Abstract
Sodium extrusion by bacteria is generally attributed to secondary antiport of Na+ for H+ energized by the proton circulation. Streptococcus faecalis is an exception, in that sodium expulsion from intact cells requires the generation of ATP but does not depend on the protonmotive force. Unfortunately, studies with everted membrane vesicles failed to reveal the expected sodium pump; instead, the vesicles contained a conventional secondary Na+/H+ antiporter. We report here that everted membrane vesicles prepared in the presence of protease inhibitors retain an ATP-driven sodium transport system. The evidence includes the findings that (i) accumulation of 22Na+ by these vesicles is resistant to reagents that dissipate the protonmotive force but requires ATP and (ii) the vesicles contain a sodium-stimulated ATPase that is distinct from F1F0 ATPase, and whose presence is correlated with sodium transport activity. Sodium movements appear to be electroneutral and are accompanied by movement of H+ in the opposite direction. When membranes are incubated in the absence of protease inhibitors, a secondary Na+/H+ antiport activity emerges, possibly by degradation of the sodium pump. We suggest that S. faecalis expels Na+ by means of an ATP-driven primary transport system that mediates exchange of Na+ for H+. The Na+/H+ antiporter seen in earlier membrane preparation is an artefact of proteolytic degradation.
Full text
PDF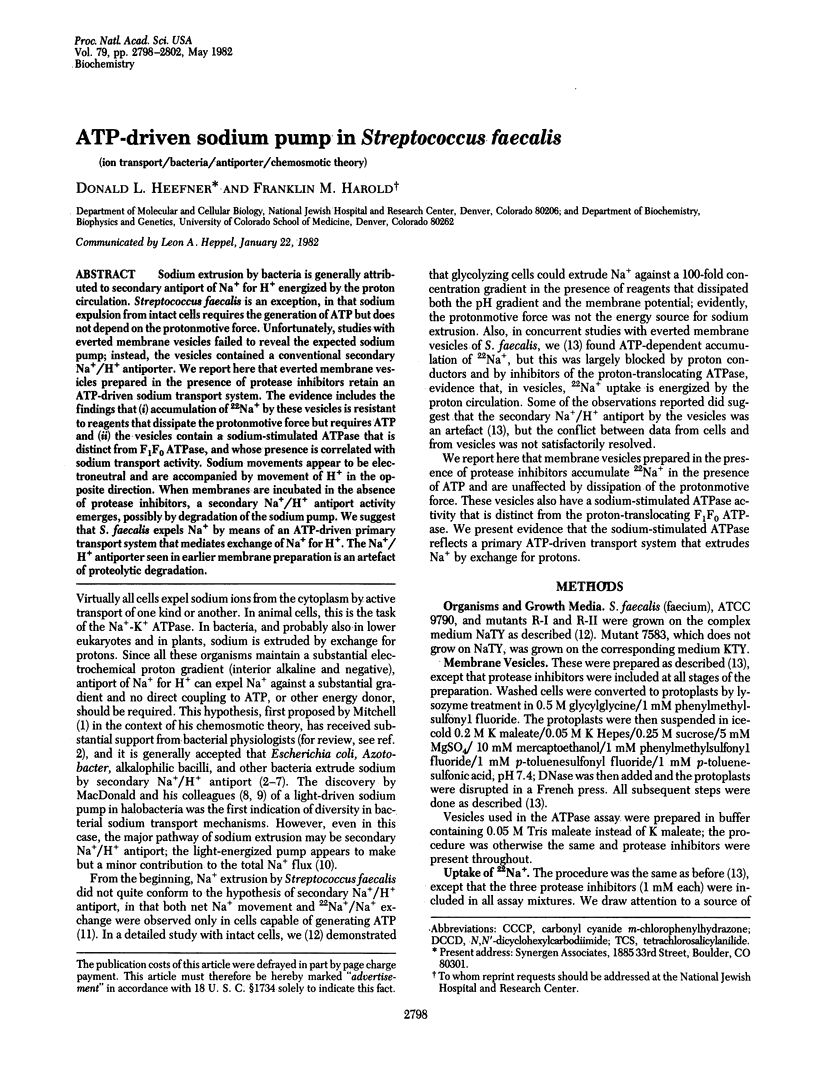
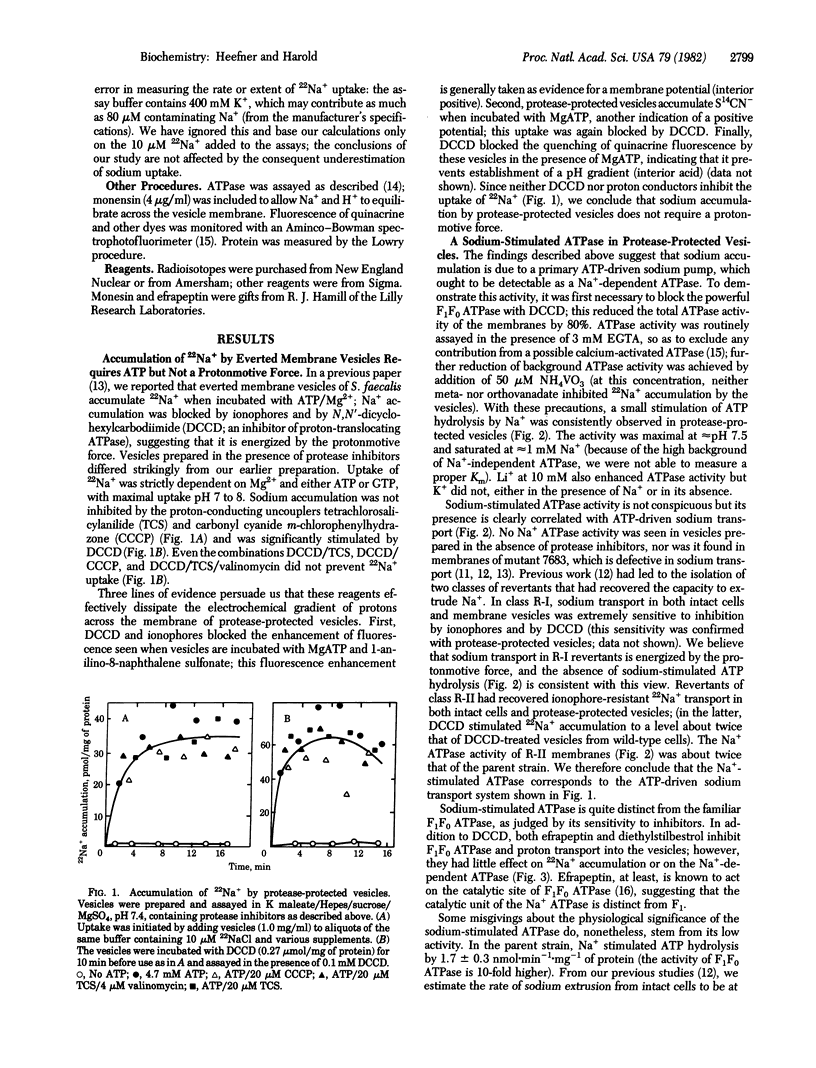
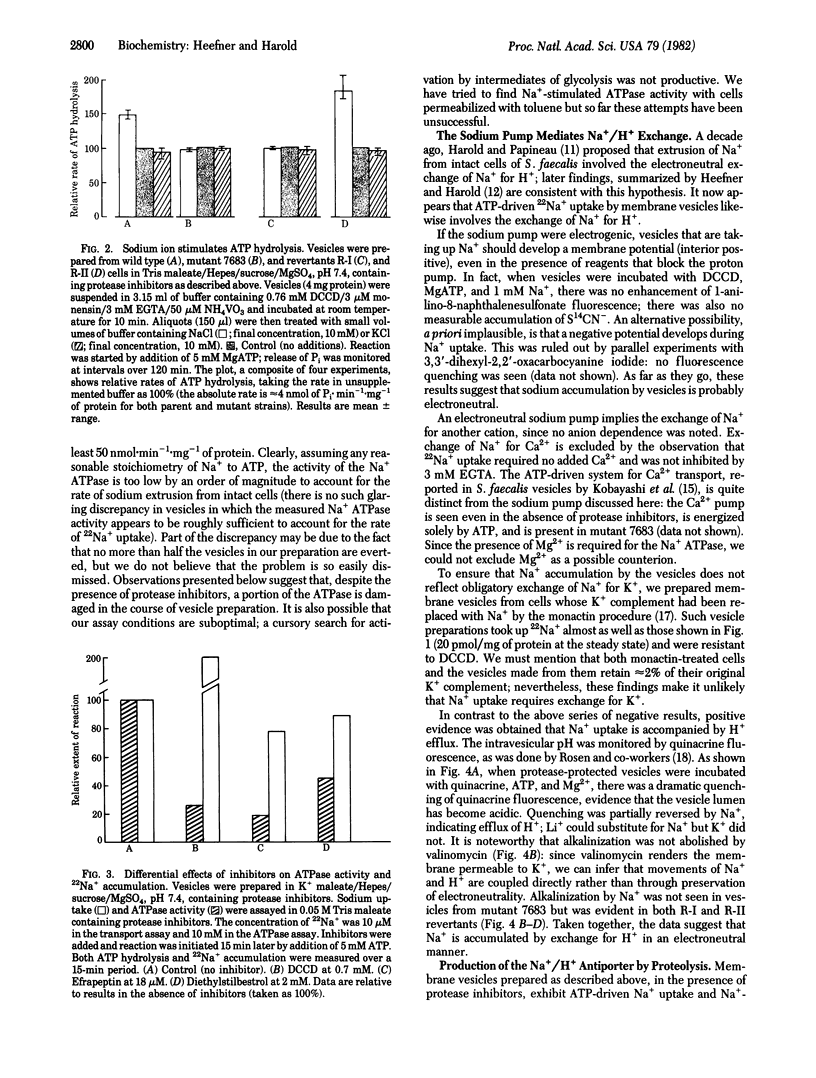
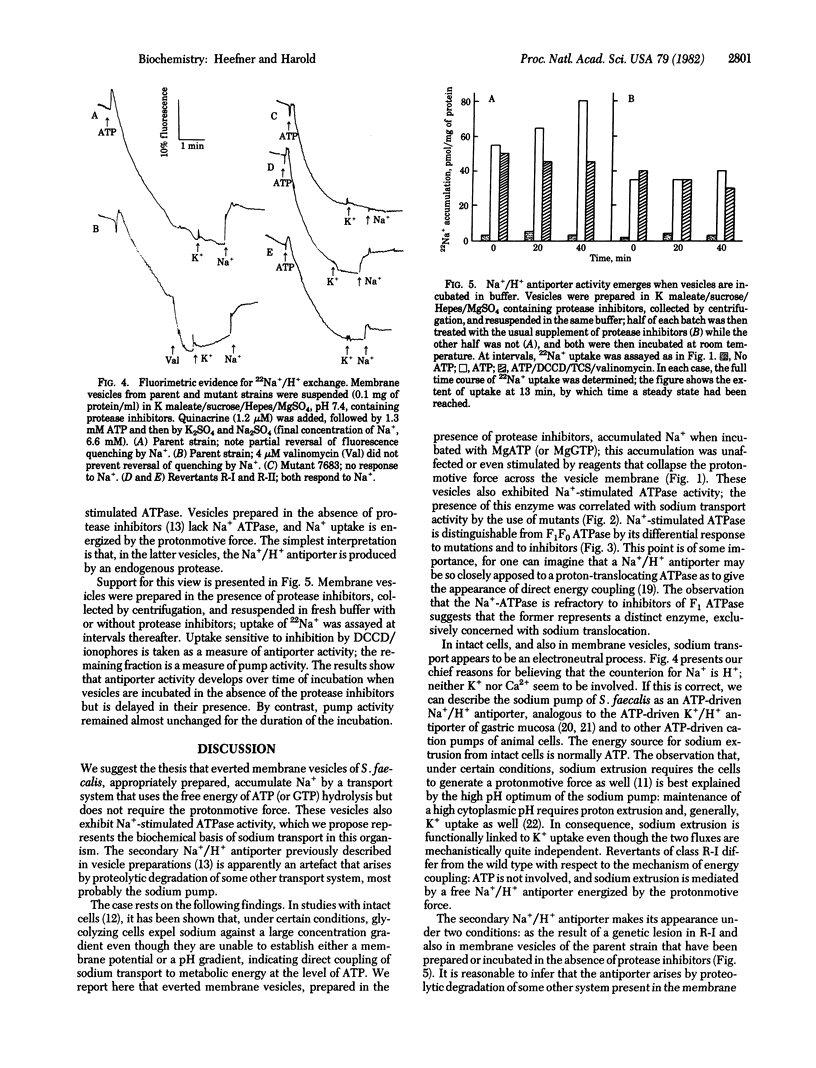
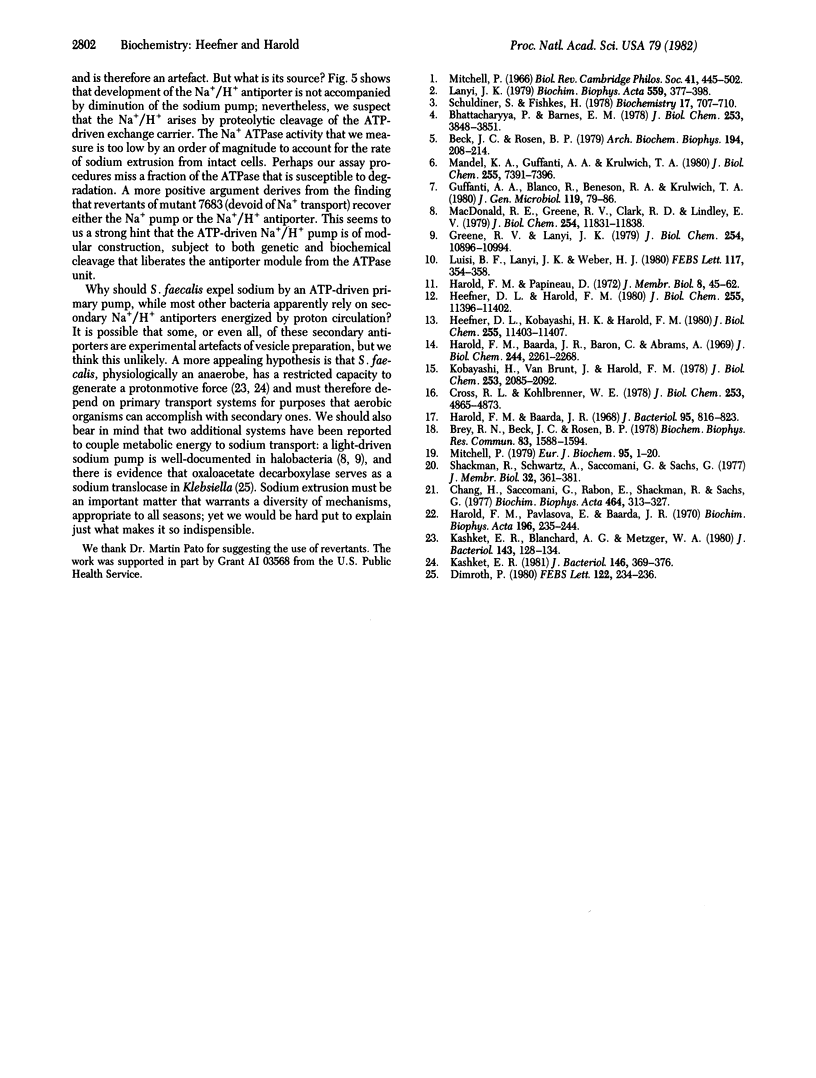
Images in this article
Selected References
These references are in PubMed. This may not be the complete list of references from this article.
- Beck J. C., Rosen B. P. Cation/proton antiport systems in escherichia coli: properties of the sodium/proton antiporter. Arch Biochem Biophys. 1979 Apr 15;194(1):208–214. doi: 10.1016/0003-9861(79)90611-8. [DOI] [PubMed] [Google Scholar]
- Bhattacharyya P., Barnes E. M., Jr Proton-coupled sodium uptake by membrane vesicles from Azotobacter vinelandii. J Biol Chem. 1978 Jun 10;253(11):3848–3851. [PubMed] [Google Scholar]
- Brey R. N., Beck J. C., Rosen B. P. Cation/proton antiport systems in Escherichia coli. Biochem Biophys Res Commun. 1978 Aug 29;83(4):1588–1594. doi: 10.1016/0006-291x(78)91403-1. [DOI] [PubMed] [Google Scholar]
- Chang H., Saccomani G., Rabon E., Schackmann R., Sachs G. Proton transport by gastric membrane vesicles. Biochim Biophys Acta. 1977 Jan 21;464(2):313–327. doi: 10.1016/0005-2736(77)90006-2. [DOI] [PubMed] [Google Scholar]
- Cross R. L., Kohlbrenner W. E. The mode of inhibition of oxidative phosphorylation by efrapeptin (A23871). Evidence for an alternating site mechanism for ATP synthesis. J Biol Chem. 1978 Jul 25;253(14):4865–4873. [PubMed] [Google Scholar]
