Abstract
We have used a circular permutation gel shift assay to show that the 5S gene transcription factor, TFIIIA, induces a bend at the internal promoter of the Xenopus oocyte-type 5S gene. The degree of bending is comparable to what we have previously observed for TFIIIA induced bending of the Xenopus somatic-type gene [Schroth, G.P. et al. (1989) Nature 340, 487-488]. In addition, we show that TFIIIA induced DNA bending is dramatically affected by the ionic conditions used during gel electrophoresis. By modifying the conditions of the electrophoresis, we can detect two distinct conformations for the TFIIIA/DNA complex. In very low ionic strength buffers, the degree of DNA bending in the complex is estimated to be about 25 to 30 degrees, whereas in higher ionic strength buffers it is about 60 to 65 degrees. These data explain the apparent discrepancy between our results and the results of another study in which it was claimed that TFIIIA did not 'substantially' bend DNA [Zweib, C. and Brown, R.S. (1990) Nucleic Acid Res. 18, 583-587]. These results also demonstrate that the TFIIIA/DNA complex has a large degree of conformational flexibility. Both DNA bending and conformational flexibility are structural features which may provide a key insight into the function of TFIIIA as a positive transcription factor.
Full text
PDF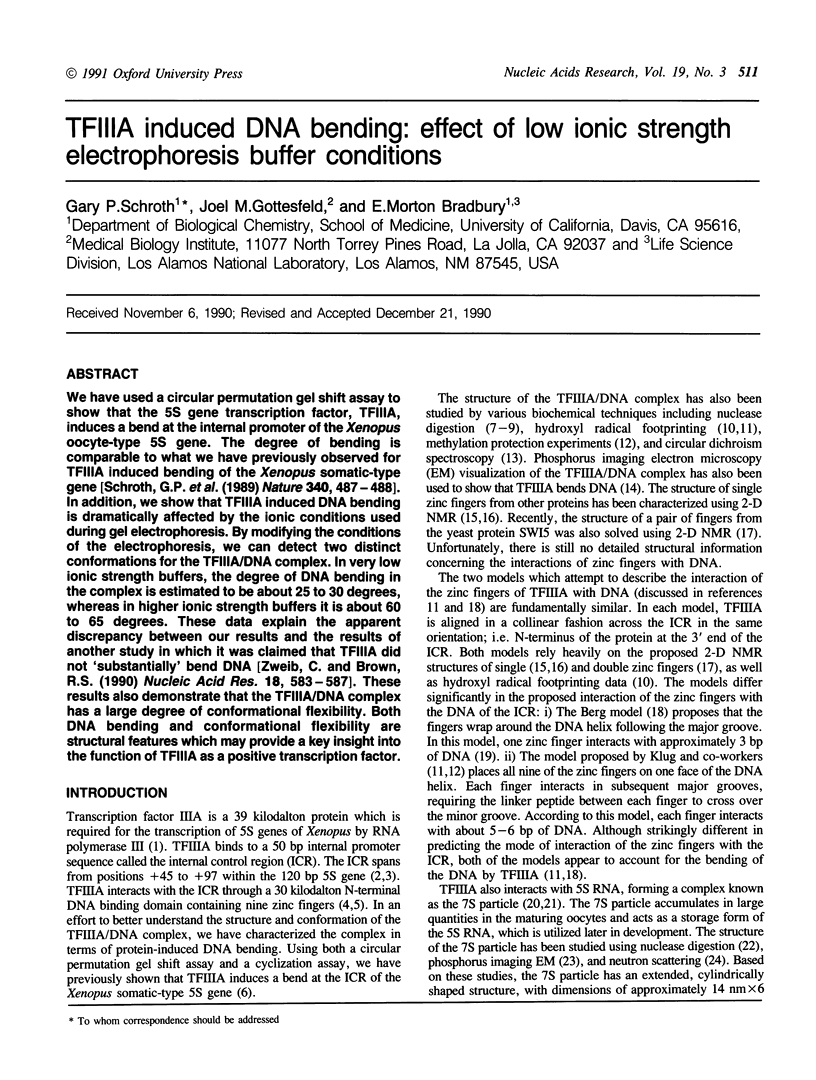
