Abstract
Two models of protein-folding pathways are proposed on the basis of equilibrium and kinetic data in the literature. One is a growth-type model--i.e., nucleation of the native-like structure occurs in the folding process, in the rate-limiting step(s), and subsequent folding around the nucleation sites proceeds smoothly to form the native disulfide bonds and conformation. The other is a rearrangement-type model--i.e., proper nucleation does not occur in the folding process; instead, non-native interactions play a significant role in the folding pathways and lead to metastable intermediate species. Such non-native interactions, including incorrect disulfide bonds and proline cis-trans isomerization, must be disrupted or rearranged to nucleate the native interactions [a process that is included in the rate-limiting step(s)] for the protein to fold. The rate-limiting steps in the pathways for regeneration of RNase A from the reduced protein are classified as growth- or rearrangement-type pathways. The growth-type pathway is the one accompanying the formation of an intramolecular disulfide bond in the rate-limiting step. The rearrangement-type pathway is the one accompanying the reshuffling or disruption of a disulfide bond in the rate-limiting step. The folding of other proteins, accompanying oxidation of the reduced form, and the folding of denatured proteins with intact disulfide bonds are discussed in terms of the growth- and rearrangement-type models.
Full text
PDF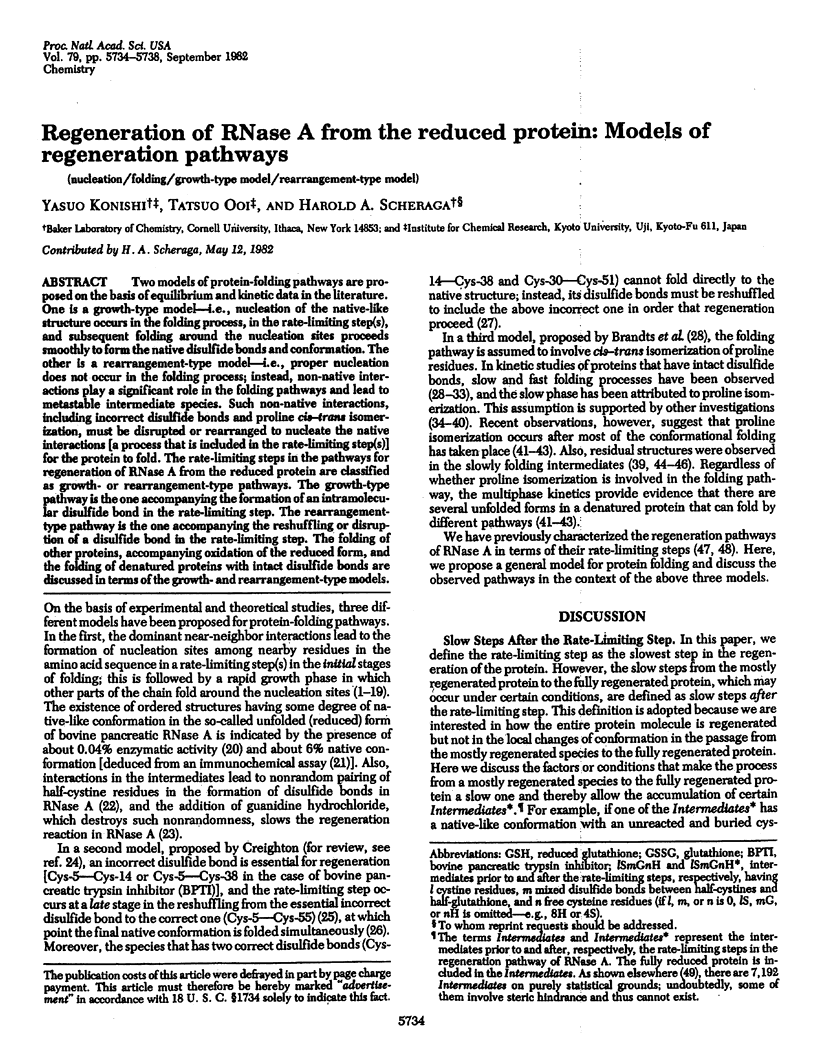
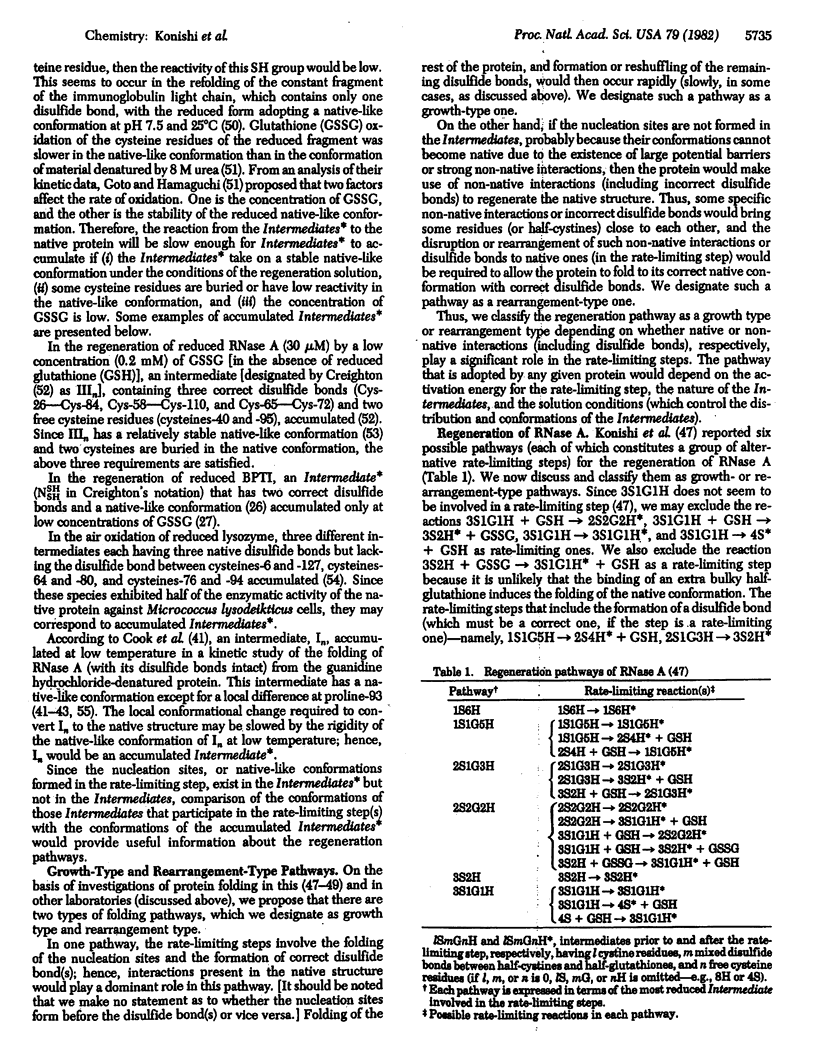
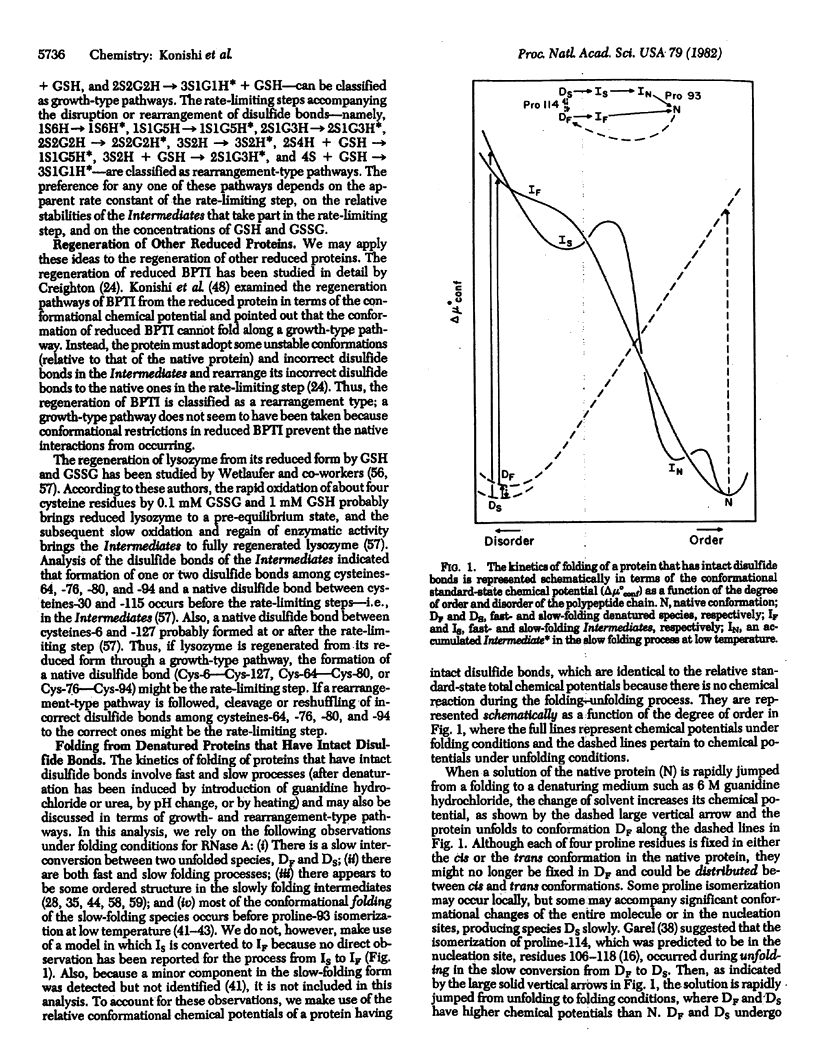
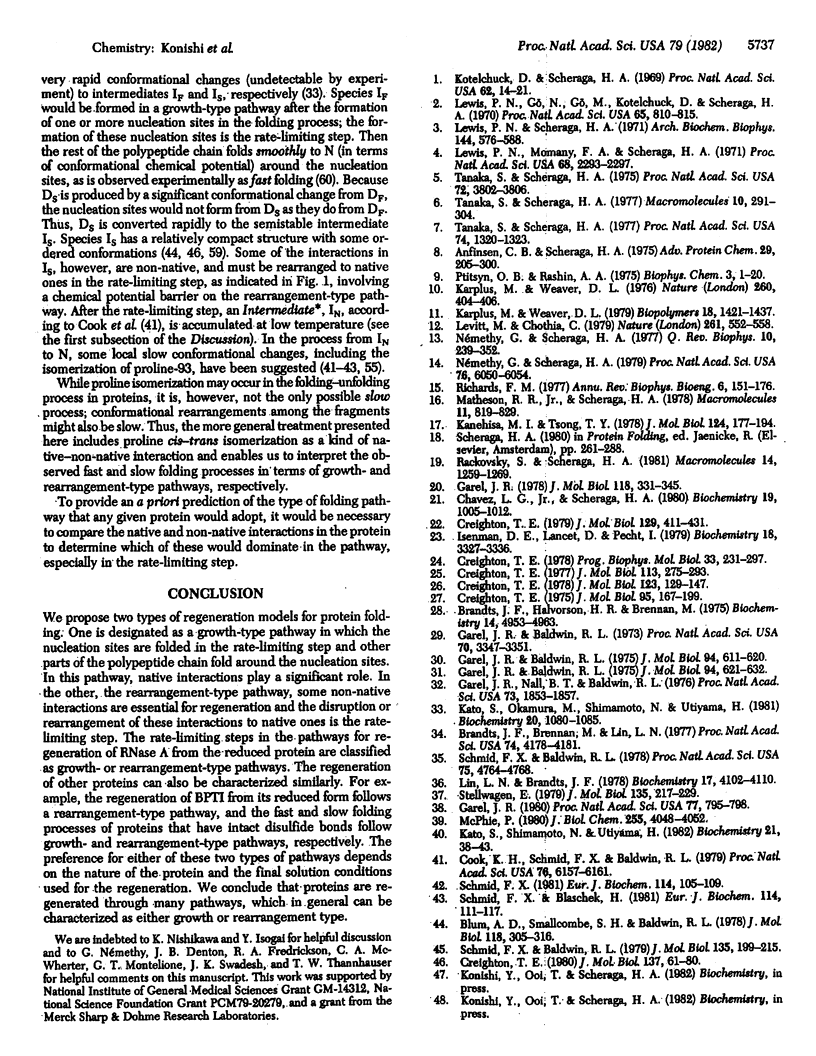
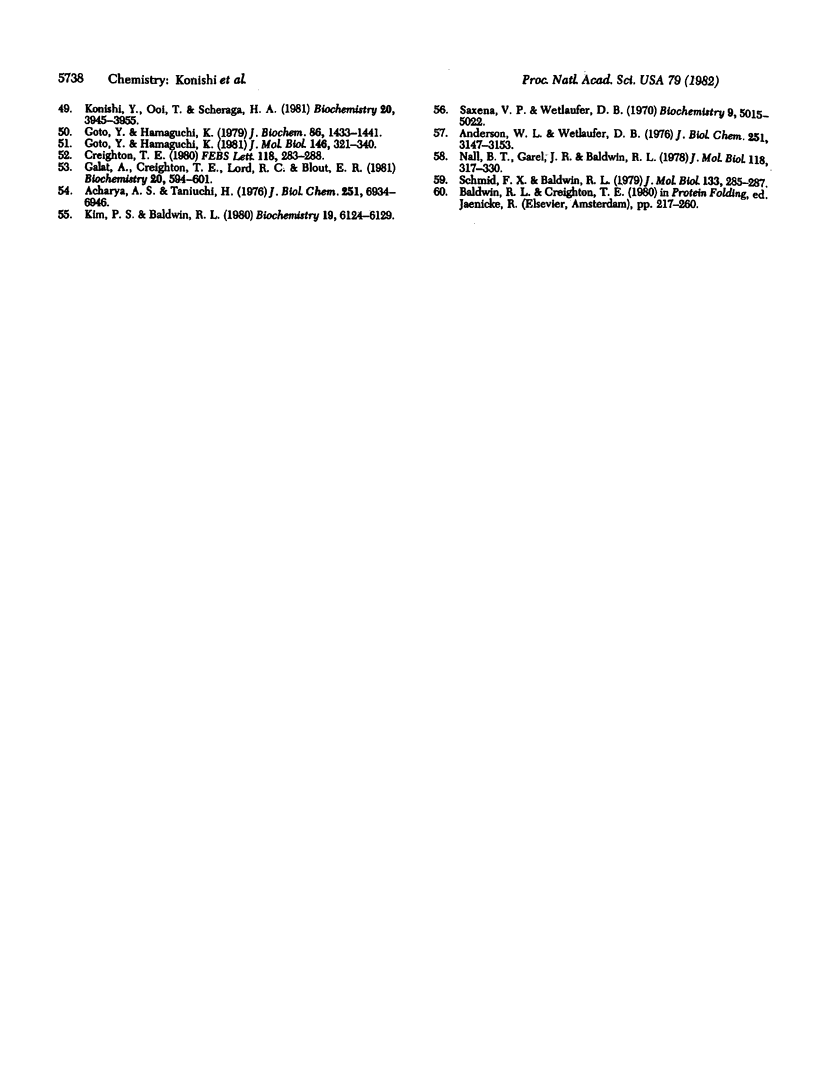
Selected References
These references are in PubMed. This may not be the complete list of references from this article.