- Dimroth P. A new sodium-transport system energized by the decarboxylation of oxaloacetate. FEBS Lett. 1980 Dec 29;122(2):234–236. doi: 10.1016/0014-5793(80)80446-7. [DOI] [PubMed] [Google Scholar]
- Greene R. V., Lanyi J. K. Proton movements in response to a light-driven electrogenic pump for sodium ions in Halobacterium halobium membranes. J Biol Chem. 1979 Nov 10;254(21):10986–10994. [PubMed] [Google Scholar]
- Harold F. M., Baarda J. R., Baron C., Abrams A. Inhibition of membrane-bound adenosine triphosphatase and of cation transport in Streptococcus faecalis by N,N'-dicyclohexylcarbodiimide. J Biol Chem. 1969 May 10;244(9):2261–2268. [PubMed] [Google Scholar]
- Harold F. M., Baarda J. R. Effects of nigericin and monactin on cation permeability of Streptococcus faecalis and metabolic capacities of potassium-depleted cells. J Bacteriol. 1968 Mar;95(3):816–823. doi: 10.1128/jb.95.3.816-823.1968. [DOI] [PMC free article] [PubMed] [Google Scholar]
- Harold F. M., Papineau D. Cation transport and electrogenesis by Streptococcus faecalis. II. Proton and sodium extrusion. J Membr Biol. 1972;8(1):45–62. doi: 10.1007/BF01868094. [DOI] [PubMed] [Google Scholar]
- Harold F. M., Pavlasová E., Baarda J. R. A transmembrane pH gradient in Streptococcus faecalis: origin, and dissipation by proton conductors and N,N'-dicyclohexylcarbodimide. Biochim Biophys Acta. 1970;196(2):235–244. doi: 10.1016/0005-2736(70)90011-8. [DOI] [PubMed] [Google Scholar]
