Abstract
The deletion of the highly conserved cytidine nucleotide at position 1054 in E. coli 16S rRNA has been characterized to confer an UGA stop codon specific suppression activity which suggested a functional participation of small subunit rRNA in translational termination. Based on this structure-function correlation we constructed the three point mutations at site 1054, changing the wild-type C residue to an A, G or U base. The mutations were expressed from a complete plasmid encoded rRNA operon (rrnB) using a conditional expression system with the lambda PL-promoter. All three altered 16S rRNA molecules were expressed and incorporated into 70S ribosomal particles. Structural analysis of the protein and 16S rRNA moieties of the mutant ribosomes showed no differences when compared to wild-type particles. The phenotypic analysis revealed that only the 1054G base change led to a significantly reduced generation time of transformed cells, which could be correlated with the inability of the mutant ribosomes to specifically stop at UGA stop codons in vivo. The response towards UAA and UAG termination codons was not altered. Furthermore, in vitro RF-2 termination factor binding experiments indicated that the association behaviour of mutant ribosomes was not changed, enforcing the view that the UGA stop codon suppression is a direct consequence of the rRNA mutation. Taken together, these results argue for a direct participation of that 16S rRNA motif in UGA dependent translational termination and furthermore, suggest that termination factor binding and stop codon recognition are two separate steps of the termination event.
Full text
PDF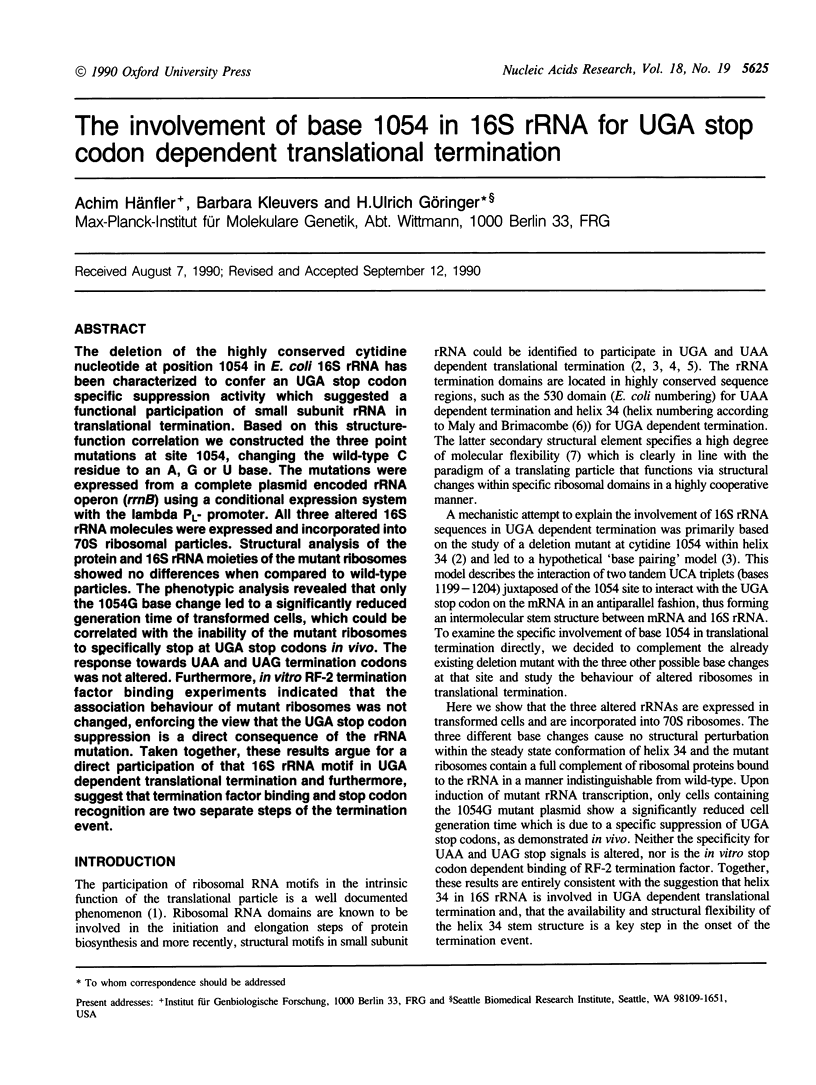
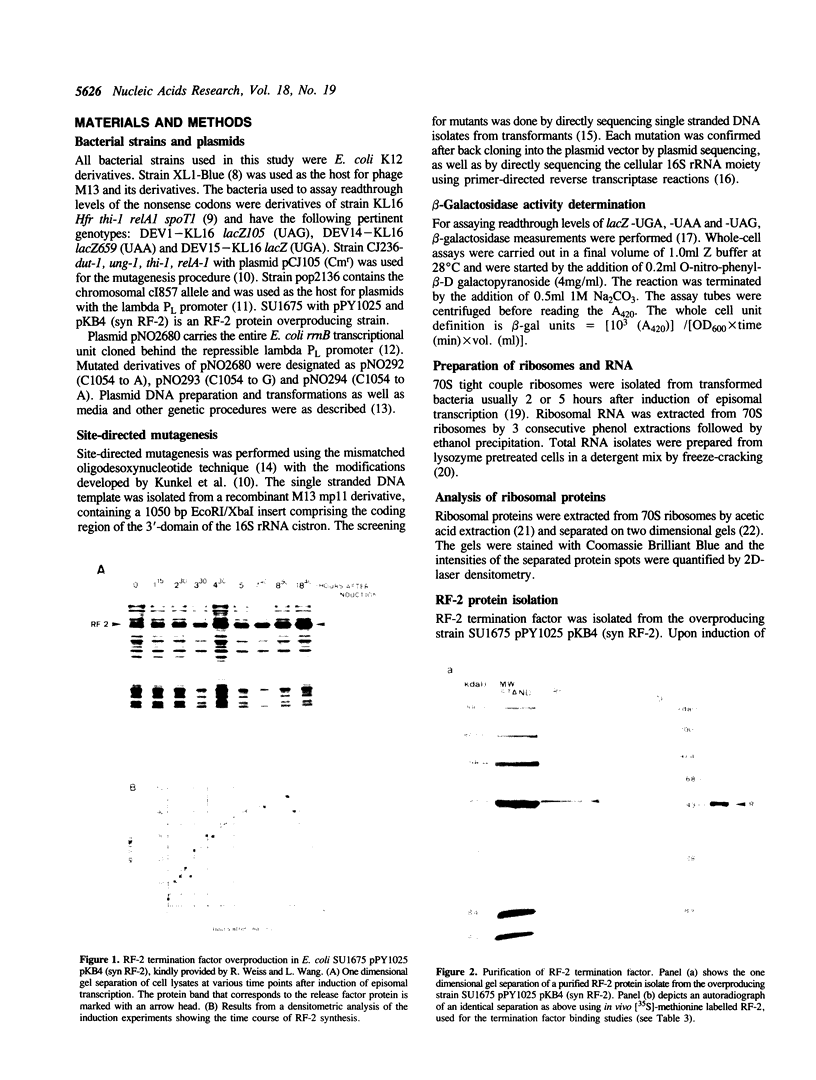
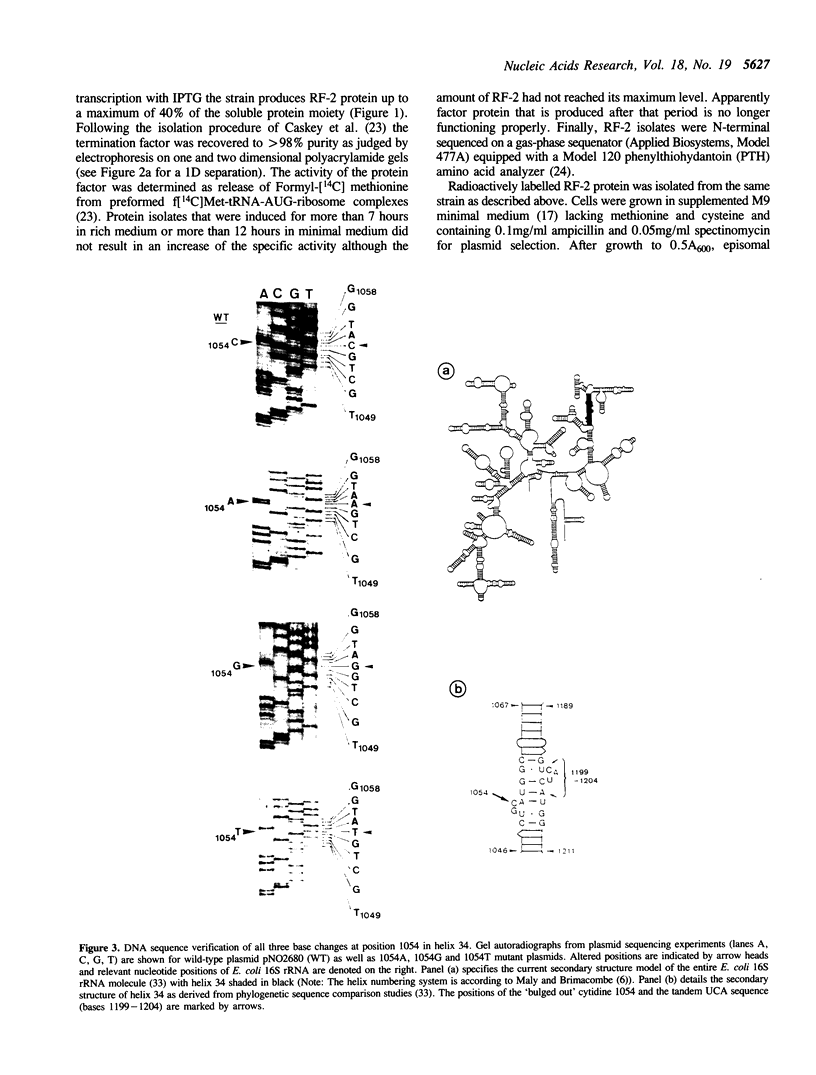
