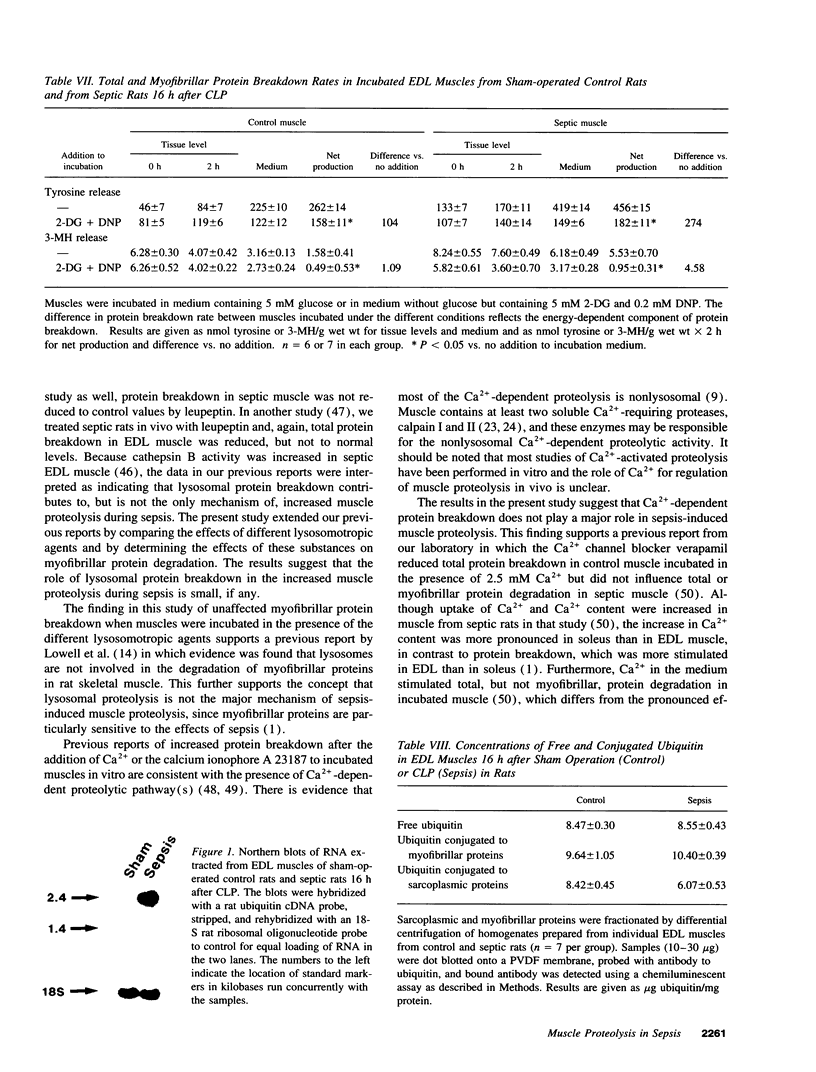
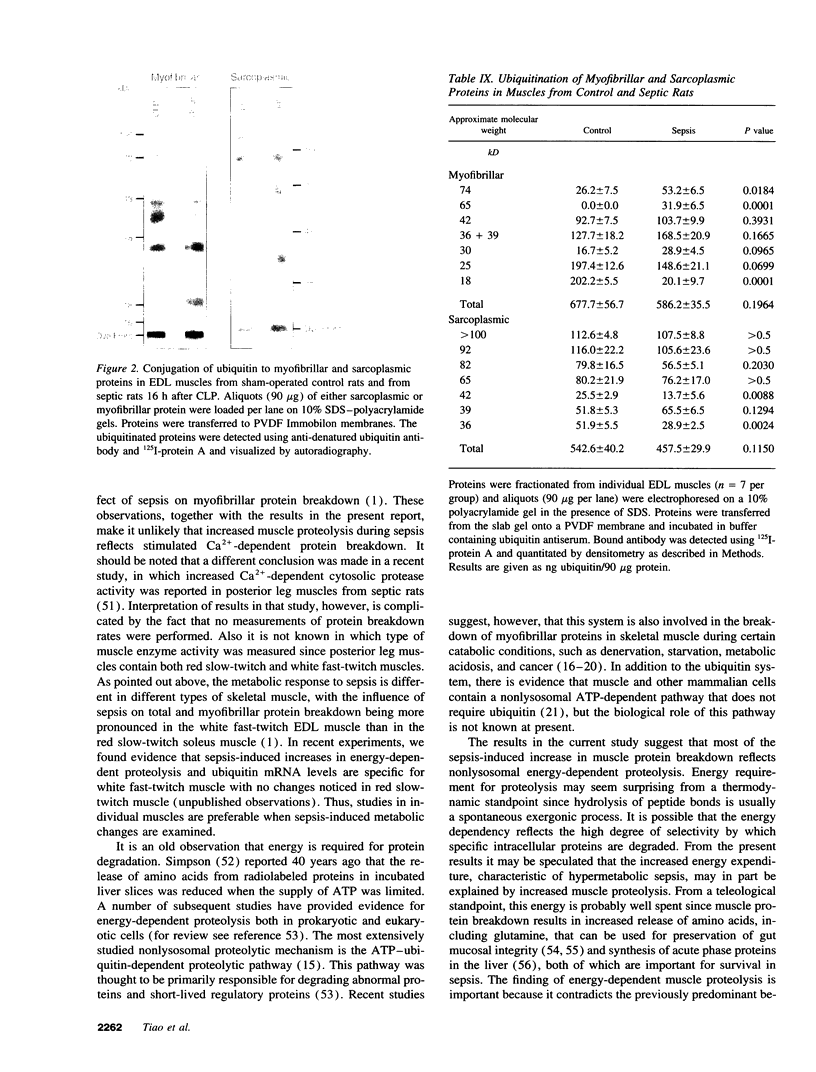
