Abstract
The effects of uncouplers and valinomycin plus nigericin (in the presence of K+) were studied on the apparent Km for substrates and apparent Vmax of the following energy-linked reactions catalyzed by submitochondrial particles: oxidative phosphorylation, NTP-33Pi exchange, ATP-driven electron transfer from succinate to NAD, and respiration-driven transhydrogenation from NADH to 3-acetylpyridine adenine dinucleotide phosphate. In all cases, partially uncoupling (up to 90%) concentrations of uncouplers of valinomycin plus nigericin were found to decrease apparent Vmax and to increase apparent Km. Results plotted as ln (Vmax/Km) versus the concentration of uncouplers or ionophores showed a linear decrease of the former as a function of increasing perturbant concentration (i.e., decreasing free energy). Because Vmax/Km may be considered as a measure of the apparent first-order rate constant for enzyme-substrate interaction and reflects the affinity between enzyme and substrate to form a complex, the results are consistent with the interpretation that membrane energization leads to a change in enzyme conformation with the resultant increase in enzyme-substrate affinity and facilitation of the reaction rate under consideration. The significance of these findings with respect to the mechanism of action of the energy-transducing systems studied is discussed.
Full text
PDF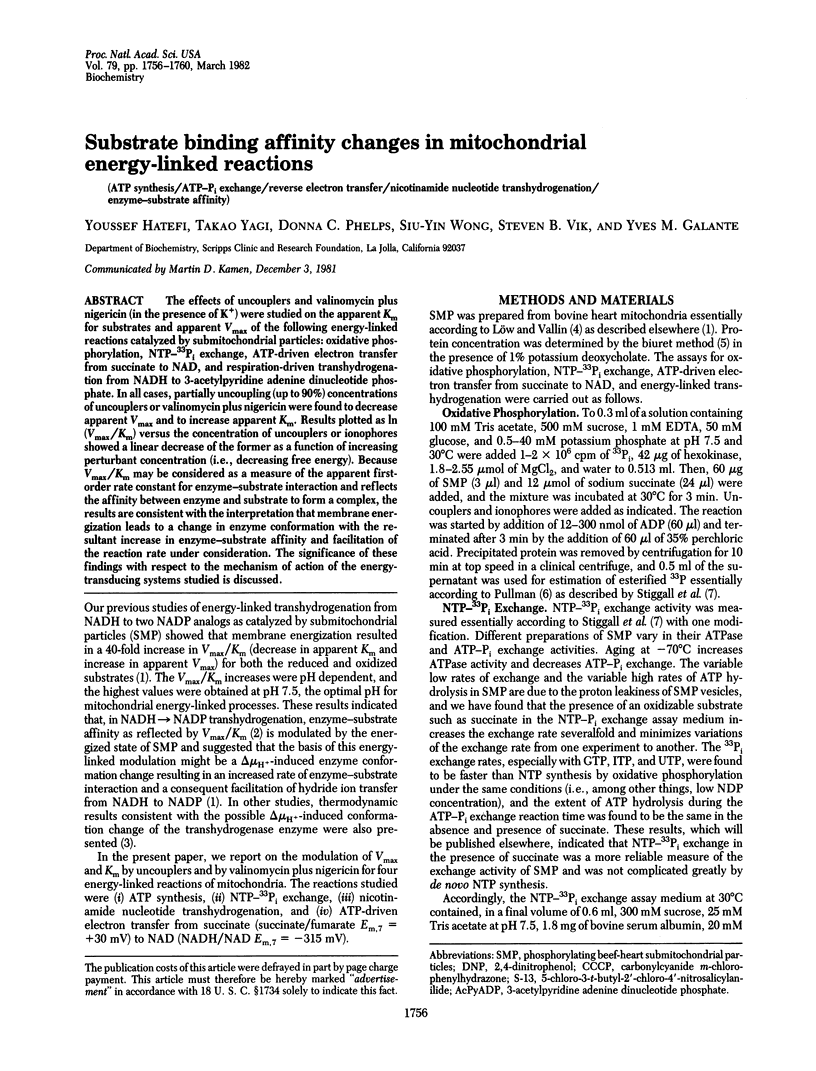
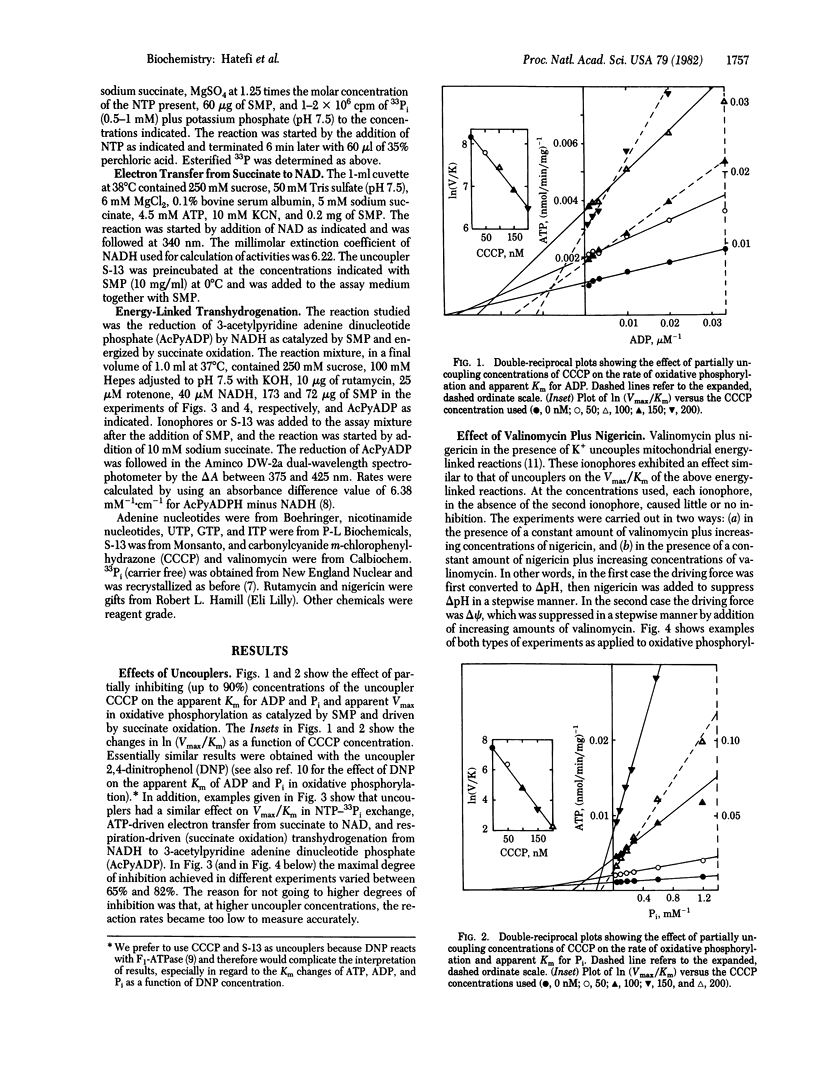
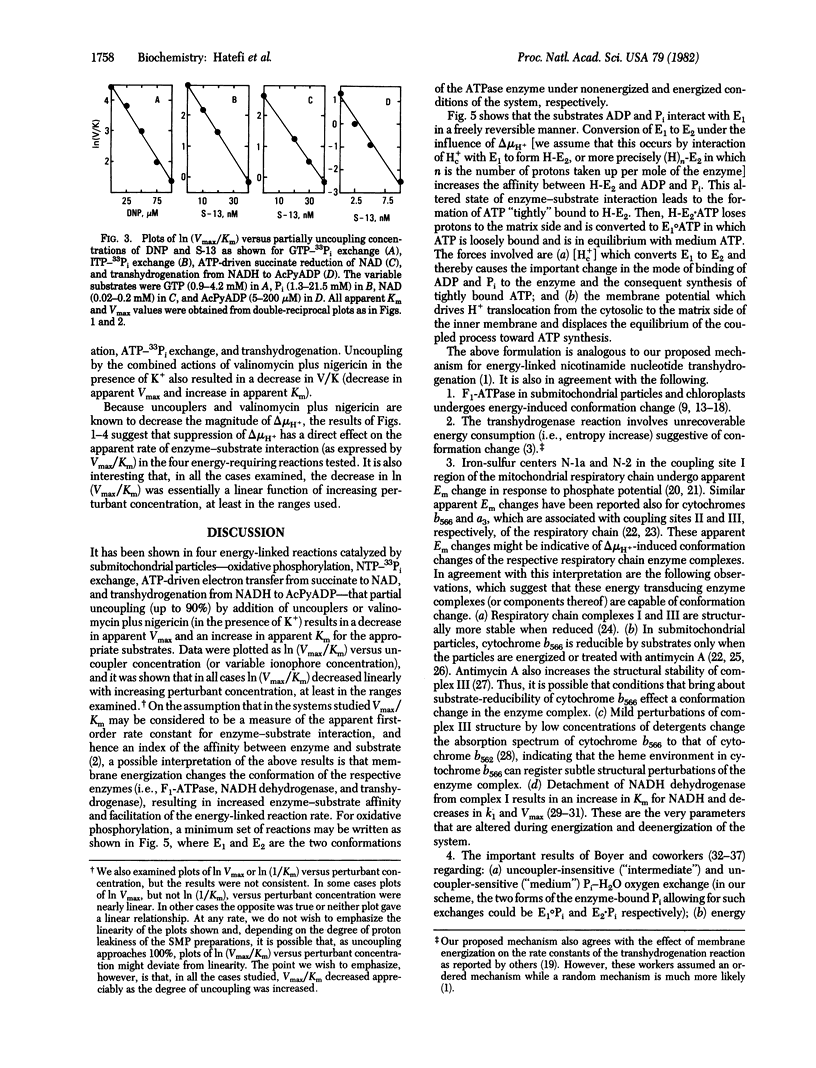
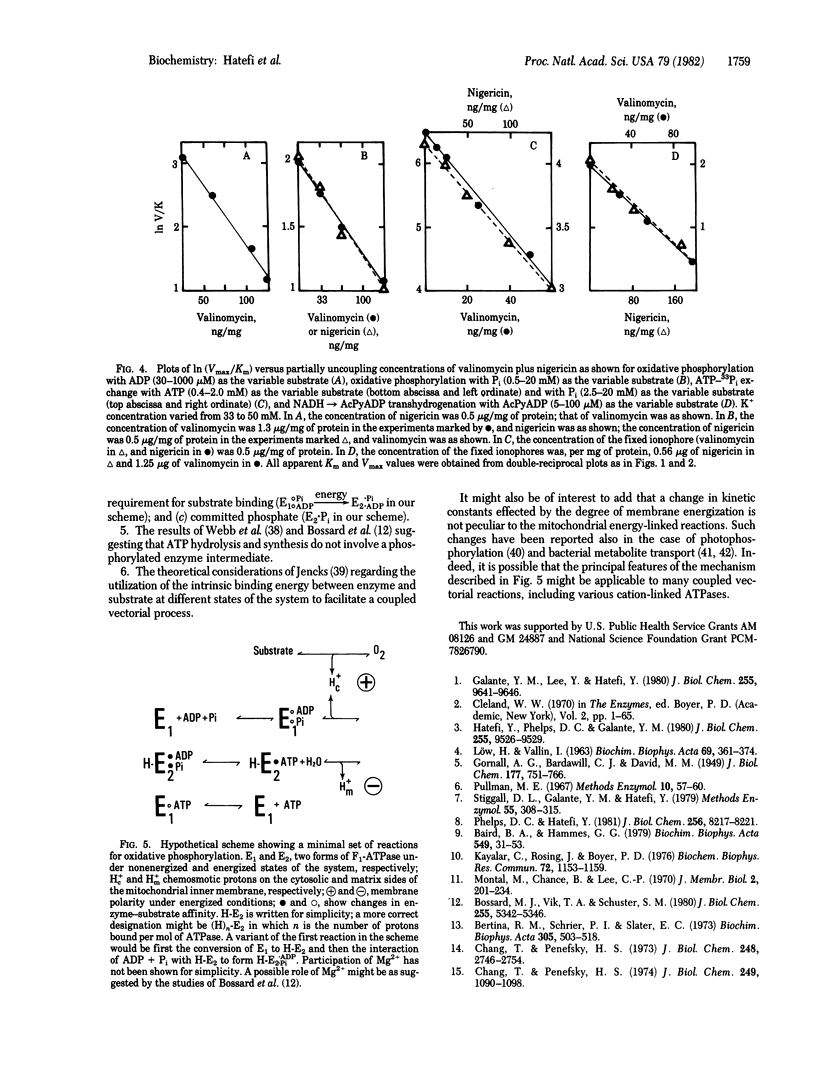
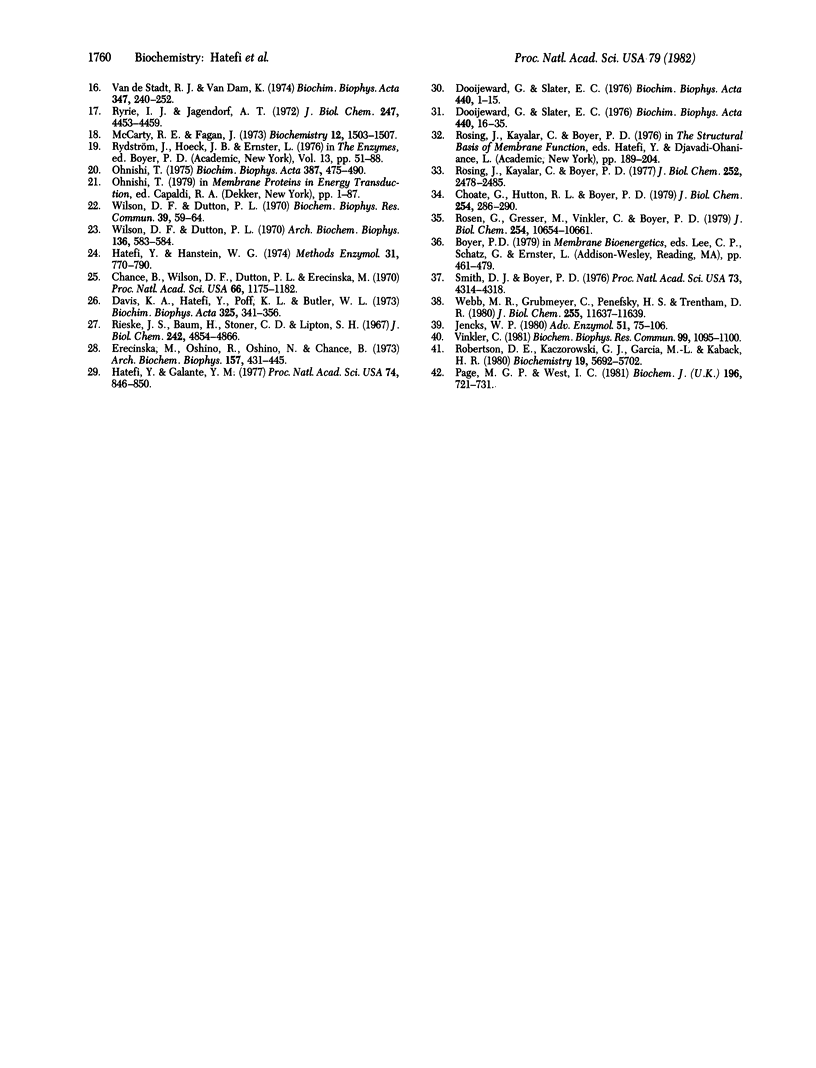