- Heefner D. L., Harold F. M. ATP-linked sodium transport in Streptococcus faecalis. I. The sodium circulation. J Biol Chem. 1980 Dec 10;255(23):11396–11402. [PubMed] [Google Scholar]
- Heefner D. L., Kobayashi H., Harold F. M. ATP-linked sodium transport in Streptococcus faecalis. II. Energy coupling in everted membrane vesicles. J Biol Chem. 1980 Dec 10;255(23):11403–11407. [PubMed] [Google Scholar]
- Kashket E. R., Blanchard A. G., Metzger W. C. Proton motive force during growth of Streptococcus lactis cells. J Bacteriol. 1980 Jul;143(1):128–134. doi: 10.1128/jb.143.1.128-134.1980. [DOI] [PMC free article] [PubMed] [Google Scholar]
- Kashket E. R. Proton motive force in growing Streptococcus lactis and Staphylococcus aureus cells under aerobic and anaerobic conditions. J Bacteriol. 1981 Apr;146(1):369–376. doi: 10.1128/jb.146.1.369-376.1981. [DOI] [PMC free article] [PubMed] [Google Scholar]
- Kobayashi H., Van Brunt J., Harold F. M. ATP-linked calcium transport in cells and membrane vesicles of Streptococcus faecalis. J Biol Chem. 1978 Apr 10;253(7):2085–2092. [PubMed] [Google Scholar]
- Lanyi J. K. The role of Na+ in transport processes of bacterial membranes. Biochim Biophys Acta. 1979 Dec 20;559(4):377–397. doi: 10.1016/0304-4157(79)90011-x. [DOI] [PubMed] [Google Scholar]
