Abstract
In order to clarify the mechanisms responsible for the observed linkage disequilibrium, such as that found between markers in the major histocompatibility complex of man and mouse, linkage disequilibrium between two linked loci was studied for a finite population with a subdivided structure. The infinite allele model was used. In analogy with the subdivision of the inbreeding coefficient, the linkage disequilibrium coefficient was subdivided, and various variance components of disequilibrium were defined. It was found that the disequilibrium components may get very large when migration is limited if the correlation of alleles at the two loci within a colony is taken relative to that of the entire population. In other words, with limited migration, random genetic drift of gamete types prevails in each colony. A possible test in which the variance components of disequilibrium are compared is suggested; the test discriminates between epistatic natural selection and limited migration, showing the former as the main cause of the observed linkage disequilibrium. It is pointed out that the major histocompatibility complex polymorphism is in accord with the population genetics model of multigene families that incorporate gene conversion or double unequal crossing-over between the loci in the supergene family.
Keywords: subdivision of linkage disequilibrium, supergene, major histocompatibility complex polymorphism
Full text
PDF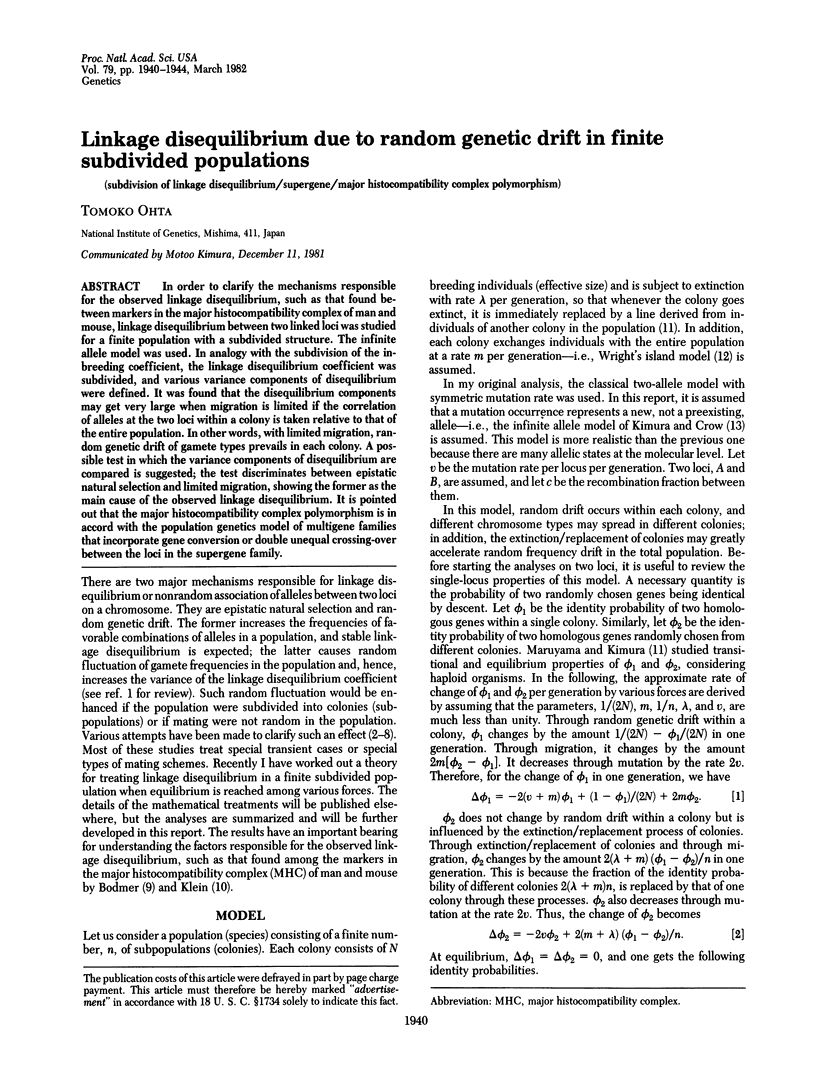