- Acharya A. S., Taniuchi H. A study of renaturation of reduced hen egg white lysozyme. Enzymically active intermediates formed during oxidation of the reduced protein. J Biol Chem. 1976 Nov 25;251(22):6934–6946. [PubMed] [Google Scholar]
- Al-Sarraf M., Amer M. H., Vaishampayan G., Loh J., Weaver A. A multidisciplinary therapeutic approach for advanced previously untreated epidermoid cancer of the head and neck: preliminary report. Int J Radiat Oncol Biol Phys. 1979 Aug;5(8):1421–1423. doi: 10.1016/0360-3016(79)90682-5. [DOI] [PubMed] [Google Scholar]
- Anderson W. L., Wetlaufer D. B. The folding pathway of reduced lysozyme. J Biol Chem. 1976 May 25;251(10):3147–3153. [PubMed] [Google Scholar]
- Anfinsen C. B., Scheraga H. A. Experimental and theoretical aspects of protein folding. Adv Protein Chem. 1975;29:205–300. doi: 10.1016/s0065-3233(08)60413-1. [DOI] [PubMed] [Google Scholar]
- Blum A. D., Smallcombe S. H., Baldwin R. L. Nuclear magnetic resonance evidence for a structural intermediate at an early stage in the refolding of ribonuclease A. J Mol Biol. 1978 Jan 25;118(3):305–316. doi: 10.1016/0022-2836(78)90230-9. [DOI] [PubMed] [Google Scholar]
- Brandts J. F., Halvorson H. R., Brennan M. Consideration of the Possibility that the slow step in protein denaturation reactions is due to cis-trans isomerism of proline residues. Biochemistry. 1975 Nov 4;14(22):4953–4963. doi: 10.1021/bi00693a026. [DOI] [PubMed] [Google Scholar]
- Chavez L. G., Jr, Scheraga H. A. Intrinsic stabilities of portions of the ribonuclease molecule. Biochemistry. 1980 Mar 4;19(5):1005–1012. doi: 10.1021/bi00546a027. [DOI] [PubMed] [Google Scholar]
- Cook K. H., Schmid F. X., Baldwin R. L. Role of proline isomerization in folding of ribonuclease A at low temperatures. Proc Natl Acad Sci U S A. 1979 Dec;76(12):6157–6161. doi: 10.1073/pnas.76.12.6157. [DOI] [PMC free article] [PubMed] [Google Scholar]
- Creighton T. E. A three-disulphide intermediate in refolding of reduced ribonuclease A with a folded conformation. FEBS Lett. 1980 Sep 8;118(2):283–288. doi: 10.1016/0014-5793(80)80239-0. [DOI] [PubMed] [Google Scholar]
- Creighton T. E. Conformational restrictions on the pathway of folding and unfolding of the pancreatic trypsin inhibitor. J Mol Biol. 1977 Jun 25;113(2):275–293. doi: 10.1016/0022-2836(77)90142-5. [DOI] [PubMed] [Google Scholar]
- Creighton T. E. Intermediates in the refolding of reduced ribonuclease A. J Mol Biol. 1979 Apr 15;129(3):411–431. doi: 10.1016/0022-2836(79)90504-7. [DOI] [PubMed] [Google Scholar]
- Creighton T. E., Kalef E., Arnon R. Immunochemical analysis of the conformational properties of intermediates trapped in the folding and unfolding of bovine pancreatic trypsin inhibitor. J Mol Biol. 1978 Aug 5;123(2):129–147. doi: 10.1016/0022-2836(78)90317-0. [DOI] [PubMed] [Google Scholar]
- Creighton T. E. Kinetic study of protein unfolding and refolding using urea gradient electrophoresis. J Mol Biol. 1980 Feb 15;137(1):61–80. doi: 10.1016/0022-2836(80)90157-6. [DOI] [PubMed] [Google Scholar]
- Galat A., Creighton T. E., Lord R. C., Blout E. R. Circular dichroism, Raman spectroscopy, and gel filtration of trapped folding intermediates of ribonuclease. Biochemistry. 1981 Feb 3;20(3):594–601. doi: 10.1021/bi00506a023. [DOI] [PubMed] [Google Scholar]
- Garel J. R., Baldwin R. L. A physical difference between the fast- and slow-refolding forms of nitrotyrosyl ribonuclease A: the pK values of the nitrotyrosyl groups. J Mol Biol. 1975 Jun 5;94(4):621–632. doi: 10.1016/0022-2836(75)90326-5. [DOI] [PubMed] [Google Scholar]
