Abstract
Identifying the specific DNA sequences involved in the chromosomal abnormalities in developmental and neoplastic diseases may be essential to understanding the molecular biology of these disorders. The use of recombinant DNA techniques in conjunction with rodent-human hybrid cells makes it possible to assign chromosomal locations to specific DNA sequences. However, the ubiquitous presence of reiterated DNA species often complicates the application of straightforward molecular hybridization. To accelerate the mapping of cloned sequences to specific chromosomal locations, we investigated the possibility that cloned sequences containing reiterated DNA might be used without isolating unique sequences. By varying conditions of hybridization (specifically temperature) and using restricted DNA samples from human genomic DNA, Chinese hamster ovary-human chromosome 11 hybrids, and non-chromosome 11 hybrids, we have been able to assign cloned DNA sequences containing reiterated sequences to their chromosome of origin. By hybridization under the high-stringency condition of 55 degrees C, specific banding was produced with both human genomic DNA and the human-chromosome-containing hybrid from which the probe was prepared. Furthermore, using a panel of chromosome 11 deletion mutants, we have been able to assign a cloned sequence to a specific chromosomal location. We believe that this approach will accelerate gene mapping procedures and facilitate identification of DNA sequences involved in chromosomal abnormalities.
Full text
PDF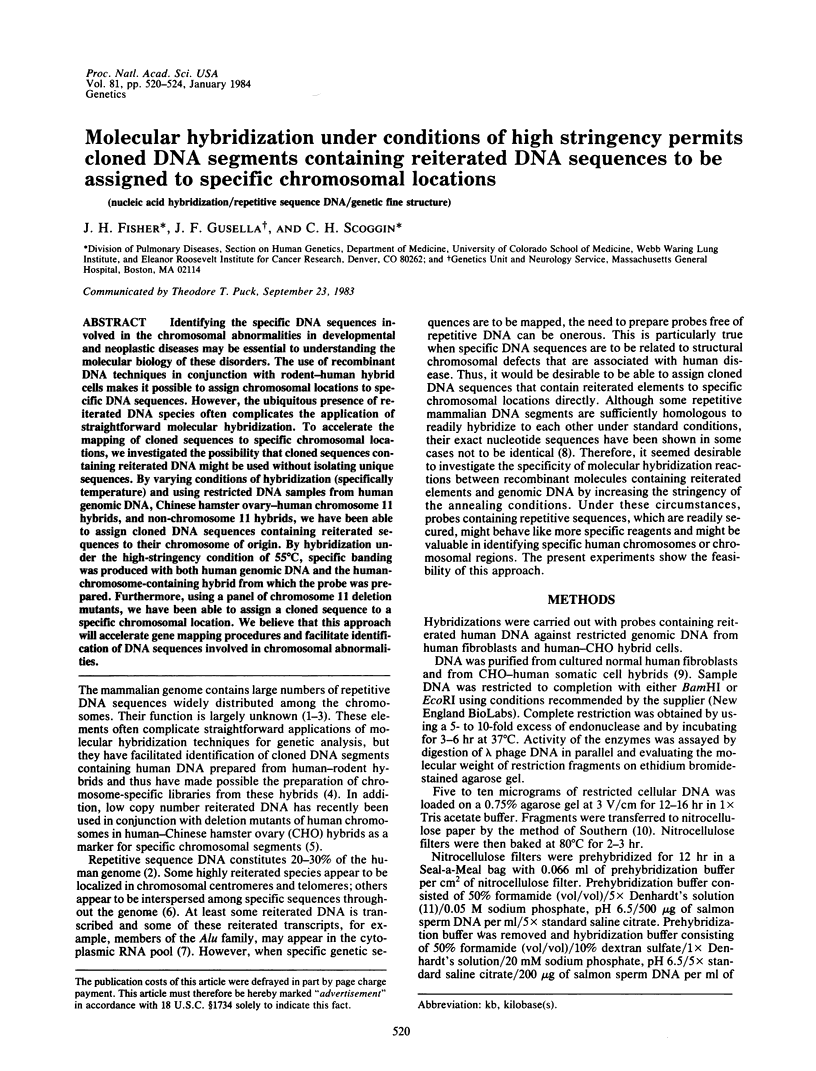
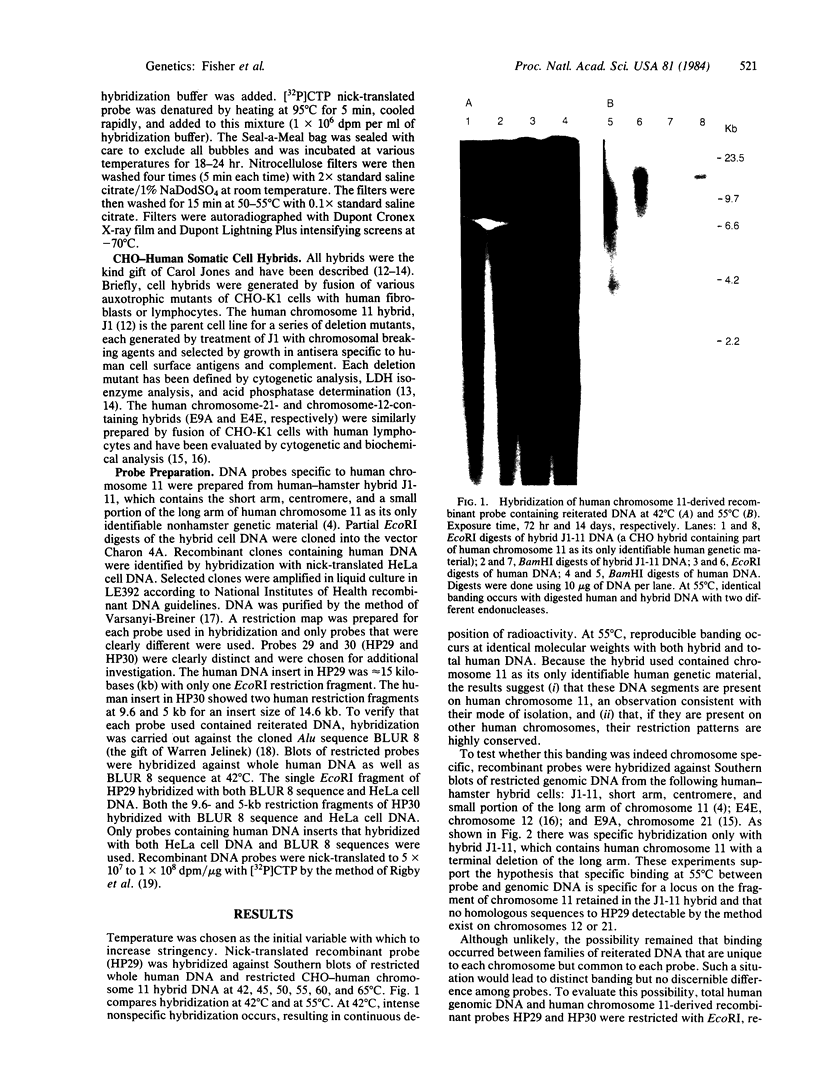
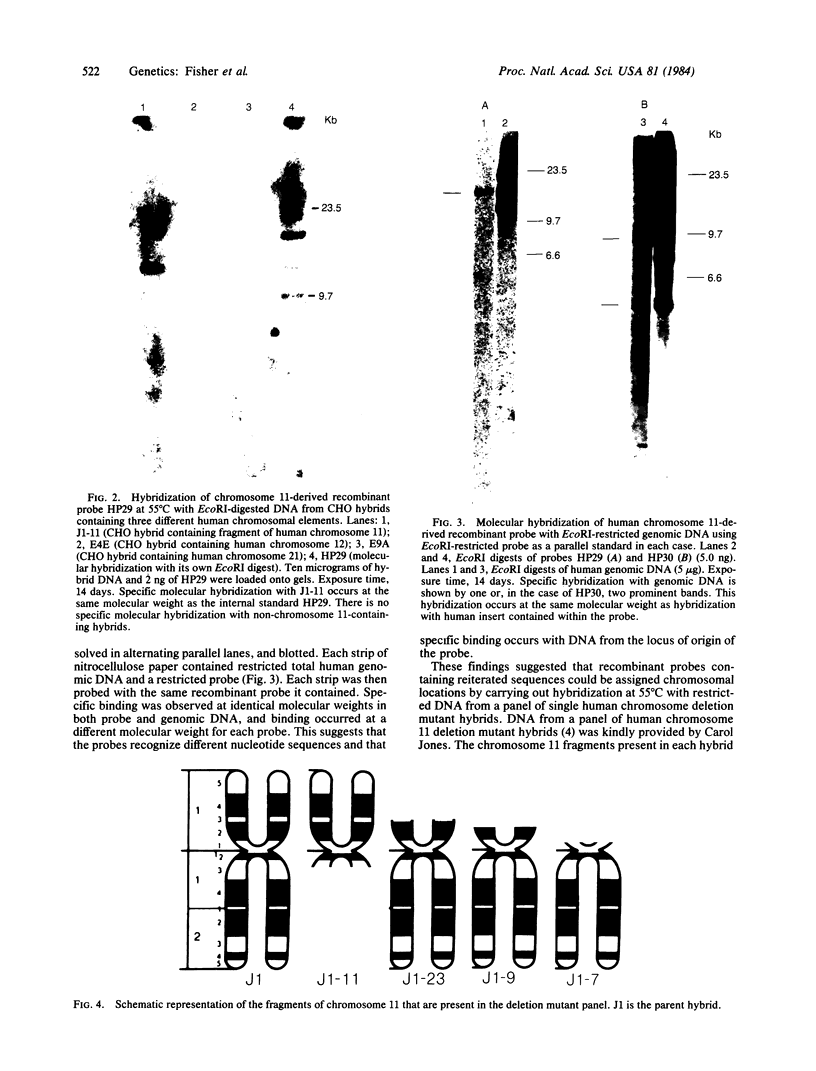
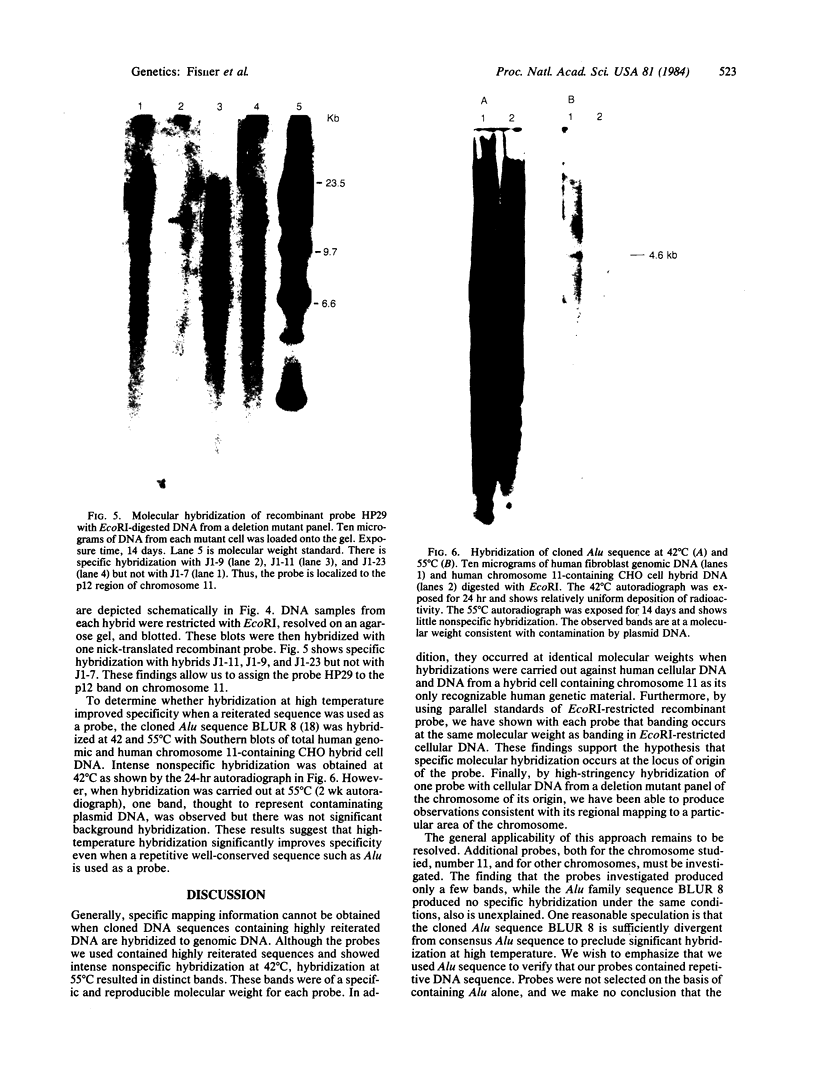
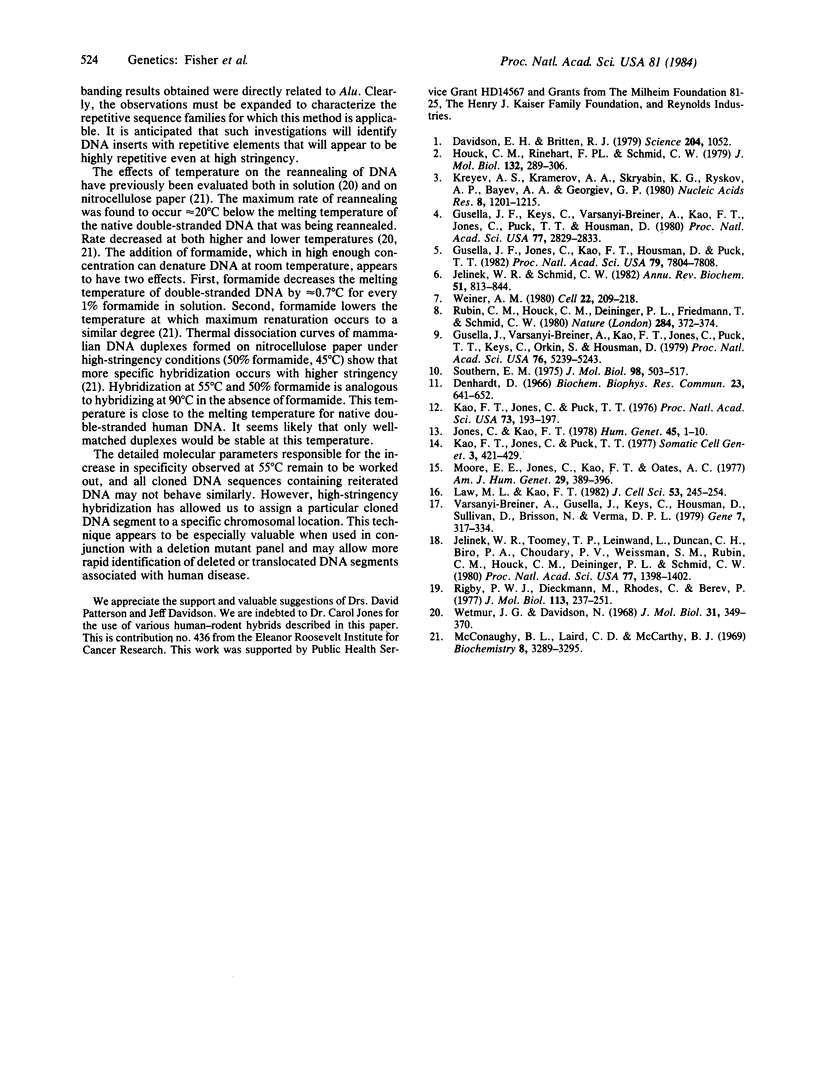
Images in this article
Selected References
These references are in PubMed. This may not be the complete list of references from this article.
- Davidson E. H., Britten R. J. Regulation of gene expression: possible role of repetitive sequences. Science. 1979 Jun 8;204(4397):1052–1059. doi: 10.1126/science.451548. [DOI] [PubMed] [Google Scholar]
- Denhardt D. T. A membrane-filter technique for the detection of complementary DNA. Biochem Biophys Res Commun. 1966 Jun 13;23(5):641–646. doi: 10.1016/0006-291x(66)90447-5. [DOI] [PubMed] [Google Scholar]
- Gusella J. F., Jones C., Kao F. T., Housman D., Puck T. T. Genetic fine-structure mapping in human chromosome 11 by use of repetitive DNA sequences. Proc Natl Acad Sci U S A. 1982 Dec;79(24):7804–7808. doi: 10.1073/pnas.79.24.7804. [DOI] [PMC free article] [PubMed] [Google Scholar]
- Gusella J. F., Keys C., VarsanyiBreiner A., Kao F. T., Jones C., Puck T. T., Housman D. Isolation and localization of DNA segments from specific human chromosomes. Proc Natl Acad Sci U S A. 1980 May;77(5):2829–2833. doi: 10.1073/pnas.77.5.2829. [DOI] [PMC free article] [PubMed] [Google Scholar]