Selected References
These references are in PubMed. This may not be the complete list of references from this article.
- Baird B. A., Hammes G. G. Structure of oxidative- and photo-phosphorylation coupling factor complexes. Biochim Biophys Acta. 1979 Jul 3;549(1):31–53. doi: 10.1016/0304-4173(79)90017-x. [DOI] [PubMed] [Google Scholar]
- Bertina R. M., Schrier P. I., Slater E. C. The binding of aurovertin to mitochondria, and its effect on mitochondrial respiration. Biochim Biophys Acta. 1973 Jun 28;305(3):503–518. doi: 10.1016/0005-2728(73)90072-8. [DOI] [PubMed] [Google Scholar]
- Bossard M. J., Vik T. A., Schuster S. M. Beef heart mitochondrial adenosine triphosphatase-catalyzed formation of a transition state analog in ATP synthesis. J Biol Chem. 1980 Jun 10;255(11):5342–5346. [PubMed] [Google Scholar]
- Chance B., Wilson D. F., Dutton P. L., Erecińska M. Energy-coupling mechanisms in mitochondria: kinetic, spectroscopic, and thermodynamic properties of an energy-transducing form of cytochrome b. Proc Natl Acad Sci U S A. 1970 Aug;66(4):1175–1182. doi: 10.1073/pnas.66.4.1175. [DOI] [PMC free article] [PubMed] [Google Scholar]
- Chang T. M., Penefsky H. S. Energy-dependent enhancement of aurovertin fluorescence. An indicator of conformational changes in beef heart mitochondrial adenosine triphosphatase. J Biol Chem. 1974 Feb 25;249(4):1090–1098. [PubMed] [Google Scholar]
- Chang T., Penefsky H. S. Aurovertin, a fluorescent probe of conformational change in beef heart mitochondrial adenosine triphosphatase. J Biol Chem. 1973 Apr 25;248(8):2746–2754. [PubMed] [Google Scholar]