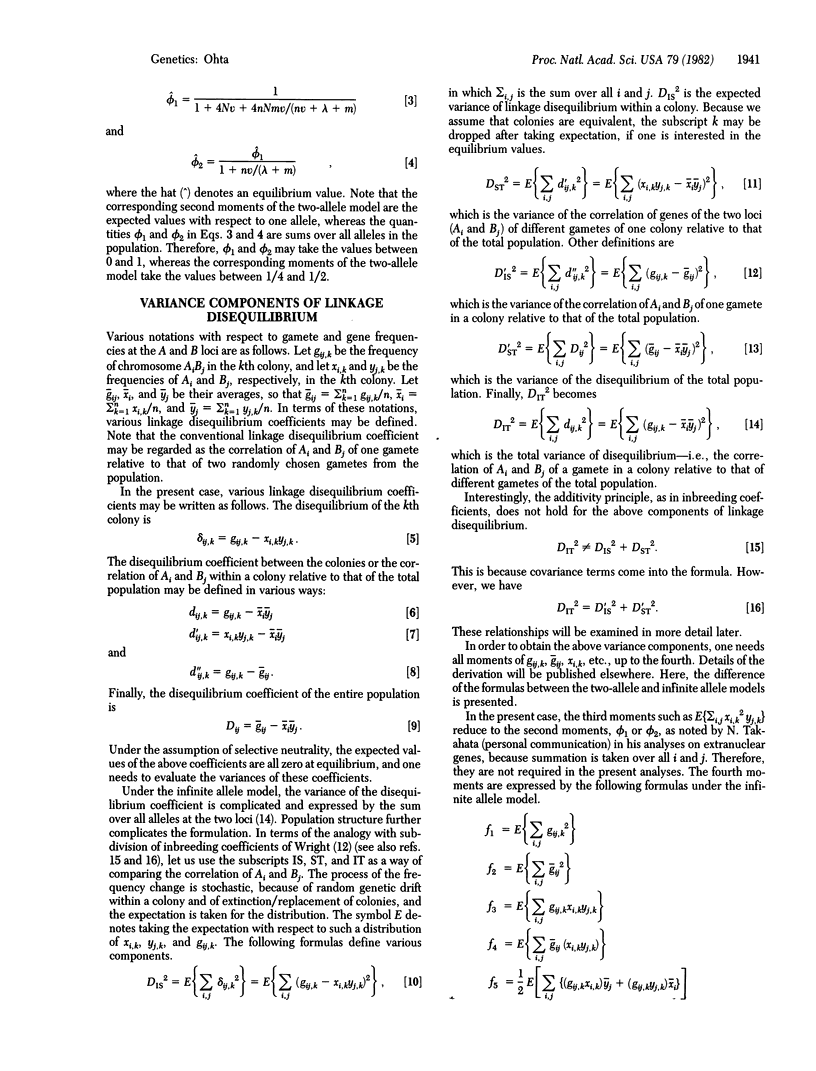
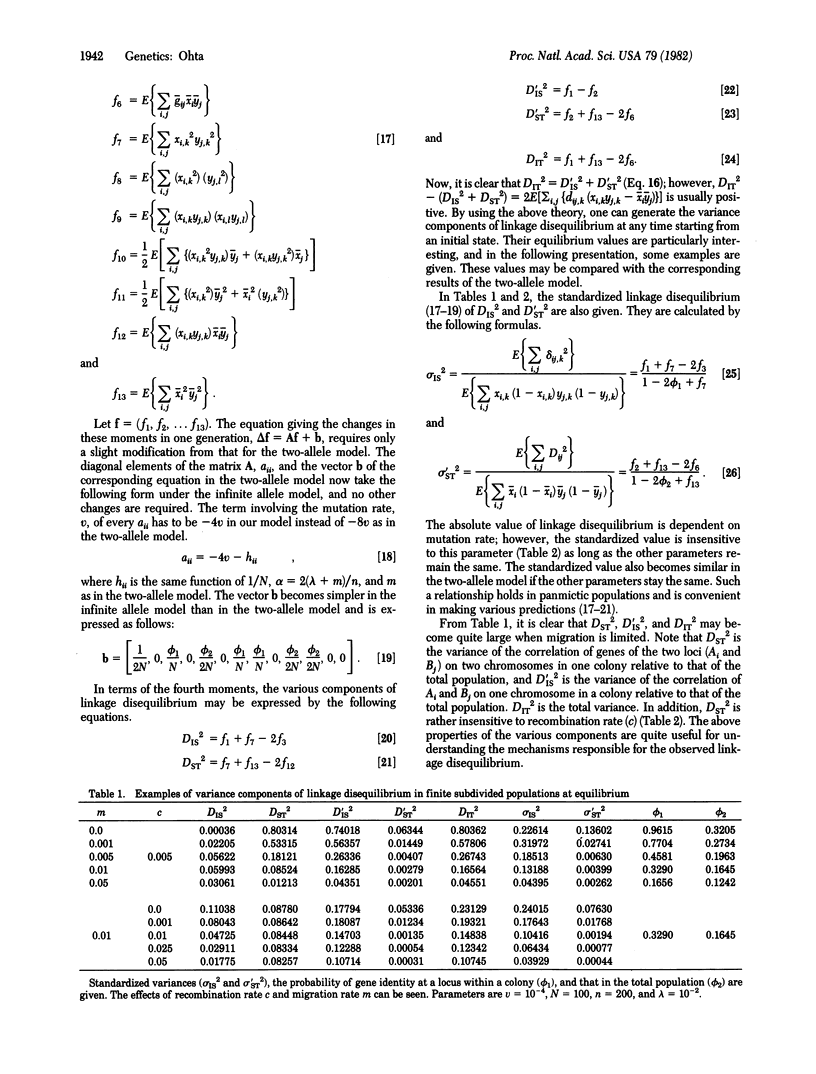
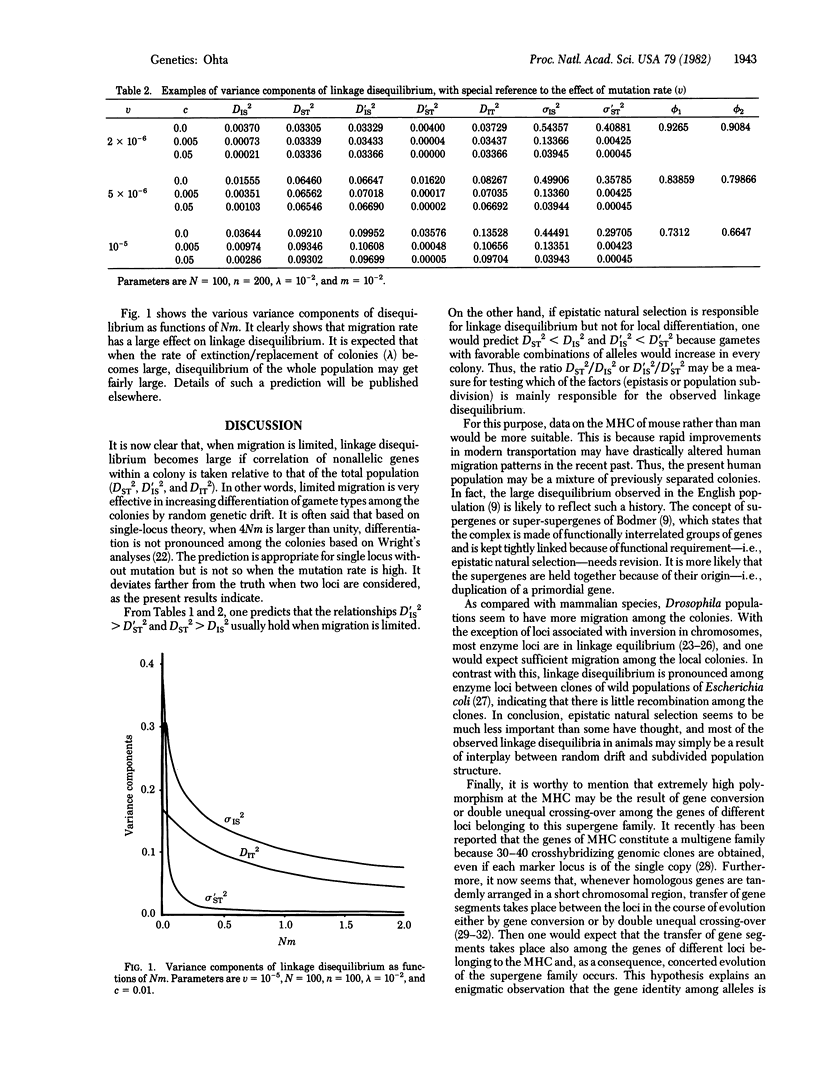
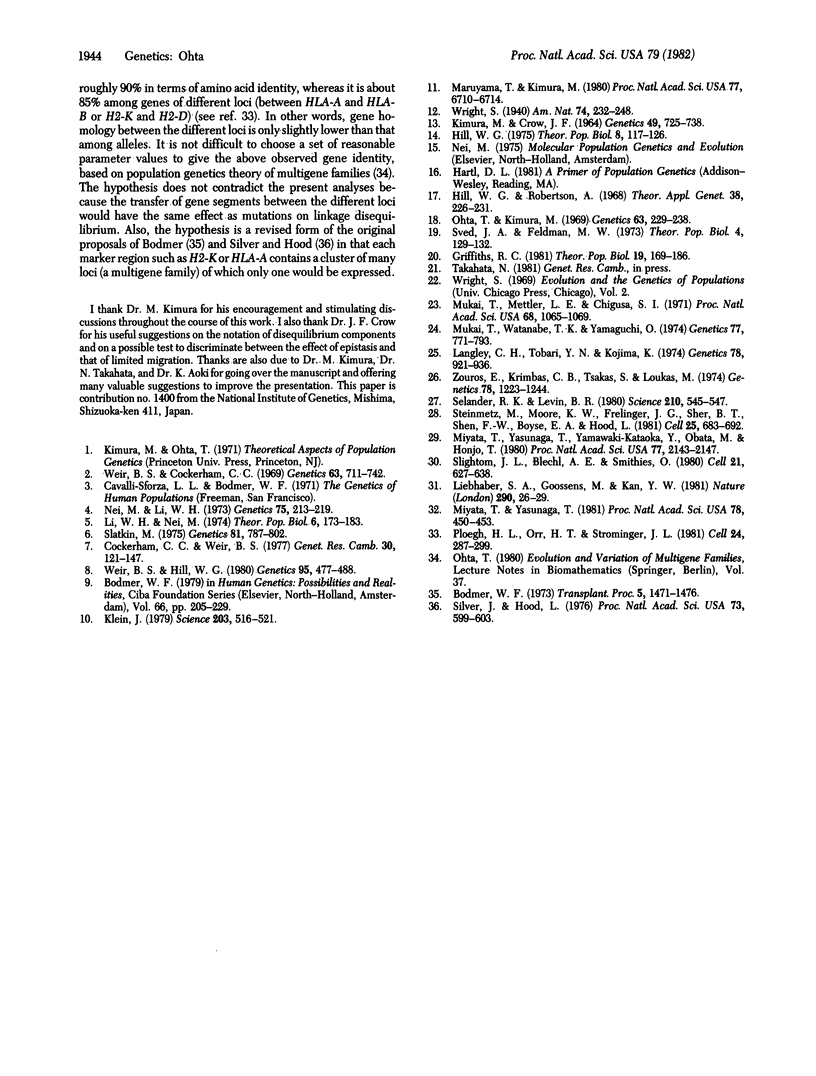
Selected References
These references are in PubMed. This may not be the complete list of references from this article.
- Bodmer W. F. New genetic model for allelism at histocompatibility and other complex loci: polymorphism for control of gene expression. Transplant Proc. 1973 Dec;5(4):1471–1475. [PubMed] [Google Scholar]
- Hill W. G. Linkage disequilibrium among multiple neutral alleles produced by mutation in finite population. Theor Popul Biol. 1975 Oct;8(2):117–126. doi: 10.1016/0040-5809(75)90028-3. [DOI] [PubMed] [Google Scholar]
- KIMURA M., CROW J. F. THE NUMBER OF ALLELES THAT CAN BE MAINTAINED IN A FINITE POPULATION. Genetics. 1964 Apr;49:725–738. doi: 10.1093/genetics/49.4.725. [DOI] [PMC free article] [PubMed] [Google Scholar]
- Klein J. The major histocompatibility complex of the mouse. Science. 1979 Feb 9;203(4380):516–521. doi: 10.1126/science.104386. [DOI] [PubMed] [Google Scholar]
- Langley C. H., Tobari Y. N., Kojima K. I. Linkage disequilibrium in natural populations of Drosophila melanogaster. Genetics. 1974 Nov;78(3):921–936. doi: 10.1093/genetics/78.3.921. [DOI] [PMC free article] [PubMed] [Google Scholar]
- Li W. H., Nei M. Stable linkage disequilibrium without epistasis in subdivided populations. Theor Popul Biol. 1974 Oct;6(2):173–183. doi: 10.1016/0040-5809(74)90022-7. [DOI] [PubMed] [Google Scholar]