- Gusella J., Varsanyi-Breiner A., Kao F. T., Jones C., Puck T. T., Keys C., Orkin S., Housman D. Precise localization of human beta-globin gene complex on chromosome 11. Proc Natl Acad Sci U S A. 1979 Oct;76(10):5239–5242. doi: 10.1073/pnas.76.10.5239. [DOI] [PMC free article] [PubMed] [Google Scholar]
- Houck C. M., Rinehart F. P., Schmid C. W. A ubiquitous family of repeated DNA sequences in the human genome. J Mol Biol. 1979 Aug 15;132(3):289–306. doi: 10.1016/0022-2836(79)90261-4. [DOI] [PubMed] [Google Scholar]
- Jelinek W. R., Schmid C. W. Repetitive sequences in eukaryotic DNA and their expression. Annu Rev Biochem. 1982;51:813–844. doi: 10.1146/annurev.bi.51.070182.004121. [DOI] [PubMed] [Google Scholar]
- Jelinek W. R., Toomey T. P., Leinwand L., Duncan C. H., Biro P. A., Choudary P. V., Weissman S. M., Rubin C. M., Houck C. M., Deininger P. L. Ubiquitous, interspersed repeated sequences in mammalian genomes. Proc Natl Acad Sci U S A. 1980 Mar;77(3):1398–1402. doi: 10.1073/pnas.77.3.1398. [DOI] [PMC free article] [PubMed] [Google Scholar]
- Jones C., Kao F. T. Regional mapping of the gene for human lysosomal acid phosphatase (ACP2) using a hybrid clone panel containing segments of human chromosome 11. Hum Genet. 1978 Nov 24;45(1):1–10. doi: 10.1007/BF00277567. [DOI] [PubMed] [Google Scholar]
