Abstract
Patch clamp techniques were used to study ionic currents in cultured mouse peritoneal macrophages. Whole-cell voltage clamp studies of cells 1-5 hr after isolation showed only a high-resistance linear membrane. After 1 day in culture, 82 of 85 cells studied had developed a voltage- and time-dependent potassium (K+) conductance similar to the delayed outward rectifier in nerve and muscle cells. The current activated when the membrane was depolarized above -50 mV. The sigmoidally rising current rose to a peak at a rate that increased with depolarization. Inactivation proceeded exponentially with a time constant of approximately equal to 450 ms. Recovery from inactivation was slow (tau = 12 s). The reversal potentials for varying extracellular K+ concentrations followed the Nernst predictions for a K+ -specific channel. The conductance was blocked by extracellular 4-aminopyridine and by intracellular tetraethylammonium chloride, barium, and cesium. Single-channel K+ currents comprising this net current had a conductance of 16 pS, exhibited bursting behavior, and inactivated with time. No inward currents were ever detected in macrophages cultivated for up to 4 days. Short-term exposure to chemoattractant and transmitter agents failed to activate an inward current. Macrophages may change their membrane electrophysiological properties depending on their state of functional activation. We postulate that the K+ conductance develops prior to depolarizing conductances involved in the macrophage's immunological functions.
Full text
PDF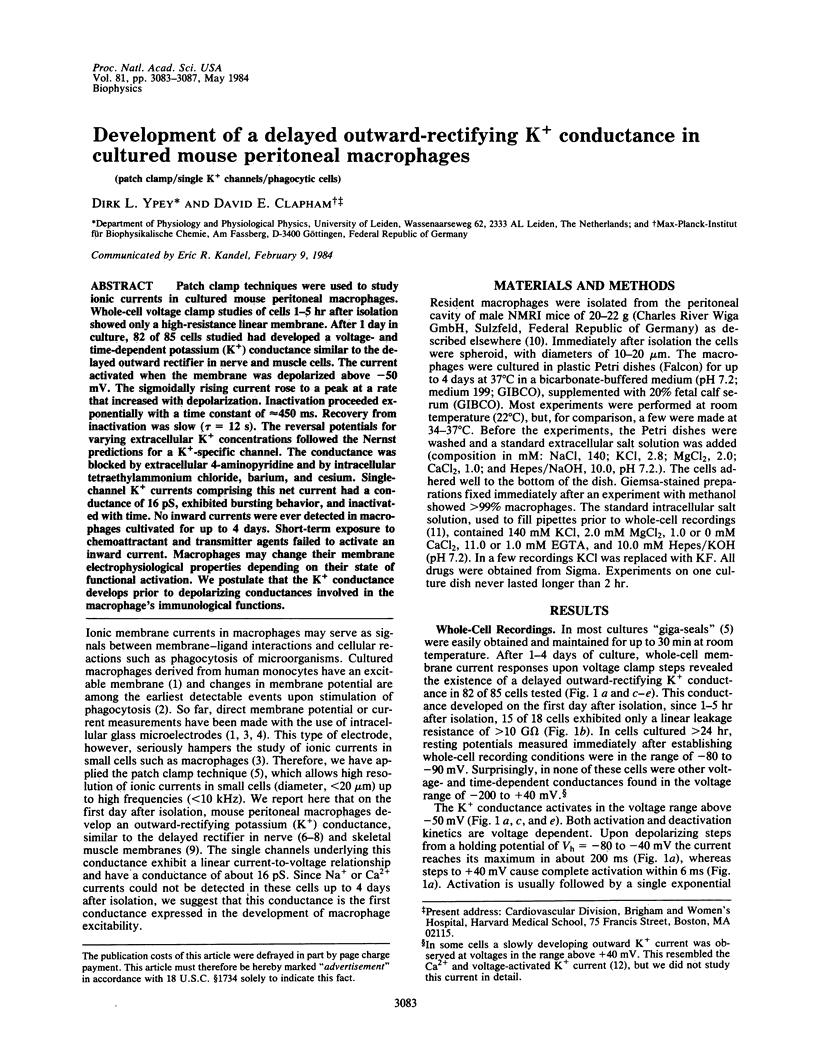
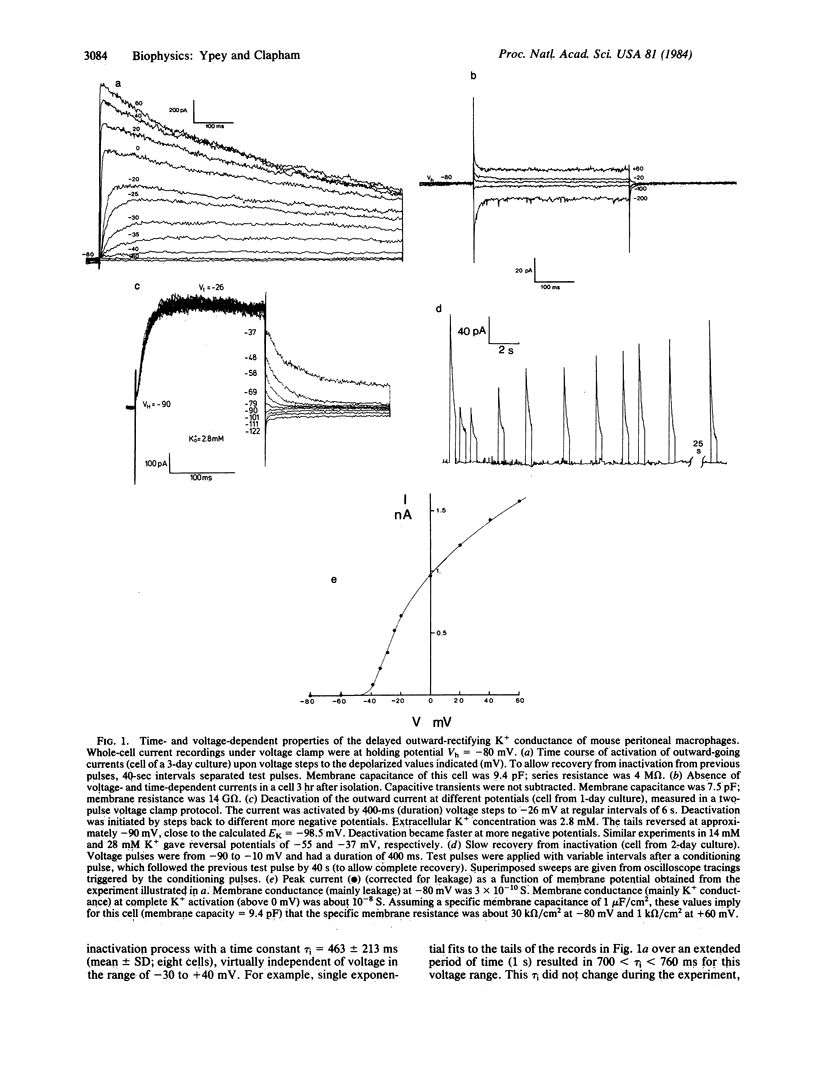
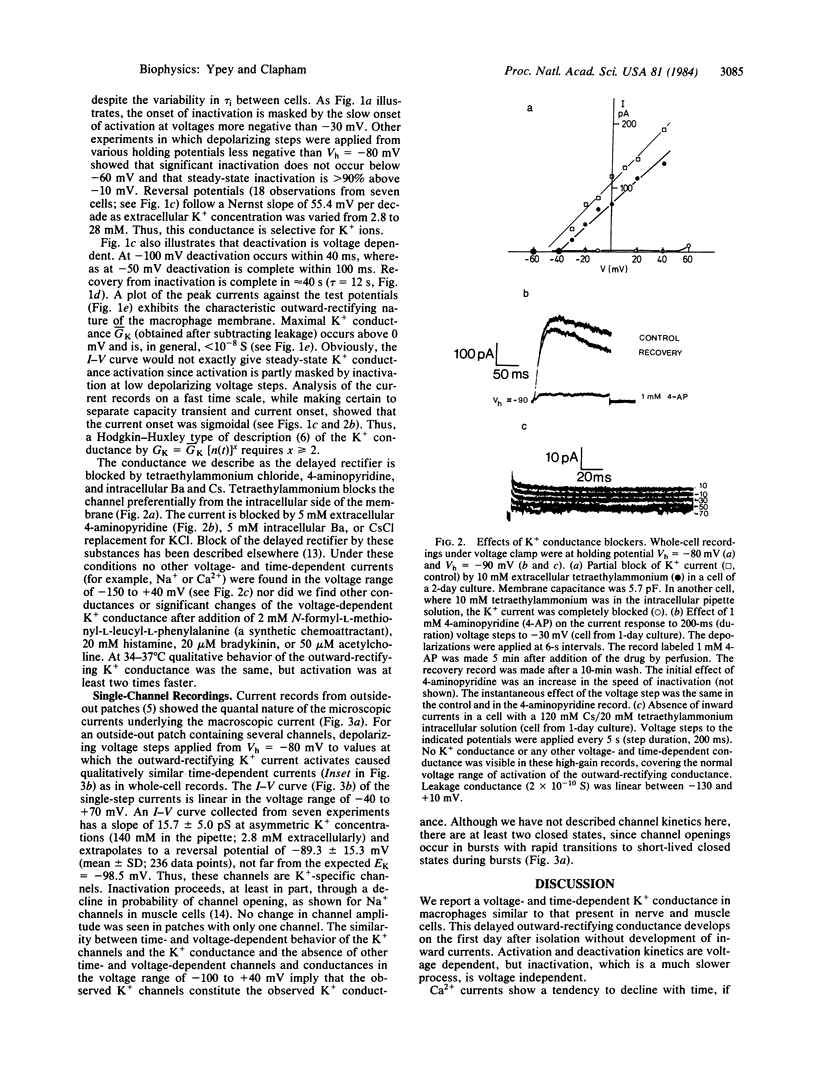
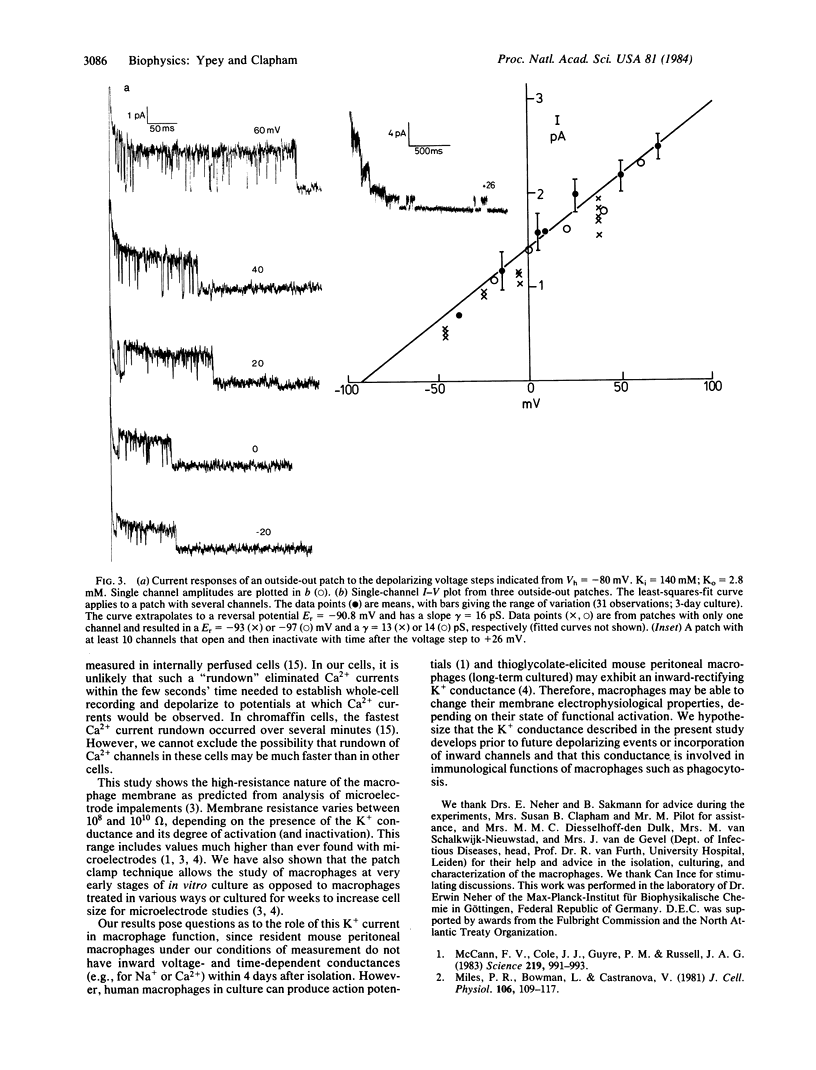
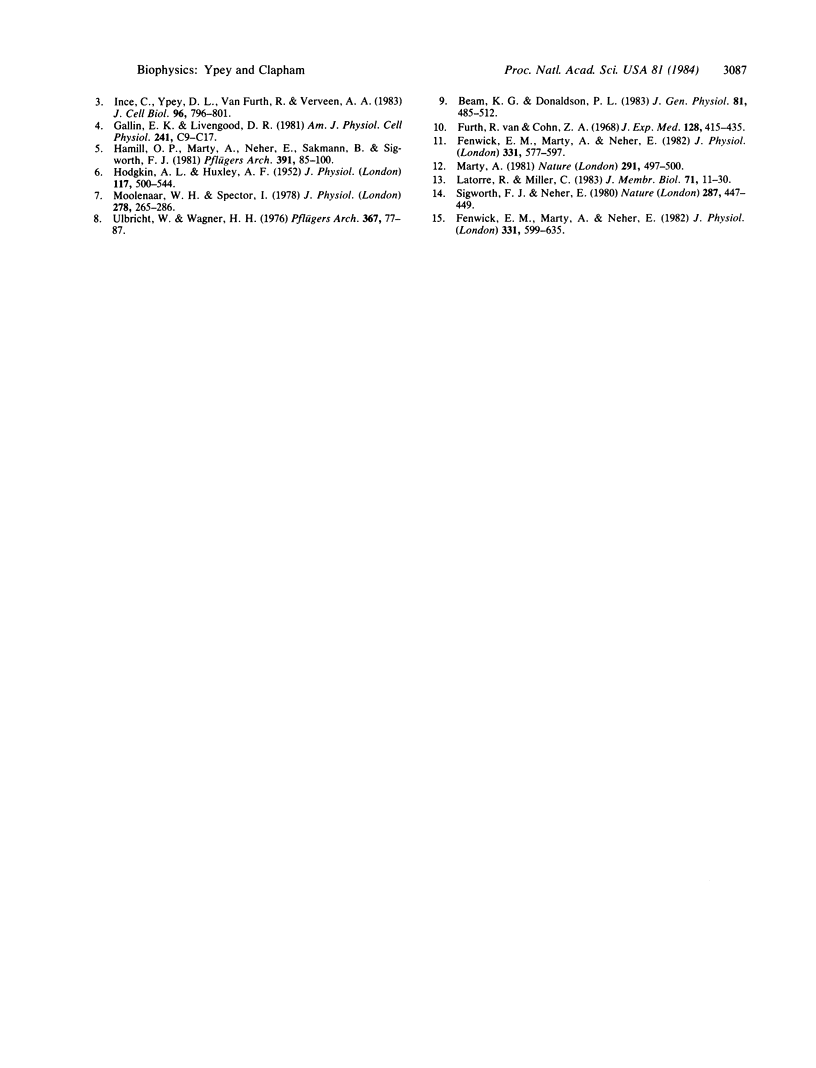
Selected References
These references are in PubMed. This may not be the complete list of references from this article.