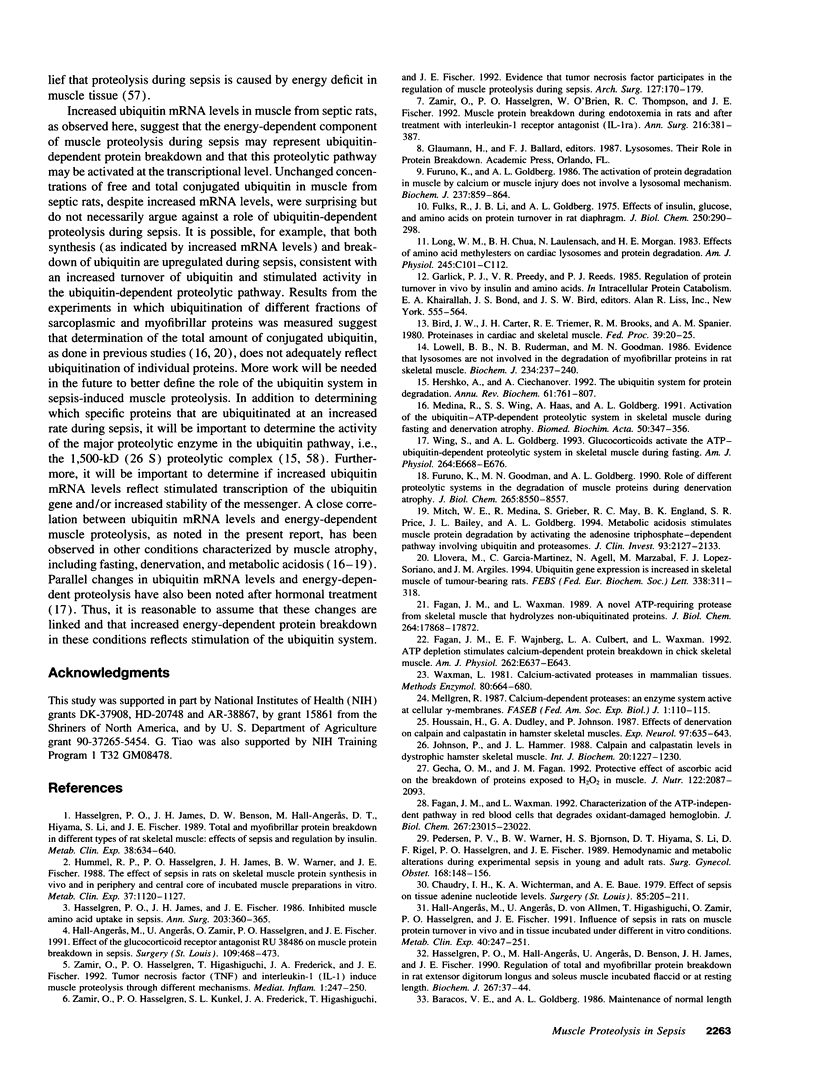
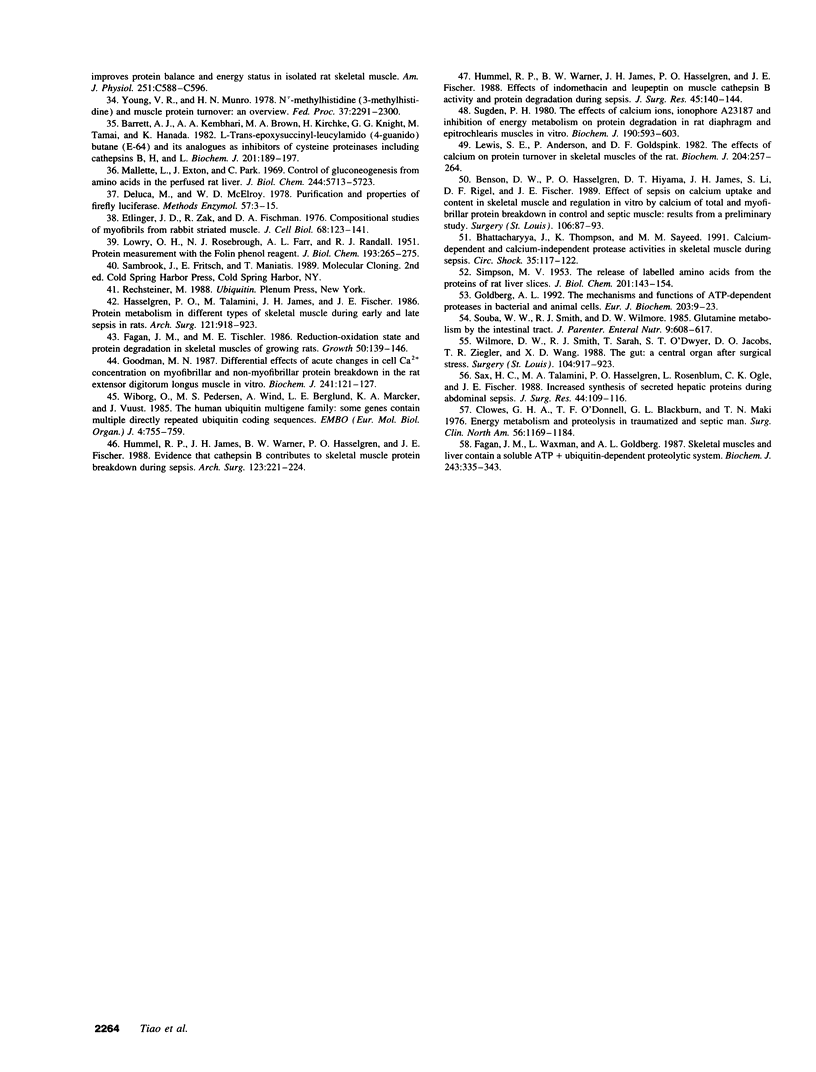
Images in this article
Selected References
These references are in PubMed. This may not be the complete list of references from this article.