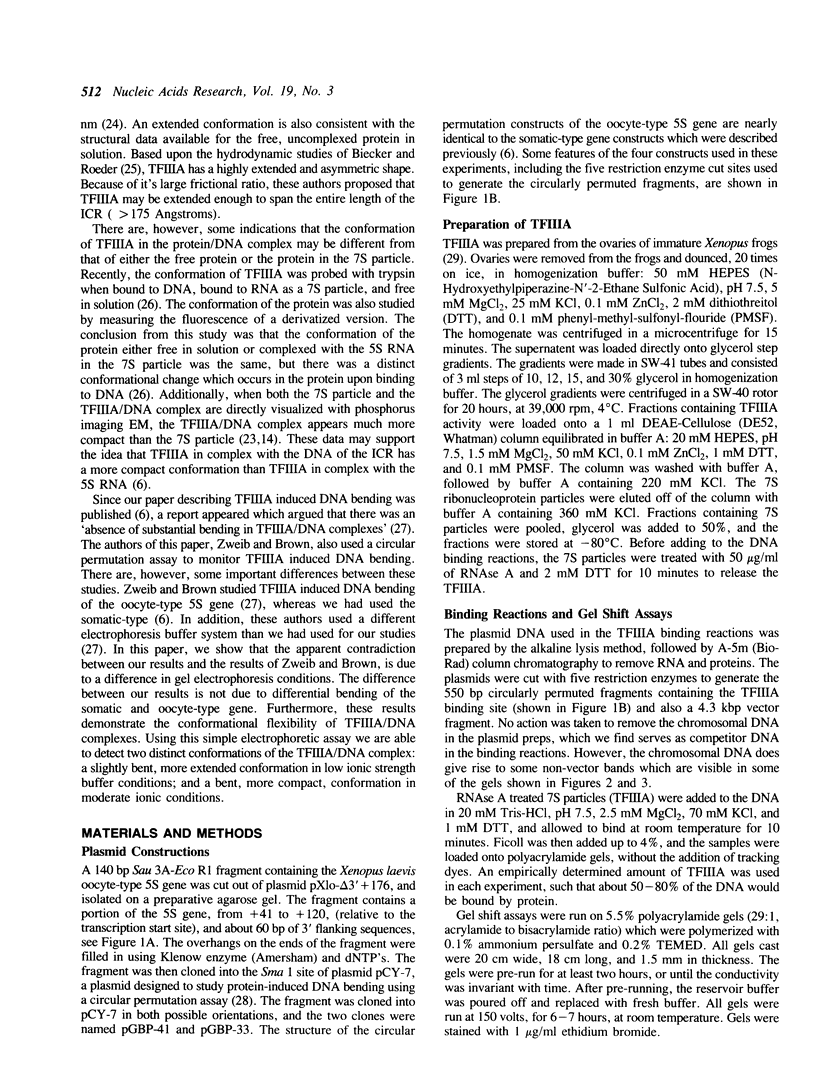
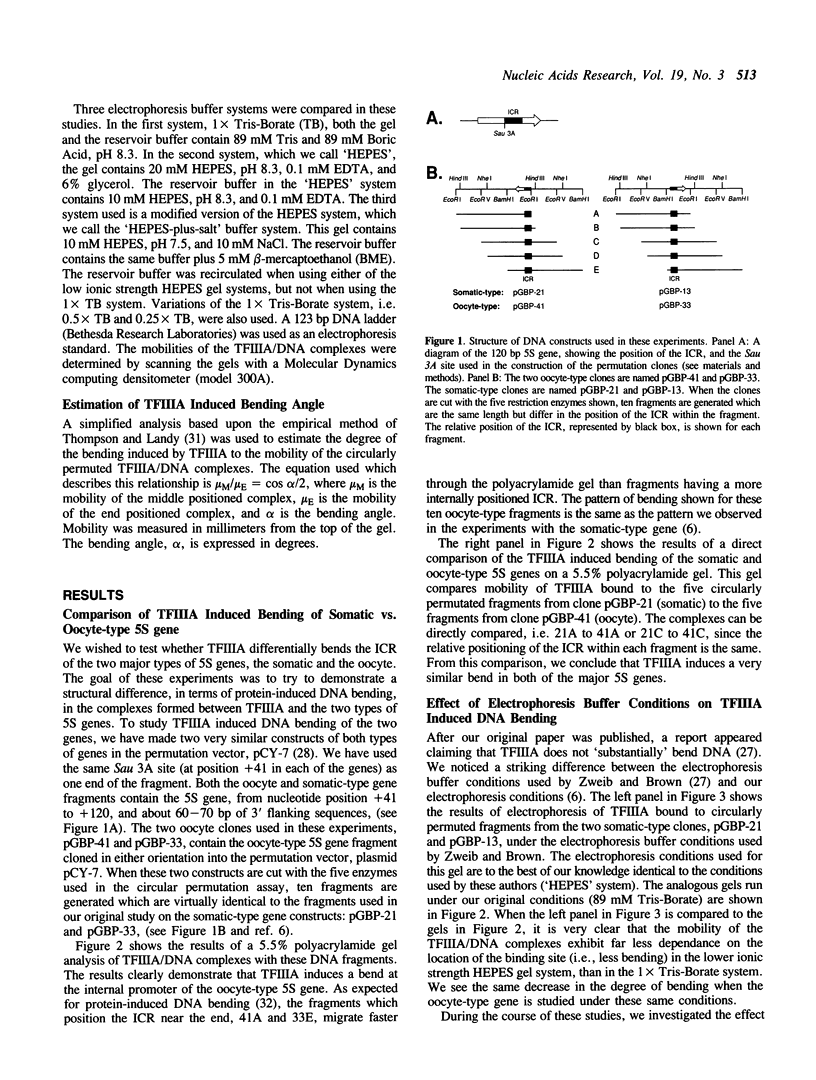
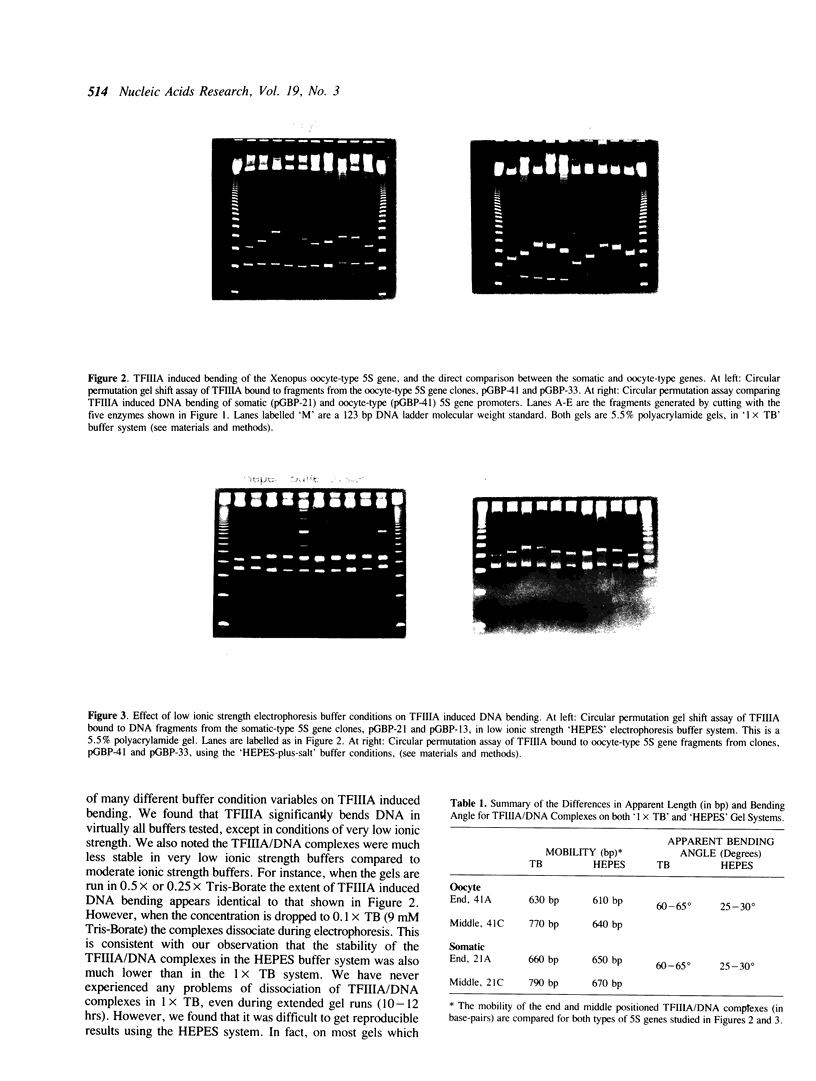
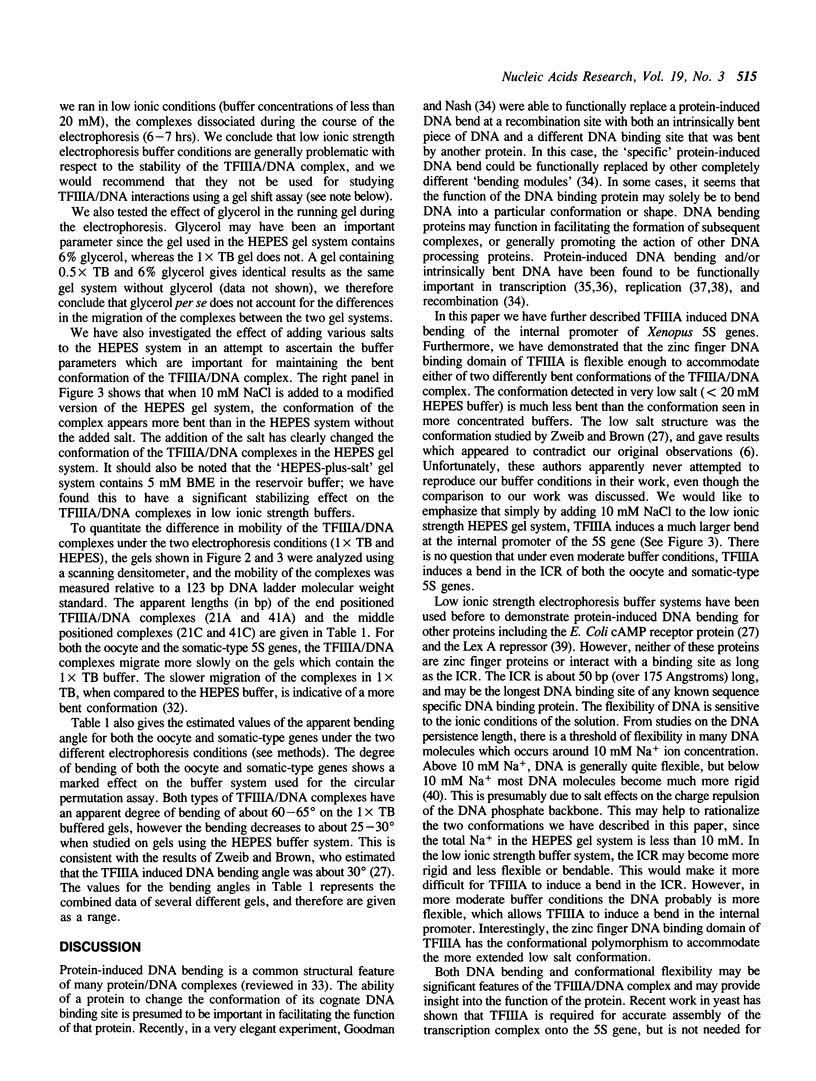
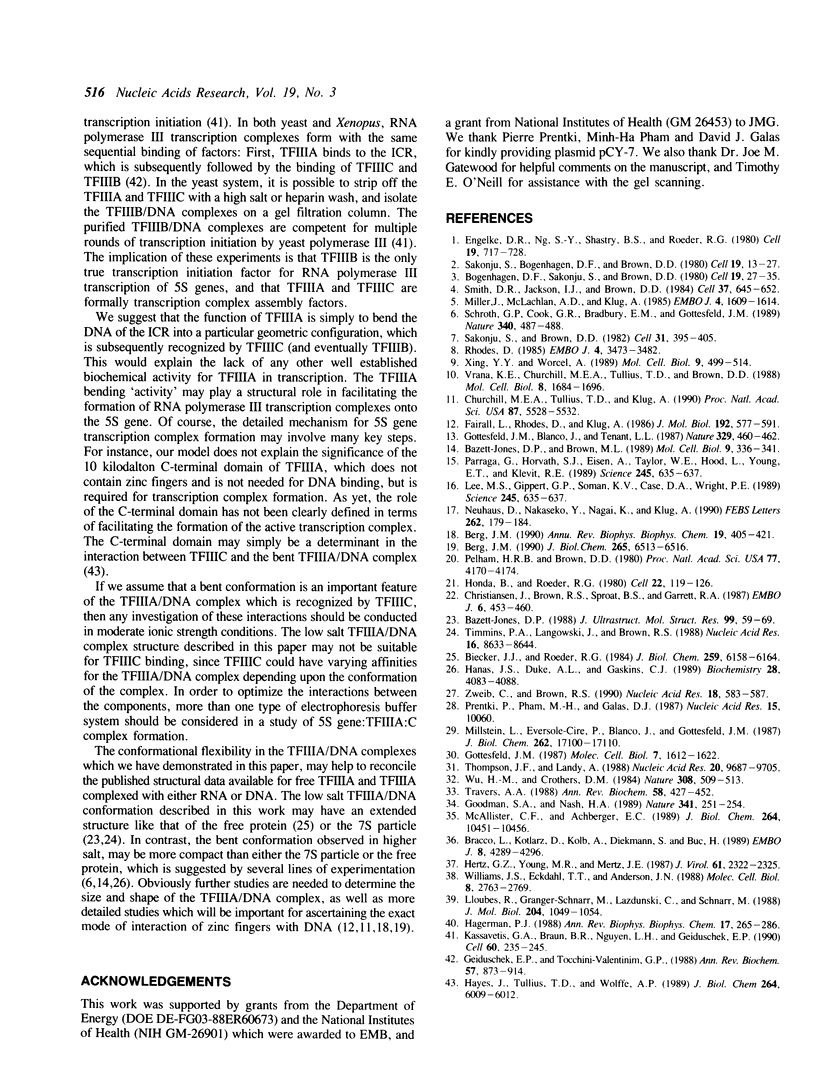
Images in this article
Selected References
These references are in PubMed. This may not be the complete list of references from this article.