- Liebhaber S. A., Goossens M., Kan Y. W. Homology and concerted evolution at the alpha 1 and alpha 2 loci of human alpha-globin. Nature. 1981 Mar 5;290(5801):26–29. doi: 10.1038/290026a0. [DOI] [PubMed] [Google Scholar]
- Maruyama T., Kimura M. Genetic variability and effective population size when local extinction and recolonization of subpopulations are frequent. Proc Natl Acad Sci U S A. 1980 Nov;77(11):6710–6714. doi: 10.1073/pnas.77.11.6710. [DOI] [PMC free article] [PubMed] [Google Scholar]
- Miyata T., Yasunaga T. Rapidly evolving mouse alpha-globin-related pseudo gene and its evolutionary history. Proc Natl Acad Sci U S A. 1981 Jan;78(1):450–453. doi: 10.1073/pnas.78.1.450. [DOI] [PMC free article] [PubMed] [Google Scholar]
- Miyata T., Yasunaga T., Yamawaki-Kataoka Y., Obata M., Honjo T. Nucleotide sequence divergence of mouse immunoglobulin gamma 1 and gamma 2b chain genes and the hypothesis of intervening sequence-mediated domain transfer. Proc Natl Acad Sci U S A. 1980 Apr;77(4):2143–2147. doi: 10.1073/pnas.77.4.2143. [DOI] [PMC free article] [PubMed] [Google Scholar]
- Mukai T., Mettler L. E., Chigusa S. I. Linkage disequilibrium in a local population of Drosophila melanogaster. Proc Natl Acad Sci U S A. 1971 May;68(5):1065–1069. doi: 10.1073/pnas.68.5.1065. [DOI] [PMC free article] [PubMed] [Google Scholar]