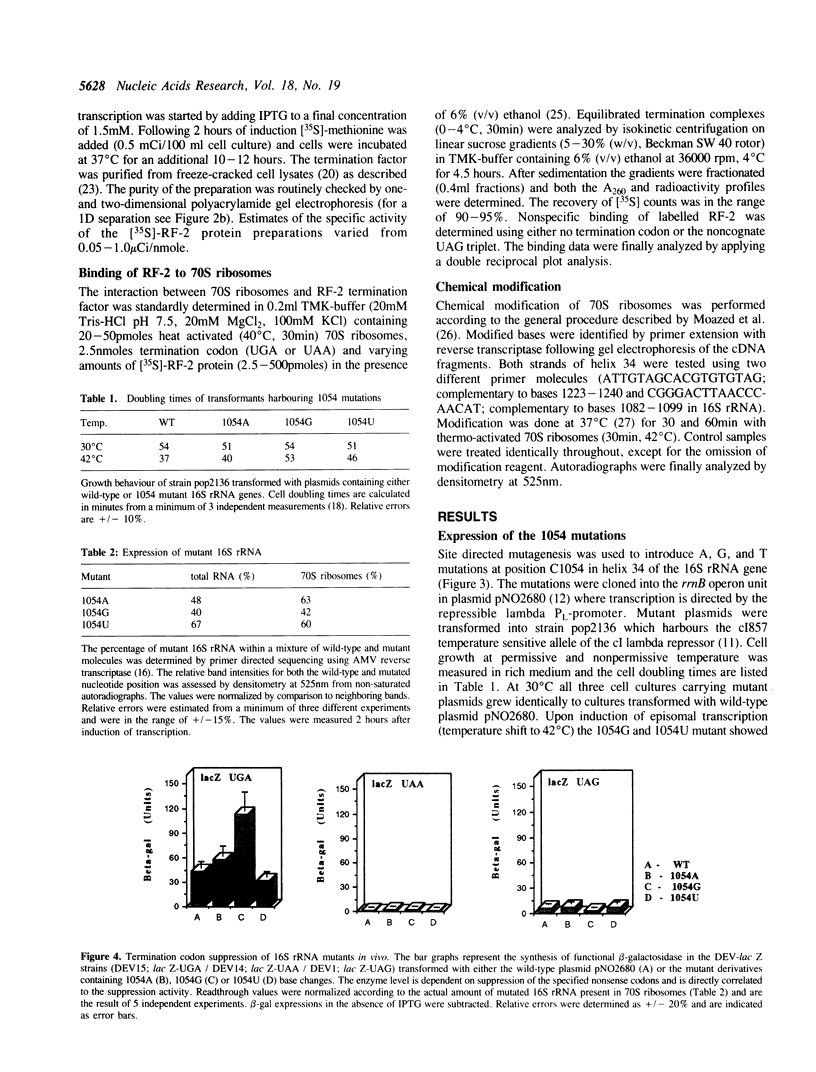
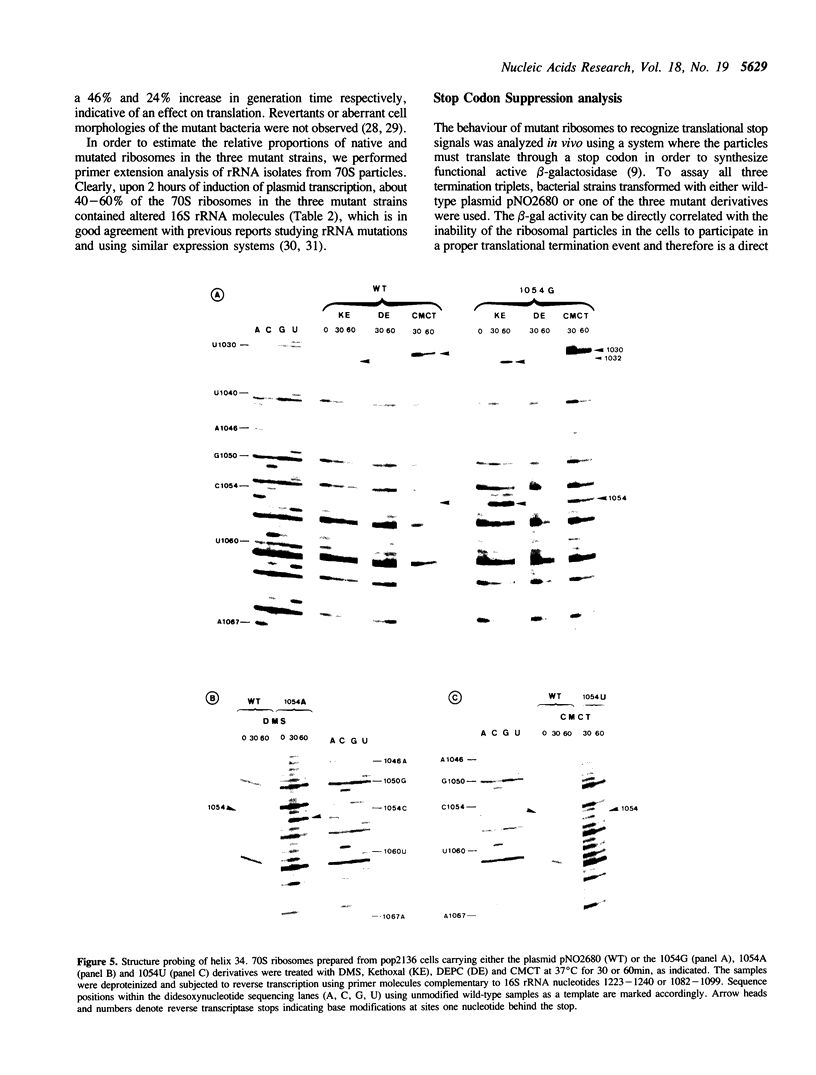
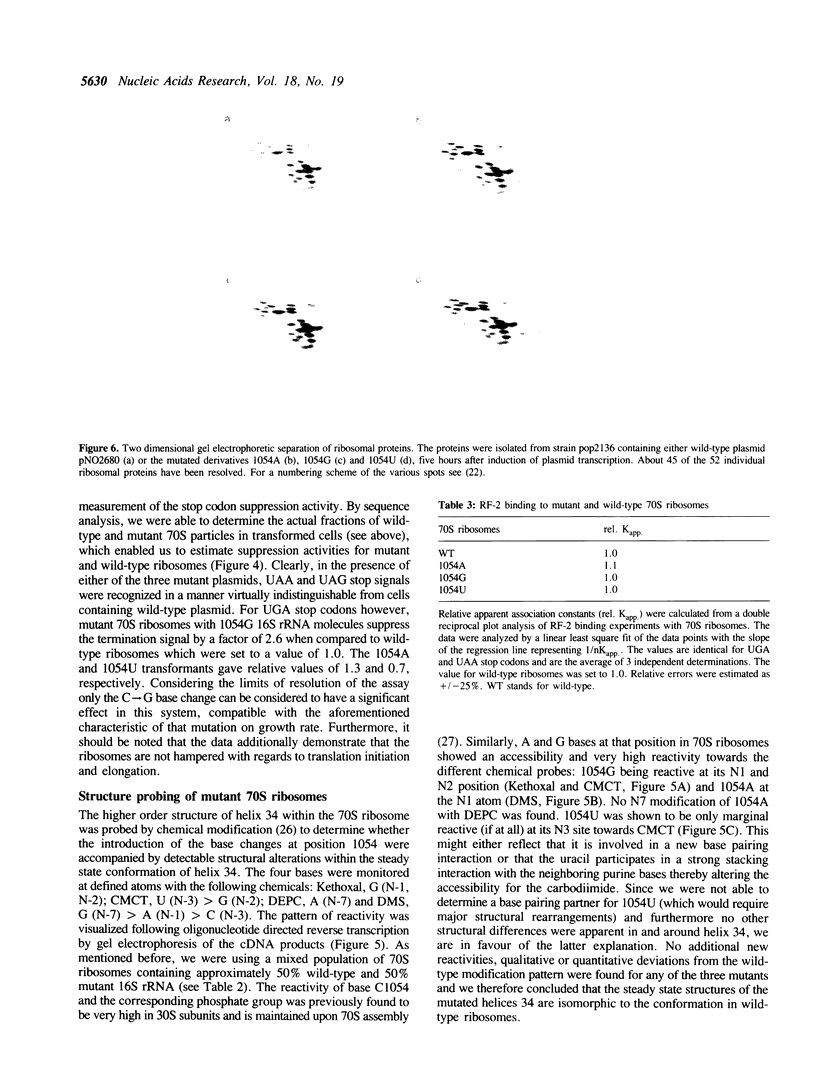
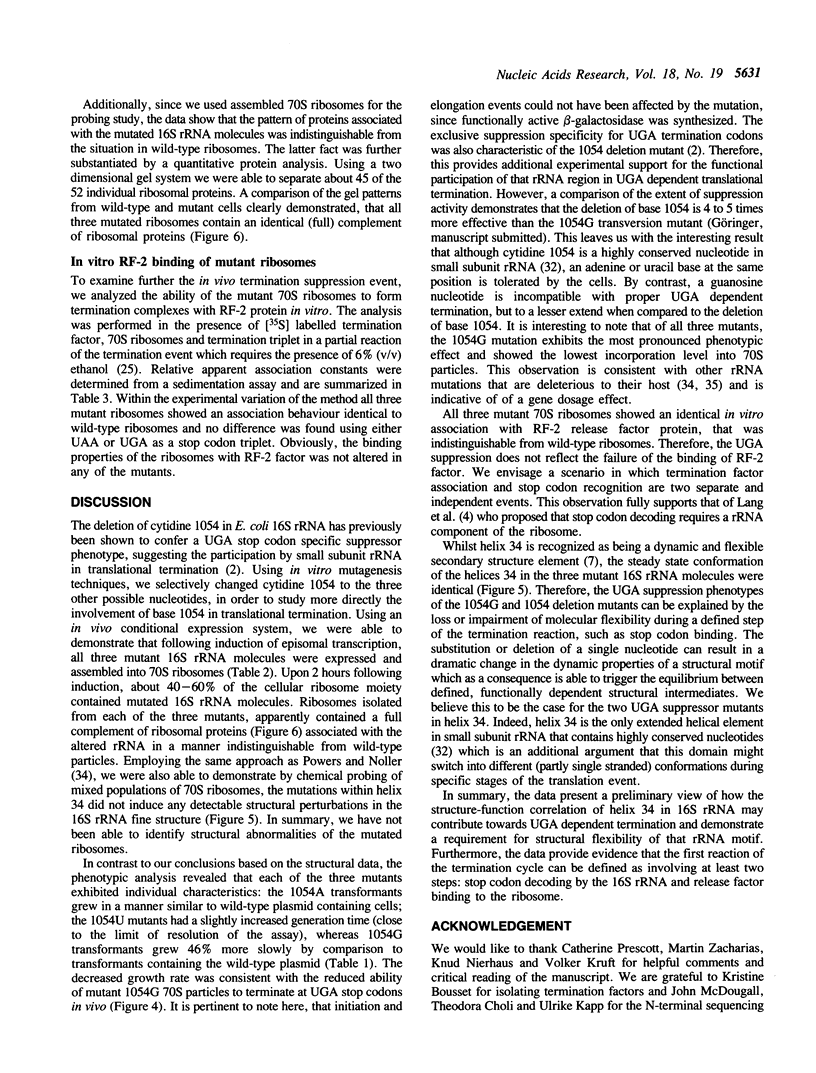
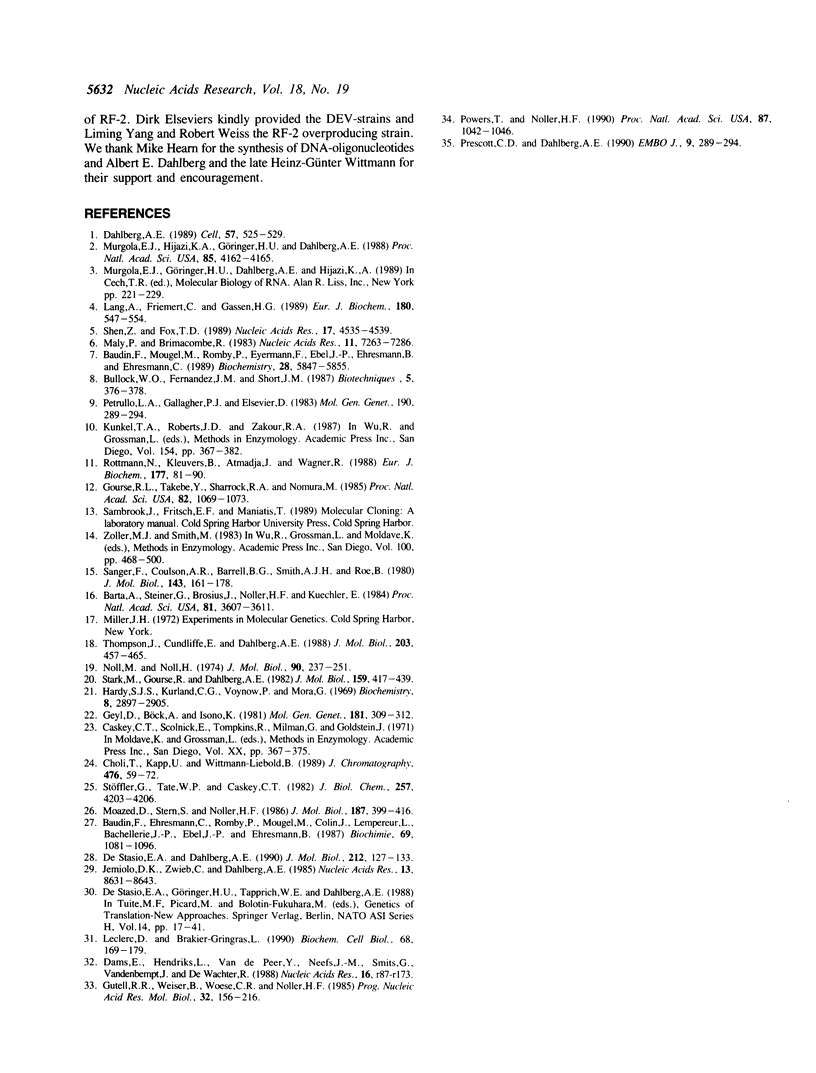
Images in this article
Selected References
These references are in PubMed. This may not be the complete list of references from this article.