- Baracos V. E., Goldberg A. L. Maintenance of normal length improves protein balance and energy status in isolated rat skeletal muscles. Am J Physiol. 1986 Oct;251(4 Pt 1):C588–C596. doi: 10.1152/ajpcell.1986.251.4.C588. [DOI] [PubMed] [Google Scholar]
- Barrett A. J., Kembhavi A. A., Brown M. A., Kirschke H., Knight C. G., Tamai M., Hanada K. L-trans-Epoxysuccinyl-leucylamido(4-guanidino)butane (E-64) and its analogues as inhibitors of cysteine proteinases including cathepsins B, H and L. Biochem J. 1982 Jan 1;201(1):189–198. doi: 10.1042/bj2010189. [DOI] [PMC free article] [PubMed] [Google Scholar]
- Benson D. W., Hasselgren P. O., Hiyama D. T., James J. H., Li S., Rigel D. F., Fischer J. E. Effect of sepsis on calcium uptake and content in skeletal muscle and regulation in vitro by calcium of total and myofibrillar protein breakdown in control and septic muscle: results from a preliminary study. Surgery. 1989 Jul;106(1):87–93. [PubMed] [Google Scholar]
- Bhattacharyya J., Thompson K., Sayeed M. M. Calcium-dependent and calcium-independent protease activities in skeletal muscle during sepsis. Circ Shock. 1991 Oct;35(2):117–122. [PubMed] [Google Scholar]
- Bird J. W., Carter J. H., Triemer R. E., Brooks R. M., Spanier A. M. Proteinases in cardiac and skeletal muscle. Fed Proc. 1980 Jan;39(1):20–25. [PubMed] [Google Scholar]
- Chaudry I. H., Wichterman K. A., Baue A. E. Effect of sepsis on tissue adenine nucleotide levels. Surgery. 1979 Feb;85(2):205–211. [PubMed] [Google Scholar]
- Clowes G. H., Jr, O'Donnell T. F., Blackburn G. L., Maki T. N. Energy metabolism and proteolysis in traumatized and septic man. Surg Clin North Am. 1976 Oct;56(5):1169–1184. doi: 10.1016/s0039-6109(16)41036-4. [DOI] [PubMed] [Google Scholar]
- Etlinger J. D., Zak R., Fischman D. A. Compositional studies of myofibrils from rabbit striated muscle. J Cell Biol. 1976 Jan;68(1):123–141. doi: 10.1083/jcb.68.1.123. [DOI] [PMC free article] [PubMed] [Google Scholar]
- Fagan J. M., Tischler M. E. Reduction-oxidation state and protein degradation in skeletal muscles of growing rats. Growth. 1986 Summer;50(2):139–146. [PubMed] [Google Scholar]
- Fagan J. M., Wajnberg E. F., Culbert L., Waxman L. ATP depletion stimulates calcium-dependent protein breakdown in chick skeletal muscle. Am J Physiol. 1992 May;262(5 Pt 1):E637–E643. doi: 10.1152/ajpendo.1992.262.5.E637. [DOI] [PubMed] [Google Scholar]
- Fagan J. M., Waxman L. A novel ATP-requiring protease from skeletal muscle that hydrolyzes non-ubiquitinated proteins. J Biol Chem. 1989 Oct 25;264(30):17868–17872. [PubMed] [Google Scholar]
- Fagan J. M., Waxman L., Goldberg A. L. Skeletal muscle and liver contain a soluble ATP + ubiquitin-dependent proteolytic system. Biochem J. 1987 Apr 15;243(2):335–343. doi: 10.1042/bj2430335. [DOI] [PMC free article] [PubMed] [Google Scholar]
- Fagan J. M., Waxman L. The ATP-independent pathway in red blood cells that degrades oxidant-damaged hemoglobin. J Biol Chem. 1992 Nov 15;267(32):23015–23022. [PubMed] [Google Scholar]
- Fulks R. M., Li J. B., Goldberg A. L. Effects of insulin, glucose, and amino acids on protein turnover in rat diaphragm. J Biol Chem. 1975 Jan 10;250(1):290–298. [PubMed] [Google Scholar]