- Muki T., Watanabe T. K., Yamaguchi O. The genetic structure of natural populations of Drosophila melanogaster. XII. Linkage disequilibrium in a large local population. Genetics. 1974 Aug;77(4):771–793. doi: 10.1093/genetics/77.4.771. [DOI] [PMC free article] [PubMed] [Google Scholar]
- Nei M., Li W. H. Linkage disequilibrium in subdivided populations. Genetics. 1973 Sep;75(1):213–219. doi: 10.1093/genetics/75.1.213. [DOI] [PMC free article] [PubMed] [Google Scholar]
- Ohta T., Kimura M. Linkage disequilibrium at steady state determined by random genetic drift and recurrent mutation. Genetics. 1969 Sep;63(1):229–238. doi: 10.1093/genetics/63.1.229. [DOI] [PMC free article] [PubMed] [Google Scholar]
- Ploegh H. L., Orr H. T., Strominger J. L. Major histocompatibility antigens: the human (HLA-A, -B, -C) and murine (H-2K, H-2D) class I molecules. Cell. 1981 May;24(2):287–299. doi: 10.1016/0092-8674(81)90318-4. [DOI] [PubMed] [Google Scholar]
- Selander R. K., Levin B. R. Genetic diversity and structure in Escherichia coli populations. Science. 1980 Oct 31;210(4469):545–547. doi: 10.1126/science.6999623. [DOI] [PubMed] [Google Scholar]
- Silver J., Hood L. Structure and evolution of transplantation antigens: partial amino-acid sequences of H-2K and H-2D alloantigens. Proc Natl Acad Sci U S A. 1976 Feb;73(2):599–603. doi: 10.1073/pnas.73.2.599. [DOI] [PMC free article] [PubMed] [Google Scholar]
- Slatkin M. Gene flow and selection in a two-locus system. Genetics. 1975 Dec;81(4):787–802. doi: 10.1093/genetics/81.4.787. [DOI] [PMC free article] [PubMed] [Google Scholar]