- Barta A., Steiner G., Brosius J., Noller H. F., Kuechler E. Identification of a site on 23S ribosomal RNA located at the peptidyl transferase center. Proc Natl Acad Sci U S A. 1984 Jun;81(12):3607–3611. doi: 10.1073/pnas.81.12.3607. [DOI] [PMC free article] [PubMed] [Google Scholar]
- Baudin F., Ehresmann C., Romby P., Mougel M., Colin J., Lempereur L., Bachellerie J. P., Ebel J. P., Ehresmann B. Higher-order structure of domain III in Escherichia coli 16S ribosomal RNA, 30S subunit and 70S ribosome. Biochimie. 1987 Oct;69(10):1081–1096. doi: 10.1016/0300-9084(87)90008-3. [DOI] [PubMed] [Google Scholar]
- Baudin F., Mougel M., Romby P., Eyermann F., Ebel J. P., Ehresmann B., Ehresmann C. Probing the phosphates of the Escherichia coli ribosomal 16S RNA in its naked form, in the 30S subunit, and in the 70S ribosome. Biochemistry. 1989 Jul 11;28(14):5847–5855. doi: 10.1021/bi00440a022. [DOI] [PubMed] [Google Scholar]
- Choli T., Kapp U., Wittmann-Liebold B. Blotting of proteins onto Immobilon membranes. In situ characterization and comparison with high-performance liquid chromatography. J Chromatogr. 1989 Aug 4;476:59–72. doi: 10.1016/s0021-9673(01)93856-7. [DOI] [PubMed] [Google Scholar]
- Dahlberg A. E. The functional role of ribosomal RNA in protein synthesis. Cell. 1989 May 19;57(4):525–529. doi: 10.1016/0092-8674(89)90122-0. [DOI] [PubMed] [Google Scholar]
- Dams E., Hendriks L., Van de Peer Y., Neefs J. M., Smits G., Vandenbempt I., De Wachter R. Compilation of small ribosomal subunit RNA sequences. Nucleic Acids Res. 1988;16 (Suppl):r87–173. doi: 10.1093/nar/16.suppl.r87. [DOI] [PMC free article] [PubMed] [Google Scholar]
- De Stasio E. A., Dahlberg A. E. Effects of mutagenesis of a conserved base-paired site near the decoding region of Escherichia coli 16 S ribosomal RNA. J Mol Biol. 1990 Mar 5;212(1):127–133. doi: 10.1016/0022-2836(90)90309-A. [DOI] [PubMed] [Google Scholar]
- Geyl D., Böck A., Isono K. An improved method for two-dimensional gel-electrophoresis: analysis of mutationally altered ribosomal proteins of Escherichia coli. Mol Gen Genet. 1981;181(3):309–312. doi: 10.1007/BF00425603. [DOI] [PubMed] [Google Scholar]
- Gourse R. L., Takebe Y., Sharrock R. A., Nomura M. Feedback regulation of rRNA and tRNA synthesis and accumulation of free ribosomes after conditional expression of rRNA genes. Proc Natl Acad Sci U S A. 1985 Feb;82(4):1069–1073. doi: 10.1073/pnas.82.4.1069. [DOI] [PMC free article] [PubMed] [Google Scholar]
- Gutell R. R., Weiser B., Woese C. R., Noller H. F. Comparative anatomy of 16-S-like ribosomal RNA. Prog Nucleic Acid Res Mol Biol. 1985;32:155–216. doi: 10.1016/s0079-6603(08)60348-7. [DOI] [PubMed] [Google Scholar]
- Hardy S. J., Kurland C. G., Voynow P., Mora G. The ribosomal proteins of Escherichia coli. I. Purification of the 30S ribosomal proteins. Biochemistry. 1969 Jul;8(7):2897–2905. doi: 10.1021/bi00835a031. [DOI] [PubMed] [Google Scholar]
- Jemiolo D. K., Zwieb C., Dahlberg A. E. Point mutations in the 3' minor domain of 16S rRNA of E.coli. Nucleic Acids Res. 1985 Dec 9;13(23):8631–8643. doi: 10.1093/nar/13.23.8631. [DOI] [PMC free article] [PubMed] [Google Scholar]
- Lang A., Friemert C., Gassen H. G. On the role of the termination factor RF-2 and the 16S RNA in protein synthesis. Eur J Biochem. 1989 Apr 1;180(3):547–554. doi: 10.1111/j.1432-1033.1989.tb14680.x. [DOI] [PubMed] [Google Scholar]
- Leclerc D., Brakier-Gingras L. Study of the function of Escherichia coli ribosomal RNA through site-directed mutagenesis. Biochem Cell Biol. 1990 Jan;68(1):169–179. doi: 10.1139/o90-023. [DOI] [PubMed] [Google Scholar]