- Choate G. L., Hutton R. L., Boyer P. D. Occurrence and significance of oxygen exchange reactions catalyzed by mitochondrial adenosine triphosphatase preparations. J Biol Chem. 1979 Jan 25;254(2):286–290. [PubMed] [Google Scholar]
- Davis K. A., Hatefi Y., Poff K. L., Butler W. L. The b-type cytochromes of bovine heart mitochondria: absorption spectra, enzymatic properties, and distribution in the electron transfer complexes. Biochim Biophys Acta. 1973 Dec 14;325(3):341–356. doi: 10.1016/0005-2728(73)90196-5. [DOI] [PubMed] [Google Scholar]
- Dooijewaard G., Slater E. C. Steady-state kinetics of high molecular weight (type-I) NADH dehydrogenase. Biochim Biophys Acta. 1976 Jul 9;440(1):1–15. doi: 10.1016/0005-2728(76)90109-2. [DOI] [PubMed] [Google Scholar]
- Dooijewaard G., Slater E. C. Steady-state kinetics of low molecular weight (type-II) NADH dehydrogenase. Biochim Biophys Acta. 1976 Jul 9;440(1):16–35. doi: 10.1016/0005-2728(76)90110-9. [DOI] [PubMed] [Google Scholar]
- Erecińska M., Oshino R., Oshino N., Chance B. The b cytochromes in succinate--cytochrome c reductase from pigeon breast mitochondria. Arch Biochem Biophys. 1973 Aug;157(2):431–445. doi: 10.1016/0003-9861(73)90659-0. [DOI] [PubMed] [Google Scholar]
- Galante Y. M., Lee Y., Hatefi Y. Effect of pH on the mitochondrial energy-linked and non-energy-linked transhydrogenation reactions. J Biol Chem. 1980 Oct 25;255(20):9641–9646. [PubMed] [Google Scholar]
- Hatefi Y., Galante Y. M. Dehydrogenase and transhydrogenase properties of the soluble NADH dehydrogenase of bovine heart mitochondria. Proc Natl Acad Sci U S A. 1977 Mar;74(3):846–850. doi: 10.1073/pnas.74.3.846. [DOI] [PMC free article] [PubMed] [Google Scholar]