- Kao F. T., Jones C., Puck T. T. Genetics of cell-surface antigens: regional mapping of three components of the human cell-surface antigen complex, AL, on chromosome 11. Somatic Cell Genet. 1977 Jul;3(4):421–429. doi: 10.1007/BF01542970. [DOI] [PubMed] [Google Scholar]
- Kao F. T., Jones C., Puck T. T. Genetics of somatic mammalian cells: genetic, immunologic, and biochemical analysis with Chinese hamster cell hybrids containing selected human chromosomes. Proc Natl Acad Sci U S A. 1976 Jan;73(1):193–197. doi: 10.1073/pnas.73.1.193. [DOI] [PMC free article] [PubMed] [Google Scholar]
- Krayev A. S., Kramerov D. A., Skryabin K. G., Ryskov A. P., Bayev A. A., Georgiev G. P. The nucleotide sequence of the ubiquitous repetitive DNA sequence B1 complementary to the most abundant class of mouse fold-back RNA. Nucleic Acids Res. 1980 Mar 25;8(6):1201–1215. doi: 10.1093/nar/8.6.1201. [DOI] [PMC free article] [PubMed] [Google Scholar]
- Law M. L., Kao F. T. Regional mapping of the gene coding for enolase-2 on human chromosome 12. J Cell Sci. 1982 Feb;53:245–254. doi: 10.1242/jcs.53.1.245. [DOI] [PubMed] [Google Scholar]
- McConaughy B. L., Laird C. D., McCarthy B. J. Nucleic acid reassociation in formamide. Biochemistry. 1969 Aug;8(8):3289–3295. doi: 10.1021/bi00836a024. [DOI] [PubMed] [Google Scholar]
- Moore E. E., Jones C., Kao F. T., Oates D. C. Synteny between glycinamide ribonucleotide synthetase and superoxide dismutase (soluble). Am J Hum Genet. 1977 Jul;29(4):389–396. [PMC free article] [PubMed] [Google Scholar]