- Beam K. G., Donaldson P. L. A quantitative study of potassium channel kinetics in rat skeletal muscle from 1 to 37 degrees C. J Gen Physiol. 1983 Apr;81(4):485–512. doi: 10.1085/jgp.81.4.485. [DOI] [PMC free article] [PubMed] [Google Scholar]
- Fenwick E. M., Marty A., Neher E. A patch-clamp study of bovine chromaffin cells and of their sensitivity to acetylcholine. J Physiol. 1982 Oct;331:577–597. doi: 10.1113/jphysiol.1982.sp014393. [DOI] [PMC free article] [PubMed] [Google Scholar]
- Fenwick E. M., Marty A., Neher E. Sodium and calcium channels in bovine chromaffin cells. J Physiol. 1982 Oct;331:599–635. doi: 10.1113/jphysiol.1982.sp014394. [DOI] [PMC free article] [PubMed] [Google Scholar]
- Gallin E. K., Livengood D. R. Inward rectification in mouse macrophages: evidence for a negative resistance region. Am J Physiol. 1981 Jul;241(1):C9–17. doi: 10.1152/ajpcell.1981.241.1.C9. [DOI] [PubMed] [Google Scholar]
- HODGKIN A. L., HUXLEY A. F. A quantitative description of membrane current and its application to conduction and excitation in nerve. J Physiol. 1952 Aug;117(4):500–544. doi: 10.1113/jphysiol.1952.sp004764. [DOI] [PMC free article] [PubMed] [Google Scholar]
- Hamill O. P., Marty A., Neher E., Sakmann B., Sigworth F. J. Improved patch-clamp techniques for high-resolution current recording from cells and cell-free membrane patches. Pflugers Arch. 1981 Aug;391(2):85–100. doi: 10.1007/BF00656997. [DOI] [PubMed] [Google Scholar]
- Ince C., Ypey D. L., Van Furth R., Verveen A. A. Estimation of the membrane potential of cultured macrophages from the fast potential transient upon microelectrode entry. J Cell Biol. 1983 Mar;96(3):796–801. doi: 10.1083/jcb.96.3.796. [DOI] [PMC free article] [PubMed] [Google Scholar]
- Latorre R., Miller C. Conduction and selectivity in potassium channels. J Membr Biol. 1983;71(1-2):11–30. doi: 10.1007/BF01870671. [DOI] [PubMed] [Google Scholar]
- Marty A. Ca-dependent K channels with large unitary conductance in chromaffin cell membranes. Nature. 1981 Jun 11;291(5815):497–500. doi: 10.1038/291497a0. [DOI] [PubMed] [Google Scholar]
- McCann F. V., Cole J. J., Guyre P. M., Russell J. A. Action potentials in macrophages derived from human monocytes. Science. 1983 Feb 25;219(4587):991–993. doi: 10.1126/science.6823563. [DOI] [PubMed] [Google Scholar]
- Miles P. R., Bowman L., Castranova V. Transmembrane potential changes during phagocytosis in rat alveolar macrophages. J Cell Physiol. 1981 Jan;106(1):109–117. doi: 10.1002/jcp.1041060112. [DOI] [PubMed] [Google Scholar]
- Moolenaar W. H., Spector I. Ionic currents in cultured mouse neuroblastoma cells under voltage-clamp conditions. J Physiol. 1978 May;278:265–286. doi: 10.1113/jphysiol.1978.sp012303. [DOI] [PMC free article] [PubMed] [Google Scholar]
- Sigworth F. J., Neher E. Single Na+ channel currents observed in cultured rat muscle cells. Nature. 1980 Oct 2;287(5781):447–449. doi: 10.1038/287447a0. [DOI] [PubMed] [Google Scholar]
- Ulbricht W., Wagner H. H. Block of potassium channels of the nodal membrane by 4-aminopyridine and its partial removal on depolarization. Pflugers Arch. 1976 Nov 30;367(1):77–87. doi: 10.1007/BF00583659. [DOI] [PubMed] [Google Scholar]
- van Furth R., Cohn Z. A. The origin and kinetics of mononuclear phagocytes. J Exp Med. 1968 Sep 1;128(3):415–435. doi: 10.1084/jem.128.3.415. [DOI] [PMC free article] [PubMed] [Google Scholar]