- Furuno K., Goldberg A. L. The activation of protein degradation in muscle by Ca2+ or muscle injury does not involve a lysosomal mechanism. Biochem J. 1986 Aug 1;237(3):859–864. doi: 10.1042/bj2370859. [DOI] [PMC free article] [PubMed] [Google Scholar]
- Furuno K., Goodman M. N., Goldberg A. L. Role of different proteolytic systems in the degradation of muscle proteins during denervation atrophy. J Biol Chem. 1990 May 25;265(15):8550–8557. [PubMed] [Google Scholar]
- Garlick P. J., Preedy V. R., Reeds P. J. Regulation of protein turnover in vivo by insulin and amino acids. Prog Clin Biol Res. 1985;180:555–564. [PubMed] [Google Scholar]
- Gecha O. M., Fagan J. M. Protective effect of ascorbic acid on the breakdown of proteins exposed to hydrogen peroxide in chicken skeletal muscle. J Nutr. 1992 Nov;122(11):2087–2093. doi: 10.1093/jn/122.11.2087. [DOI] [PubMed] [Google Scholar]
- Goldberg A. L. The mechanism and functions of ATP-dependent proteases in bacterial and animal cells. Eur J Biochem. 1992 Jan 15;203(1-2):9–23. doi: 10.1111/j.1432-1033.1992.tb19822.x. [DOI] [PubMed] [Google Scholar]
- Goodman M. N. Differential effects of acute changes in cell Ca2+ concentration on myofibrillar and non-myofibrillar protein breakdown in the rat extensor digitorum longus muscle in vitro. Assessment by production of tyrosine and N tau-methylhistidine. Biochem J. 1987 Jan 1;241(1):121–127. doi: 10.1042/bj2410121. [DOI] [PMC free article] [PubMed] [Google Scholar]
- Hall-Angerås M., Angerås U., Zamir O., Hasselgren P. O., Fischer J. E. Effect of the glucocorticoid receptor antagonist RU 38486 on muscle protein breakdown in sepsis. Surgery. 1991 Apr;109(4):468–473. [PubMed] [Google Scholar]
- Hall-Angerås M., Angerås U., von Allmen D., Higashiguchi T., Zamir O., Hasselgren P. O., Fischer J. E. Influence of sepsis in rats on muscle protein turnover in vivo and in tissue incubated under different in vitro conditions. Metabolism. 1991 Mar;40(3):247–251. doi: 10.1016/0026-0495(91)90105-6. [DOI] [PubMed] [Google Scholar]
- Hasselgren P. O., Hall-Angerås M., Angerås U., Benson D., James J. H., Fischer J. E. Regulation of total and myofibrillar protein breakdown in rat extensor digitorum longus and soleus muscle incubated flaccid or at resting length. Biochem J. 1990 Apr 1;267(1):37–44. doi: 10.1042/bj2670037. [DOI] [PMC free article] [PubMed] [Google Scholar]
- Hasselgren P. O., James J. H., Benson D. W., Hall-Angerås M., Angerås U., Hiyama D. T., Li S., Fischer J. E. Total and myofibrillar protein breakdown in different types of rat skeletal muscle: effects of sepsis and regulation by insulin. Metabolism. 1989 Jul;38(7):634–640. doi: 10.1016/0026-0495(89)90100-5. [DOI] [PubMed] [Google Scholar]
- Hasselgren P. O., James J. H., Fischer J. E. Inhibited muscle amino acid uptake in sepsis. Ann Surg. 1986 Apr;203(4):360–365. doi: 10.1097/00000658-198604000-00004. [DOI] [PMC free article] [PubMed] [Google Scholar]
- Hasselgren P. O., Talamini M., James J. H., Fischer J. E. Protein metabolism in different types of skeletal muscle during early and late sepsis in rats. Arch Surg. 1986 Aug;121(8):918–923. doi: 10.1001/archsurg.1986.01400080064011. [DOI] [PubMed] [Google Scholar]
- Hershko A., Ciechanover A. The ubiquitin system for protein degradation. Annu Rev Biochem. 1992;61:761–807. doi: 10.1146/annurev.bi.61.070192.003553. [DOI] [PubMed] [Google Scholar]