- Luisi B. F., Lanyi J. K., Weber H. J. Na+ transport via Na+/H+ antiport in Halobacterium halobium envelope vesicles. FEBS Lett. 1980 Aug 11;117(1):354–358. doi: 10.1016/0014-5793(80)80979-3. [DOI] [PubMed] [Google Scholar]
- MacDonald R. E., Greene R. V., Clark R. D., Lindley E. V. Characterization of the light-driven sodium pump of Halobacterium halobium. Consequences of sodium efflux as the primary light-driven event. J Biol Chem. 1979 Dec 10;254(23):11831–11838. [PubMed] [Google Scholar]
- Mandel K. G., Guffanti A. A., Krulwich T. A. Monovalent cation/proton antiporters in membrane vesicles from Bacillus alcalophilus. J Biol Chem. 1980 Aug 10;255(15):7391–7396. [PubMed] [Google Scholar]
- Mitchell P. Chemiosmotic coupling in oxidative and photosynthetic phosphorylation. Biol Rev Camb Philos Soc. 1966 Aug;41(3):445–502. doi: 10.1111/j.1469-185x.1966.tb01501.x. [DOI] [PubMed] [Google Scholar]
- Mitchell P. The Ninth Sir Hans Krebs Lecture. Compartmentation and communication in living systems. Ligand conduction: a general catalytic principle in chemical, osmotic and chemiosmotic reaction systems. Eur J Biochem. 1979 Mar 15;95(1):1–20. doi: 10.1111/j.1432-1033.1979.tb12934.x. [DOI] [PubMed] [Google Scholar]
- Schackmann R., Schwartz A., Saccomani G., Sachs G. Cation transport by gastric H+:K+ ATPase. J Membr Biol. 1977 Apr 22;32(3-4):361–381. doi: 10.1007/BF01905228. [DOI] [PubMed] [Google Scholar]