- Rigby P. W., Dieckmann M., Rhodes C., Berg P. Labeling deoxyribonucleic acid to high specific activity in vitro by nick translation with DNA polymerase I. J Mol Biol. 1977 Jun 15;113(1):237–251. doi: 10.1016/0022-2836(77)90052-3. [DOI] [PubMed] [Google Scholar]
- Rubin C. M., Houck C. M., Deininger P. L., Friedmann T., Schmid C. W. Partial nucleotide sequence of the 300-nucleotide interspersed repeated human DNA sequences. Nature. 1980 Mar 27;284(5754):372–374. doi: 10.1038/284372a0. [DOI] [PubMed] [Google Scholar]
- Southern E. M. Detection of specific sequences among DNA fragments separated by gel electrophoresis. J Mol Biol. 1975 Nov 5;98(3):503–517. doi: 10.1016/s0022-2836(75)80083-0. [DOI] [PubMed] [Google Scholar]
- Varsanyi-Breiner A., Gusella J. F., Keys C., Housman D. E., Sullivan D., Brisson N., Verma D. P. The organization of a nuclear DNA sequence from a higher plant: molecular cloning and characterization of soybean ribosomal DNA. Gene. 1979 Nov;7(3-4):317–334. doi: 10.1016/0378-1119(79)90051-9. [DOI] [PubMed] [Google Scholar]
- Weiner A. M. An abundant cytoplasmic 7S RNA is complementary to the dominant interspersed middle repetitive DNA sequence family in the human genome. Cell. 1980 Nov;22(1 Pt 1):209–218. doi: 10.1016/0092-8674(80)90169-5. [DOI] [PubMed] [Google Scholar]
- Wetmur J. G., Davidson N. Kinetics of renaturation of DNA. J Mol Biol. 1968 Feb 14;31(3):349–370. doi: 10.1016/0022-2836(68)90414-2. [DOI] [PubMed] [Google Scholar]