- Bazett-Jones D. P., Brown M. L. Electron microscopy reveals that transcription factor TFIIIA bends 5S DNA. Mol Cell Biol. 1989 Jan;9(1):336–341. doi: 10.1128/mcb.9.1.336. [DOI] [PMC free article] [PubMed] [Google Scholar]
- Bazett-Jones D. P. Phosphorus imaging of the 7-S ribonucleoprotein particle. J Ultrastruct Mol Struct Res. 1988 Apr;99(1):59–69. doi: 10.1016/0889-1605(88)90033-x. [DOI] [PubMed] [Google Scholar]
- Berg J. M. Zinc finger domains: hypotheses and current knowledge. Annu Rev Biophys Biophys Chem. 1990;19:405–421. doi: 10.1146/annurev.bb.19.060190.002201. [DOI] [PubMed] [Google Scholar]
- Berg J. M. Zinc fingers and other metal-binding domains. Elements for interactions between macromolecules. J Biol Chem. 1990 Apr 25;265(12):6513–6516. [PubMed] [Google Scholar]
- Bieker J. J., Roeder R. G. Physical properties and DNA-binding stoichiometry of a 5 S gene-specific transcription factor. J Biol Chem. 1984 May 25;259(10):6158–6164. [PubMed] [Google Scholar]
- Bogenhagen D. F., Sakonju S., Brown D. D. A control region in the center of the 5S RNA gene directs specific initiation of transcription: II. The 3' border of the region. Cell. 1980 Jan;19(1):27–35. doi: 10.1016/0092-8674(80)90385-2. [DOI] [PubMed] [Google Scholar]
- Bracco L., Kotlarz D., Kolb A., Diekmann S., Buc H. Synthetic curved DNA sequences can act as transcriptional activators in Escherichia coli. EMBO J. 1989 Dec 20;8(13):4289–4296. doi: 10.1002/j.1460-2075.1989.tb08615.x. [DOI] [PMC free article] [PubMed] [Google Scholar]
- Christiansen J., Brown R. S., Sproat B. S., Garrett R. A. Xenopus transcription factor IIIA binds primarily at junctions between double helical stems and internal loops in oocyte 5S RNA. EMBO J. 1987 Feb;6(2):453–460. doi: 10.1002/j.1460-2075.1987.tb04775.x. [DOI] [PMC free article] [PubMed] [Google Scholar]
- Churchill M. E., Tullius T. D., Klug A. Mode of interaction of the zinc finger protein TFIIIA with a 5S RNA gene of Xenopus. Proc Natl Acad Sci U S A. 1990 Jul;87(14):5528–5532. doi: 10.1073/pnas.87.14.5528. [DOI] [PMC free article] [PubMed] [Google Scholar]
- Engelke D. R., Ng S. Y., Shastry B. S., Roeder R. G. Specific interaction of a purified transcription factor with an internal control region of 5S RNA genes. Cell. 1980 Mar;19(3):717–728. doi: 10.1016/s0092-8674(80)80048-1. [DOI] [PubMed] [Google Scholar]
- Fairall L., Rhodes D., Klug A. Mapping of the sites of protection on a 5 S RNA gene by the Xenopus transcription factor IIIA. A model for the interaction. J Mol Biol. 1986 Dec 5;192(3):577–591. doi: 10.1016/0022-2836(86)90278-0. [DOI] [PubMed] [Google Scholar]
- Geiduschek E. P., Tocchini-Valentini G. P. Transcription by RNA polymerase III. Annu Rev Biochem. 1988;57:873–914. doi: 10.1146/annurev.bi.57.070188.004301. [DOI] [PubMed] [Google Scholar]
- Goodman S. D., Nash H. A. Functional replacement of a protein-induced bend in a DNA recombination site. Nature. 1989 Sep 21;341(6239):251–254. doi: 10.1038/341251a0. [DOI] [PubMed] [Google Scholar]
- Gottesfeld J. M., Blanco J., Tennant L. L. The 5S gene internal control region is B-form both free in solution and in a complex with TFIIIA. Nature. 1987 Oct 1;329(6138):460–462. doi: 10.1038/329460a0. [DOI] [PubMed] [Google Scholar]