- Garel J. R., Baldwin R. L. Both the fast and slow refolding reactions of ribonuclease A yield native enzyme. Proc Natl Acad Sci U S A. 1973 Dec;70(12):3347–3351. doi: 10.1073/pnas.70.12.3347. [DOI] [PMC free article] [PubMed] [Google Scholar]
- Garel J. R., Baldwin R. L. The heat-unfolded state of ribonuclease A is an equilibrium mixture of fast and slow refolding species. J Mol Biol. 1975 Jun 5;94(4):611–620. doi: 10.1016/0022-2836(75)90325-3. [DOI] [PubMed] [Google Scholar]
- Garel J. R. Evidence for involvement of proline cis-trans isomerization in the slow unfolding reaction of RNase A. Proc Natl Acad Sci U S A. 1980 Feb;77(2):795–798. doi: 10.1073/pnas.77.2.795. [DOI] [PMC free article] [PubMed] [Google Scholar]
- Garel J. R., Nall B. T., Baldwin R. L. Guanidine-unfolded state of ribonuclease A contains both fast- and slow-refolding species. Proc Natl Acad Sci U S A. 1976 Jun;73(6):1853–1857. doi: 10.1073/pnas.73.6.1853. [DOI] [PMC free article] [PubMed] [Google Scholar]
- Goto Y., Hamaguchi K. Formation of the intrachain disulfide bond in the constant fragment of the immunoglobulin light chain. J Mol Biol. 1981 Mar 5;146(3):321–340. doi: 10.1016/0022-2836(81)90391-0. [DOI] [PubMed] [Google Scholar]
- Goto Y., Hamaguchi K. The role of the intrachain disulfide bond in the conformation and stability of the constant fragment of the immunoglobulin light chain. J Biochem. 1979 Nov;86(5):1433–1441. doi: 10.1093/oxfordjournals.jbchem.a132661. [DOI] [PubMed] [Google Scholar]
- Isenman D. E., Lancet D., Pecht I. Folding pathways of immunoglobulin domains. The folding kinetics of the Cgamma3 domain of human IgG1. Biochemistry. 1979 Jul 24;18(15):3327–3336. doi: 10.1021/bi00582a020. [DOI] [PubMed] [Google Scholar]
- Kanehisa M. I., Tsong T. Y. Mechanism of the multiphasic kinetics in the folding and unfolding of globular proteins. J Mol Biol. 1978 Sep 5;124(1):177–194. doi: 10.1016/0022-2836(78)90155-9. [DOI] [PubMed] [Google Scholar]
- Kato S., Okamura M., Shimamoto N., Utiyama H. Spectral evidence for a rapidly formed structural intermediate in the refolding kinetics of hen egg-white lysozyme. Biochemistry. 1981 Mar 3;20(5):1080–1085. doi: 10.1021/bi00508a006. [DOI] [PubMed] [Google Scholar]
- Kato S., Shimamoto N., Utiyama H. Identification and characterization of the direct folding process of hen egg-white lysozyme. Biochemistry. 1982 Jan 5;21(1):38–43. doi: 10.1021/bi00530a007. [DOI] [PubMed] [Google Scholar]
- Kim P. S., Baldwin R. L. Structural intermediates trapped during the folding of ribonuclease A by amide proton exchange. Biochemistry. 1980 Dec 23;19(26):6124–6129. doi: 10.1021/bi00567a027. [DOI] [PubMed] [Google Scholar]
- Konishi Y., Ooi T., Scheraga H. A. Regeneration of ribonuclease A from the reduced protein. Isolation and identification of intermediates, and equilibrium treatment. Biochemistry. 1981 Jul 7;20(14):3945–3955. doi: 10.1021/bi00517a001. [DOI] [PubMed] [Google Scholar]
- Kotelchuck D., Scheraga H. A. The influence of short-range interactions on protein onformation. II. A model for predicting the alpha-helical regions of proteins. Proc Natl Acad Sci U S A. 1969 Jan;62(1):14–21. doi: 10.1073/pnas.62.1.14. [DOI] [PMC free article] [PubMed] [Google Scholar]
- Levitt M., Chothia C. Structural patterns in globular proteins. Nature. 1976 Jun 17;261(5561):552–558. doi: 10.1038/261552a0. [DOI] [PubMed] [Google Scholar]