- Hatefi Y., Hanstein W. G. Destabilization of membranes with chaotropic ions. Methods Enzymol. 1974;31:770–790. doi: 10.1016/0076-6879(74)31080-4. [DOI] [PubMed] [Google Scholar]
- Hatefi Y., Phelps D. C., Galante Y. M. Energy-linked transhydrogenation from NADPH to [14C]NADP. J Biol Chem. 1980 Oct 25;255(20):9526–9529. [PubMed] [Google Scholar]
- Jencks W. P. The utilization of binding energy in coupled vectorial processes. Adv Enzymol Relat Areas Mol Biol. 1980;51:75–106. doi: 10.1002/9780470122969.ch2. [DOI] [PubMed] [Google Scholar]
- Kayalar C., Rosing J., Boyer P. D. 2,4-Dinitrophenol causes a marked increase in the apparent Km of Pi and of ADP for oxidative phosphorylation. Biochem Biophys Res Commun. 1976 Oct 4;72(3):1153–1159. doi: 10.1016/s0006-291x(76)80252-5. [DOI] [PubMed] [Google Scholar]
- McCarty R. E., Fagan J. Light-stimulated incorporation of N-ethylmaleimide into coupling factor 1 in spinach chloroplasts. Biochemistry. 1973 Apr 10;12(8):1503–1507. doi: 10.1021/bi00732a006. [DOI] [PubMed] [Google Scholar]
- Ohnishi T. Thermodynamic and EPR characterization of iron-sulfur centers in the NADH-ubiquinone segment of the mitochondrial respiratory chain in pigeon heart. Biochim Biophys Acta. 1975 Jun 17;387(3):475–490. doi: 10.1016/0005-2728(75)90087-0. [DOI] [PubMed] [Google Scholar]
- Page M. G., West I. C. The kinetics of the beta-galactoside-proton symport of Escherichia coli. Biochem J. 1981 Jun 15;196(3):721–731. doi: 10.1042/bj1960721. [DOI] [PMC free article] [PubMed] [Google Scholar]