- Maly P., Brimacombe R. Refined secondary structure models for the 16S and 23S ribosomal RNA of Escherichia coli. Nucleic Acids Res. 1983 Nov 11;11(21):7263–7286. doi: 10.1093/nar/11.21.7263. [DOI] [PMC free article] [PubMed] [Google Scholar]
- Moazed D., Stern S., Noller H. F. Rapid chemical probing of conformation in 16 S ribosomal RNA and 30 S ribosomal subunits using primer extension. J Mol Biol. 1986 Feb 5;187(3):399–416. doi: 10.1016/0022-2836(86)90441-9. [DOI] [PubMed] [Google Scholar]
- Murgola E. J., Hijazi K. A., Göringer H. U., Dahlberg A. E. Mutant 16S ribosomal RNA: a codon-specific translational suppressor. Proc Natl Acad Sci U S A. 1988 Jun;85(12):4162–4165. doi: 10.1073/pnas.85.12.4162. [DOI] [PMC free article] [PubMed] [Google Scholar]
- Noll M., Noll H. Structural dynamics of bacterial ribosomes. III. Quantitative conversion of vacant ribosome couples into an initiation complex with R17 RNA as messenger. J Mol Biol. 1974 Dec 5;90(2):237–251. doi: 10.1016/0022-2836(74)90370-2. [DOI] [PubMed] [Google Scholar]
- Petrullo L. A., Gallagher P. J., Elseviers D. The role of 2-methylthio-N6-isopentenyladenosine in readthrough and suppression of nonsense codons in Escherichia coli. Mol Gen Genet. 1983;190(2):289–294. doi: 10.1007/BF00330653. [DOI] [PubMed] [Google Scholar]
- Powers T., Noller H. F. Dominant lethal mutations in a conserved loop in 16S rRNA. Proc Natl Acad Sci U S A. 1990 Feb;87(3):1042–1046. doi: 10.1073/pnas.87.3.1042. [DOI] [PMC free article] [PubMed] [Google Scholar]
- Prescott C. D., Dahlberg A. E. A single base change at 726 in 16S rRNA radically alters the pattern of proteins synthesized in vivo. EMBO J. 1990 Jan;9(1):289–294. doi: 10.1002/j.1460-2075.1990.tb08107.x. [DOI] [PMC free article] [PubMed] [Google Scholar]
- Rottmann N., Kleuvers B., Atmadja J., Wagner R. Mutants with base changes at the 3'-end of the 16S RNA from Escherichia coli. Construction, expression and functional analysis. Eur J Biochem. 1988 Oct 15;177(1):81–90. doi: 10.1111/j.1432-1033.1988.tb14347.x. [DOI] [PubMed] [Google Scholar]
- Sanger F., Coulson A. R., Barrell B. G., Smith A. J., Roe B. A. Cloning in single-stranded bacteriophage as an aid to rapid DNA sequencing. J Mol Biol. 1980 Oct 25;143(2):161–178. doi: 10.1016/0022-2836(80)90196-5. [DOI] [PubMed] [Google Scholar]
- Shen Z. H., Fox T. D. Substitution of an invariant nucleotide at the base of the highly conserved '530-loop' of 15S rRNA causes suppression of yeast mitochondrial ochre mutations. Nucleic Acids Res. 1989 Jun 26;17(12):4535–4539. doi: 10.1093/nar/17.12.4535. [DOI] [PMC free article] [PubMed] [Google Scholar]
- Stark M. J., Gourse R. L., Dahlberg A. E. Site-directed mutagenesis of ribosomal RNA. Analysis of ribosomal RNA deletion mutants using maxicells. J Mol Biol. 1982 Aug 15;159(3):417–439. doi: 10.1016/0022-2836(82)90292-3. [DOI] [PubMed] [Google Scholar]
- Stöffler G., Tate W. P., Caskey C. T. Ribosomal proteins cross-linked to peptide chain termination release factor 2. J Biol Chem. 1982 Apr 25;257(8):4203–4206. [PubMed] [Google Scholar]
- Thompson J., Cundliffe E., Dahlberg A. E. Site-directed mutagenesis of Escherichia coli 23 S ribosomal RNA at position 1067 within the GTP hydrolysis centre. J Mol Biol. 1988 Sep 20;203(2):457–465. doi: 10.1016/0022-2836(88)90012-5. [DOI] [PubMed] [Google Scholar]
- Zoller M. J., Smith M. Oligonucleotide-directed mutagenesis of DNA fragments cloned into M13 vectors. Methods Enzymol. 1983;100:468–500. doi: 10.1016/0076-6879(83)00074-9. [DOI] [PubMed] [Google Scholar]