- Slightom J. L., Blechl A. E., Smithies O. Human fetal G gamma- and A gamma-globin genes: complete nucleotide sequences suggest that DNA can be exchanged between these duplicated genes. Cell. 1980 Oct;21(3):627–638. doi: 10.1016/0092-8674(80)90426-2. [DOI] [PubMed] [Google Scholar]
- Steinmetz M., Moore K. W., Frelinger J. G., Sher B. T., Shen F. W., Boyse E. A., Hood L. A pseudogene homologous to mouse transplantation antigens: transplantation antigens are encoded by eight exons that correlate with protein domains. Cell. 1981 Sep;25(3):683–692. doi: 10.1016/0092-8674(81)90175-6. [DOI] [PubMed] [Google Scholar]
- Sved J. A., Feldman M. W. Correlation and probability methods for one and two loci. Theor Popul Biol. 1973 Mar;4(1):129–132. doi: 10.1016/0040-5809(73)90008-7. [DOI] [PubMed] [Google Scholar]
- Weir B. S., Cockerham C. C. Group inbreeding with two linked loci. Genetics. 1969 Nov;63(3):711–742. doi: 10.1093/genetics/63.3.711. [DOI] [PMC free article] [PubMed] [Google Scholar]
- Weir B. S., Hill W. G. Effect of mating structure on variation in linkage disequilibrium. Genetics. 1980 Jun;95(2):477–488. doi: 10.1093/genetics/95.2.477. [DOI] [PMC free article] [PubMed] [Google Scholar]
- Zouros E. Genic versus chromosomal variation in natural populations of Drosophila subobscura. Genetics. 1974 Dec;78(4):1223–1244. doi: 10.1093/genetics/78.4.1223. [DOI] [PMC free article] [PubMed] [Google Scholar]