- Phelps D. C., Hatefi Y. Inhibition of the mitochondrial nicotinamide nucleotide transhydrogenase by dicyclohexylcarbodiimide and diethylpyrocarbonate. J Biol Chem. 1981 Aug 10;256(15):8217–8221. [PubMed] [Google Scholar]
- Rieske J. S., Baum H., Stoner C. D., Lipton S. H. On the antimycin-sensitive cleavage of complex 3 of the mitochondrial respiratory chain. J Biol Chem. 1967 Nov 10;242(21):4854–4866. [PubMed] [Google Scholar]
- Robertson D. E., Kaczorowski G. J., Garcia M. L., Kaback H. R. Active transport in membrane vesicles from Escherichia coli: the electrochemical proton gradient alters the distribution of the lac carrier between two different kinetic states. Biochemistry. 1980 Dec 9;19(25):5692–5702. doi: 10.1021/bi00566a005. [DOI] [PubMed] [Google Scholar]
- Rosen G., Gresser M., Vinkler C., Boyer P. D. Assessment of total catalytic sites and the nature of bound nucleotide participation in photophosphorylation. J Biol Chem. 1979 Nov 10;254(21):10654–10661. [PubMed] [Google Scholar]
- Rosing J., Kayalar C., Boyer P. D. Evidence for energy-dependent change in phosphate binding for mitochondrial oxidative phosphorylation based on measurements of medium and intermediate phosphate-water exchanges. J Biol Chem. 1977 Apr 25;252(8):2478–2485. [PubMed] [Google Scholar]
- Ryrie I. J., Jagendorf A. T. Correlation between a conformational change in the coupling factor protein and the high energy state in chloroplasts. J Biol Chem. 1972 Jul 25;247(14):4453–4459. [PubMed] [Google Scholar]