- Gottesfeld J. M. DNA sequence-directed nucleosome reconstitution on 5S RNA genes of Xenopus laevis. Mol Cell Biol. 1987 May;7(5):1612–1622. doi: 10.1128/mcb.7.5.1612. [DOI] [PMC free article] [PubMed] [Google Scholar]
- Hagerman P. J. Flexibility of DNA. Annu Rev Biophys Biophys Chem. 1988;17:265–286. doi: 10.1146/annurev.bb.17.060188.001405. [DOI] [PubMed] [Google Scholar]
- Hanas J. S., Duke A. L., Gaskins C. J. Conformation states of Xenopus transcription factor IIIA. Biochemistry. 1989 May 2;28(9):4083–4088. doi: 10.1021/bi00435a068. [DOI] [PubMed] [Google Scholar]
- Hayes J., Tullius T. D., Wolffe A. P. A protein-protein interaction is essential for stable complex formation on a 5 S RNA gene. J Biol Chem. 1989 Apr 15;264(11):6009–6012. [PubMed] [Google Scholar]
- Hertz G. Z., Young M. R., Mertz J. E. The A+T-rich sequence of the simian virus 40 origin is essential for replication and is involved in bending of the viral DNA. J Virol. 1987 Jul;61(7):2322–2325. doi: 10.1128/jvi.61.7.2322-2325.1987. [DOI] [PMC free article] [PubMed] [Google Scholar]
- Honda B. M., Roeder R. G. Association of a 5S gene transcription factor with 5S RNA and altered levels of the factor during cell differentiation. Cell. 1980 Nov;22(1 Pt 1):119–126. doi: 10.1016/0092-8674(80)90160-9. [DOI] [PubMed] [Google Scholar]
- Kassavetis G. A., Braun B. R., Nguyen L. H., Geiduschek E. P. S. cerevisiae TFIIIB is the transcription initiation factor proper of RNA polymerase III, while TFIIIA and TFIIIC are assembly factors. Cell. 1990 Jan 26;60(2):235–245. doi: 10.1016/0092-8674(90)90739-2. [DOI] [PubMed] [Google Scholar]
- Lee M. S., Gippert G. P., Soman K. V., Case D. A., Wright P. E. Three-dimensional solution structure of a single zinc finger DNA-binding domain. Science. 1989 Aug 11;245(4918):635–637. doi: 10.1126/science.2503871. [DOI] [PubMed] [Google Scholar]
- Lee M. S., Gippert G. P., Soman K. V., Case D. A., Wright P. E. Three-dimensional solution structure of a single zinc finger DNA-binding domain. Science. 1989 Aug 11;245(4918):635–637. doi: 10.1126/science.2503871. [DOI] [PubMed] [Google Scholar]
- Lloubès R., Granger-Schnarr M., Lazdunski C., Schnarr M. LexA repressor induces operator-dependent DNA bending. J Mol Biol. 1988 Dec 20;204(4):1049–1054. doi: 10.1016/0022-2836(88)90062-9. [DOI] [PubMed] [Google Scholar]
- McAllister C. F., Achberger E. C. Rotational orientation of upstream curved DNA affects promoter function in Bacillus subtilis. J Biol Chem. 1989 Jun 25;264(18):10451–10456. [PubMed] [Google Scholar]
- Miller J., McLachlan A. D., Klug A. Repetitive zinc-binding domains in the protein transcription factor IIIA from Xenopus oocytes. EMBO J. 1985 Jun;4(6):1609–1614. doi: 10.1002/j.1460-2075.1985.tb03825.x. [DOI] [PMC free article] [PubMed] [Google Scholar]
- Millstein L., Eversole-Cire P., Blanco J., Gottesfeld J. M. Differential transcription of Xenopus oocyte and somatic-type 5 S genes in a Xenopus oocyte extract. J Biol Chem. 1987 Dec 15;262(35):17100–17110. [PubMed] [Google Scholar]
- Neuhaus D., Nakaseko Y., Nagai K., Klug A. Sequence-specific [1H]NMR resonance assignments and secondary structure identification for 1- and 2-zinc finger constructs from SW15. A hydrophobic core involving four invariant residues. FEBS Lett. 1990 Mar 26;262(2):179–184. doi: 10.1016/0014-5793(90)80184-k. [DOI] [PubMed] [Google Scholar]