- Lewis P. N., Go N., Go M., Kotelchuck D., Scheraga H. A. Helix probability profiles of denatured proteins and their correlation with native structures. Proc Natl Acad Sci U S A. 1970 Apr;65(4):810–815. doi: 10.1073/pnas.65.4.810. [DOI] [PMC free article] [PubMed] [Google Scholar]
- Lewis P. N., Momany F. A., Scheraga H. A. Folding of polypeptide chains in proteins: a proposed mechanism for folding. Proc Natl Acad Sci U S A. 1971 Sep;68(9):2293–2297. doi: 10.1073/pnas.68.9.2293. [DOI] [PMC free article] [PubMed] [Google Scholar]
- Lewis P. N., Scheraga H. A. Predictions of structural homologies in cytochrome c proteins. Arch Biochem Biophys. 1971 Jun;144(2):576–583. doi: 10.1016/0003-9861(71)90363-8. [DOI] [PubMed] [Google Scholar]
- Lin L. N., Brandts J. F. Further evidence suggesting that the slow phase in protein unfolding and refolding is due to proline isomerization: a kinetic study of carp parvalbumins. Biochemistry. 1978 Sep 19;17(19):4102–4110. doi: 10.1021/bi00612a036. [DOI] [PubMed] [Google Scholar]
- McPhie P. Kinetic studies on the unfolding and refolding of pepsinogen in urea. The nature of the rate-limiting step. J Biol Chem. 1980 May 10;255(9):4048–4052. [PubMed] [Google Scholar]
- Saxena V. P., Wetlaufer D. B. Formation of three-dimensional structure in proteins. I. Rapid nonenzymic reactivation of reduced lysozyme. Biochemistry. 1970 Dec 8;9(25):5015–5023. doi: 10.1021/bi00827a028. [DOI] [PubMed] [Google Scholar]
- Schmid F. X. A native-like intermediate on the ribonuclease A folding pathway. 1. Detection by tyrosine fluorescence changes. Eur J Biochem. 1981;114(1):105–109. doi: 10.1111/j.1432-1033.1981.tb06179.x. [DOI] [PubMed] [Google Scholar]
- Schmid F. X., Baldwin R. L. Acid catalysis of the formation of the slow-folding species of RNase A: evidence that the reaction is proline isomerization. Proc Natl Acad Sci U S A. 1978 Oct;75(10):4764–4768. doi: 10.1073/pnas.75.10.4764. [DOI] [PMC free article] [PubMed] [Google Scholar]
- Schmid F. X., Baldwin R. L. Detection of an early intermediate in the folding of ribonuclease A by protection of amide protons against exchange. J Mol Biol. 1979 Nov 25;135(1):199–215. doi: 10.1016/0022-2836(79)90347-4. [DOI] [PubMed] [Google Scholar]
- Schmid F. X., Baldwin R. L. The rate of interconversion between the two unfolded forms of ribonuclease A does not depend on guanidinium chloride concentration. J Mol Biol. 1979 Sep 15;133(2):285–287. doi: 10.1016/0022-2836(79)90536-9. [DOI] [PubMed] [Google Scholar]
- Schmid F. X., Blaschek H. A native-like intermediate on the ribonuclease A folding pathway. 2. Comparison of its properties to native ribonuclease A. Eur J Biochem. 1981;114(1):111–117. doi: 10.1111/j.1432-1033.1981.tb06180.x. [DOI] [PubMed] [Google Scholar]
- Tanaka S., Scheraga H. A. Hypothesis about the mechanism of protein folding. Macromolecules. 1977 Mar-Apr;10(2):291–304. doi: 10.1021/ma60056a015. [DOI] [PubMed] [Google Scholar]
- Tanaka S., Scheraga H. A. Model of protein folding: inclusion of short-, medium-, and long-range interactions. Proc Natl Acad Sci U S A. 1975 Oct;72(10):3802–3806. doi: 10.1073/pnas.72.10.3802. [DOI] [PMC free article] [PubMed] [Google Scholar]
- Tanaka S., Scheraga H. A. Model of protein folding: incorporation of a one-dimensional short-range (Ising) model into a three-dimensional model. Proc Natl Acad Sci U S A. 1977 Apr;74(4):1320–1323. doi: 10.1073/pnas.74.4.1320. [DOI] [PMC free article] [PubMed] [Google Scholar]