- Smith D. J., Boyer P. D. Demonstration of a transitory tight binding of ATP and of committed P(i) and ADP during ATP synthesis by chloroplasts. Proc Natl Acad Sci U S A. 1976 Dec;73(12):4314–4318. doi: 10.1073/pnas.73.12.4314. [DOI] [PMC free article] [PubMed] [Google Scholar]
- Stiggall D. L., Galante Y. M., Hatefi Y. Preparation and properties of complex V. Methods Enzymol. 1979;55:308-15, 819-21. doi: 10.1016/0076-6879(79)55036-8. [DOI] [PubMed] [Google Scholar]
- Vinkler C. Opposite modulation by uncoupling and electron transport limitation of the Kmapp of ADP for photophosphorylation. Biochem Biophys Res Commun. 1981 Apr 30;99(4):1095–1100. doi: 10.1016/0006-291x(81)90731-2. [DOI] [PubMed] [Google Scholar]
- Webb M. R., Grubmeyer C., Penefsky H. S., Trentham D. R. The stereochemical course of phosphoric residue transfer catalyzed by beef heart mitochondrial ATPase. J Biol Chem. 1980 Dec 25;255(24):11637–11639. [PubMed] [Google Scholar]
- Wilson D. F., Dutton P. L. Energy dependent changes in the oxidation-reduction potential of cytochrome b. Biochem Biophys Res Commun. 1970 Apr 8;39(1):59–64. doi: 10.1016/0006-291x(70)90757-6. [DOI] [PubMed] [Google Scholar]
- Wilson D. F., Dutton P. L. The oxidation-reduction potentials of cytochromes a and a3 in intact rat liver mitochondria. Arch Biochem Biophys. 1970 Feb;136(2):583–585. doi: 10.1016/0003-9861(70)90233-x. [DOI] [PubMed] [Google Scholar]
- van de Stadt R. J., van Dam K. The equilibrium between the mitochondrial ATPase (F1) and its natural inhibitor in submitochondrial particles. Biochim Biophys Acta. 1974 May 22;347(2):240–252. doi: 10.1016/0005-2728(74)90048-6. [DOI] [PubMed] [Google Scholar]