- Hummel R. P., 3rd, Hasselgren P. O., James J. H., Warner B. W., Fischer J. E. The effect of sepsis in rats on skeletal muscle protein synthesis in vivo and in periphery and central core of incubated muscle preparations in vitro. Metabolism. 1988 Dec;37(12):1120–1127. doi: 10.1016/0026-0495(88)90187-4. [DOI] [PubMed] [Google Scholar]
- Hummel R. P., 3rd, James J. H., Warner B. W., Hasselgren P. O., Fischer J. E. Evidence that cathepsin B contributes to skeletal muscle protein breakdown during sepsis. Arch Surg. 1988 Feb;123(2):221–224. doi: 10.1001/archsurg.1988.01400260105013. [DOI] [PubMed] [Google Scholar]
- Hummel R. P., 3rd, Warner B. W., James J. H., Hasselgren P. O., Fischer J. E. Effects of indomethacin and leupeptin on muscle cathepsin B activity and protein degradation during sepsis. J Surg Res. 1988 Jul;45(1):140–144. doi: 10.1016/0022-4804(88)90033-9. [DOI] [PubMed] [Google Scholar]
- Hussain H., Dudley G. A., Johnson P. Effects of denervation on calpain and calpastatin in hamster skeletal muscles. Exp Neurol. 1987 Sep;97(3):635–643. doi: 10.1016/0014-4886(87)90120-8. [DOI] [PubMed] [Google Scholar]
- Johnson P., Hammer J. L. Calpain and calpastatin levels in dystrophic hamster skeletal muscles. Int J Biochem. 1988;20(11):1227–1230. doi: 10.1016/0020-711x(88)90223-6. [DOI] [PubMed] [Google Scholar]
- LOWRY O. H., ROSEBROUGH N. J., FARR A. L., RANDALL R. J. Protein measurement with the Folin phenol reagent. J Biol Chem. 1951 Nov;193(1):265–275. [PubMed] [Google Scholar]
- Lewis S. E., Anderson P., Goldspink D. F. The effects of calcium on protein turnover in skeletal muscles of the rat. Biochem J. 1982 Apr 15;204(1):257–264. doi: 10.1042/bj2040257. [DOI] [PMC free article] [PubMed] [Google Scholar]
- Llovera M., García-Martínez C., Agell N., Marzábal M., López-Soriano F. J., Argilés J. M. Ubiquitin gene expression is increased in skeletal muscle of tumour-bearing rats. FEBS Lett. 1994 Feb 7;338(3):311–318. doi: 10.1016/0014-5793(94)80290-4. [DOI] [PubMed] [Google Scholar]
- Long W. M., Chua B. H., Lautensack N., Morgan H. E. Effects of amino acid methyl esters on cardiac lysosomes and protein degradation. Am J Physiol. 1983 Jul;245(1):C101–C112. doi: 10.1152/ajpcell.1983.245.1.C101. [DOI] [PubMed] [Google Scholar]
- Lowell B. B., Ruderman N. B., Goodman M. N. Evidence that lysosomes are not involved in the degradation of myofibrillar proteins in rat skeletal muscle. Biochem J. 1986 Feb 15;234(1):237–240. doi: 10.1042/bj2340237. [DOI] [PMC free article] [PubMed] [Google Scholar]
- Mallet L. E., Exton J. H., Park C. R. Control of gluconeogenesis from amino acids in the perfused rat liver. J Biol Chem. 1969 Oct 25;244(20):5713–5723. [PubMed] [Google Scholar]
- Medina R., Wing S. S., Haas A., Goldberg A. L. Activation of the ubiquitin-ATP-dependent proteolytic system in skeletal muscle during fasting and denervation atrophy. Biomed Biochim Acta. 1991;50(4-6):347–356. [PubMed] [Google Scholar]
- Mellgren R. L. Calcium-dependent proteases: an enzyme system active at cellular membranes? FASEB J. 1987 Aug;1(2):110–115. doi: 10.1096/fasebj.1.2.2886390. [DOI] [PubMed] [Google Scholar]