- Pelham H. R., Brown D. D. A specific transcription factor that can bind either the 5S RNA gene or 5S RNA. Proc Natl Acad Sci U S A. 1980 Jul;77(7):4170–4174. doi: 10.1073/pnas.77.7.4170. [DOI] [PMC free article] [PubMed] [Google Scholar]
- Prentki P., Pham M. H., Galas D. J. Plasmid permutation vectors to monitor DNA bending. Nucleic Acids Res. 1987 Dec 10;15(23):10060–10060. doi: 10.1093/nar/15.23.10060. [DOI] [PMC free article] [PubMed] [Google Scholar]
- Rhodes D. Structural analysis of a triple complex between the histone octamer, a Xenopus gene for 5S RNA and transcription factor IIIA. EMBO J. 1985 Dec 16;4(13A):3473–3482. doi: 10.1002/j.1460-2075.1985.tb04106.x. [DOI] [PMC free article] [PubMed] [Google Scholar]
- Sakonju S., Brown D. D. Contact points between a positive transcription factor and the Xenopus 5S RNA gene. Cell. 1982 Dec;31(2 Pt 1):395–405. doi: 10.1016/0092-8674(82)90133-7. [DOI] [PubMed] [Google Scholar]
- Schroth G. P., Cook G. R., Bradbury E. M., Gottesfeld J. M. Transcription factor IIIA induced bending of the Xenopus somatic 5S gene promoter. Nature. 1989 Aug 10;340(6233):487–488. doi: 10.1038/340487a0. [DOI] [PubMed] [Google Scholar]
- Smith D. R., Jackson I. J., Brown D. D. Domains of the positive transcription factor specific for the Xenopus 5S RNA gene. Cell. 1984 Jun;37(2):645–652. doi: 10.1016/0092-8674(84)90396-9. [DOI] [PubMed] [Google Scholar]
- Thompson J. F., Landy A. Empirical estimation of protein-induced DNA bending angles: applications to lambda site-specific recombination complexes. Nucleic Acids Res. 1988 Oct 25;16(20):9687–9705. doi: 10.1093/nar/16.20.9687. [DOI] [PMC free article] [PubMed] [Google Scholar]
- Timmins P. A., Langowski J., Brown R. S. An elongated model of the Xenopus laevis transcription factor IIIA-5S ribosomal RNA complex derived from neutron scattering and hydrodynamic measurements. Nucleic Acids Res. 1988 Sep 12;16(17):8633–8644. doi: 10.1093/nar/16.17.8633. [DOI] [PMC free article] [PubMed] [Google Scholar]
- Travers A. A. DNA conformation and protein binding. Annu Rev Biochem. 1989;58:427–452. doi: 10.1146/annurev.bi.58.070189.002235. [DOI] [PubMed] [Google Scholar]
- Vrana K. E., Churchill M. E., Tullius T. D., Brown D. D. Mapping functional regions of transcription factor TFIIIA. Mol Cell Biol. 1988 Apr;8(4):1684–1696. doi: 10.1128/mcb.8.4.1684. [DOI] [PMC free article] [PubMed] [Google Scholar]
- Williams J. S., Eckdahl T. T., Anderson J. N. Bent DNA functions as a replication enhancer in Saccharomyces cerevisiae. Mol Cell Biol. 1988 Jul;8(7):2763–2769. doi: 10.1128/mcb.8.7.2763. [DOI] [PMC free article] [PubMed] [Google Scholar]
- Wu H. M., Crothers D. M. The locus of sequence-directed and protein-induced DNA bending. Nature. 1984 Apr 5;308(5959):509–513. doi: 10.1038/308509a0. [DOI] [PubMed] [Google Scholar]
- Xing Y. Y., Worcel A. The C-terminal domain of transcription factor IIIA interacts differently with different 5S RNA genes. Mol Cell Biol. 1989 Feb;9(2):499–514. doi: 10.1128/mcb.9.2.499. [DOI] [PMC free article] [PubMed] [Google Scholar]
- Zwieb C., Brown R. S. Absence of substantial bending in Xenopus laevis transcription factor IIIA-DNA complexes. Nucleic Acids Res. 1990 Feb 11;18(3):583–587. doi: 10.1093/nar/18.3.583. [DOI] [PMC free article] [PubMed] [Google Scholar]