- Mitch W. E., Medina R., Grieber S., May R. C., England B. K., Price S. R., Bailey J. L., Goldberg A. L. Metabolic acidosis stimulates muscle protein degradation by activating the adenosine triphosphate-dependent pathway involving ubiquitin and proteasomes. J Clin Invest. 1994 May;93(5):2127–2133. doi: 10.1172/JCI117208. [DOI] [PMC free article] [PubMed] [Google Scholar]
- Pedersen P. V., Warner B. W., Bjornson H. S., Hiyama D. T., Li S., Rigel D. F., Hasselgren P. O., Fischer J. E. Hemodynamic and metabolic alterations during experimental sepsis in young and adult rats. Surg Gynecol Obstet. 1989 Feb;168(2):148–156. [PubMed] [Google Scholar]
- SIMPSON M. V. The release of labeled amino acids from the proteins of rat liver slices. J Biol Chem. 1953 Mar;201(1):143–154. [PubMed] [Google Scholar]
- Sax H. C., Talamini M. A., Hasselgren P. O., Rosenblum L., Ogle C. K., Fischer J. E. Increased synthesis of secreted hepatic proteins during abdominal sepsis. J Surg Res. 1988 Feb;44(2):109–116. doi: 10.1016/0022-4804(88)90038-8. [DOI] [PubMed] [Google Scholar]
- Souba W. W., Smith R. J., Wilmore D. W. Glutamine metabolism by the intestinal tract. JPEN J Parenter Enteral Nutr. 1985 Sep-Oct;9(5):608–617. doi: 10.1177/0148607185009005608. [DOI] [PubMed] [Google Scholar]
- Sugden P. H. The effects of calcium ions, ionophore A23187 and inhibition of energy metabolism on protein degradation in the rat diaphragm and epitrochlearis muscles in vitro. Biochem J. 1980 Sep 15;190(3):593–603. doi: 10.1042/bj1900593. [DOI] [PMC free article] [PubMed] [Google Scholar]
- Waxman L. Calcium-activated proteases in mammalian tissues. Methods Enzymol. 1981;80(Pt 100):664–680. doi: 10.1016/s0076-6879(81)80051-1. [DOI] [PubMed] [Google Scholar]
- Wiborg O., Pedersen M. S., Wind A., Berglund L. E., Marcker K. A., Vuust J. The human ubiquitin multigene family: some genes contain multiple directly repeated ubiquitin coding sequences. EMBO J. 1985 Mar;4(3):755–759. doi: 10.1002/j.1460-2075.1985.tb03693.x. [DOI] [PMC free article] [PubMed] [Google Scholar]
- Wilmore D. W., Smith R. J., O'Dwyer S. T., Jacobs D. O., Ziegler T. R., Wang X. D. The gut: a central organ after surgical stress. Surgery. 1988 Nov;104(5):917–923. [PubMed] [Google Scholar]
- Wing S. S., Goldberg A. L. Glucocorticoids activate the ATP-ubiquitin-dependent proteolytic system in skeletal muscle during fasting. Am J Physiol. 1993 Apr;264(4 Pt 1):E668–E676. doi: 10.1152/ajpendo.1993.264.4.E668. [DOI] [PubMed] [Google Scholar]
- Young V. R., Munro H. N. Ntau-methylhistidine (3-methylhistidine) and muscle protein turnover: an overview. Fed Proc. 1978 Jul;37(9):2291–2300. [PubMed] [Google Scholar]
- Zamir O., Hasselgren P. O., Kunkel S. L., Frederick J., Higashiguchi T., Fischer J. E. Evidence that tumor necrosis factor participates in the regulation of muscle proteolysis during sepsis. Arch Surg. 1992 Feb;127(2):170–174. doi: 10.1001/archsurg.1992.01420020052008. [DOI] [PubMed] [Google Scholar]
- Zamir O., Hasselgren P. O., O'Brien W., Thompson R. C., Fischer J. E. Muscle protein breakdown during endotoxemia in rats and after treatment with interleukin-1 receptor antagonist (IL-1ra). Ann Surg. 1992 Sep;216(3):381–387. doi: 10.1097/00000658-199209000-00018. [DOI] [PMC free article] [PubMed] [Google Scholar]