Abstract
The energetics and efficiency of light-induced electron transfer across membranes is examined on a molecular level. It is found that the activation energies that control the efficiency are determined by the solvation energies of the charge-transfer states, the redox potentials of the donors and acceptors, and the dielectric relaxation of the system. The formalism developed allows one to assess the efficiency of any artificial photosynthetic system in terms of its molecular components and their local environment. It is pointed out that the key problem in designing an efficient photosynthetic system is the transfer of a charge through a low dielectric environment and that this problem cannot be overcome by choosing the position of the primary donor and acceptor in the membrane. It is predicted that artificial photosynthetic systems can be optimized by placing the acceptors in polar sites that provide a large effective dielectric constant and low dielectric relaxation and by arranging the acceptors in order of increasing redox potentials. The implication regarding bacterial photosynthesis is discussed.
Keywords: photosynthesis, light energy storage, charge separation, bioenergetics
Full text
PDF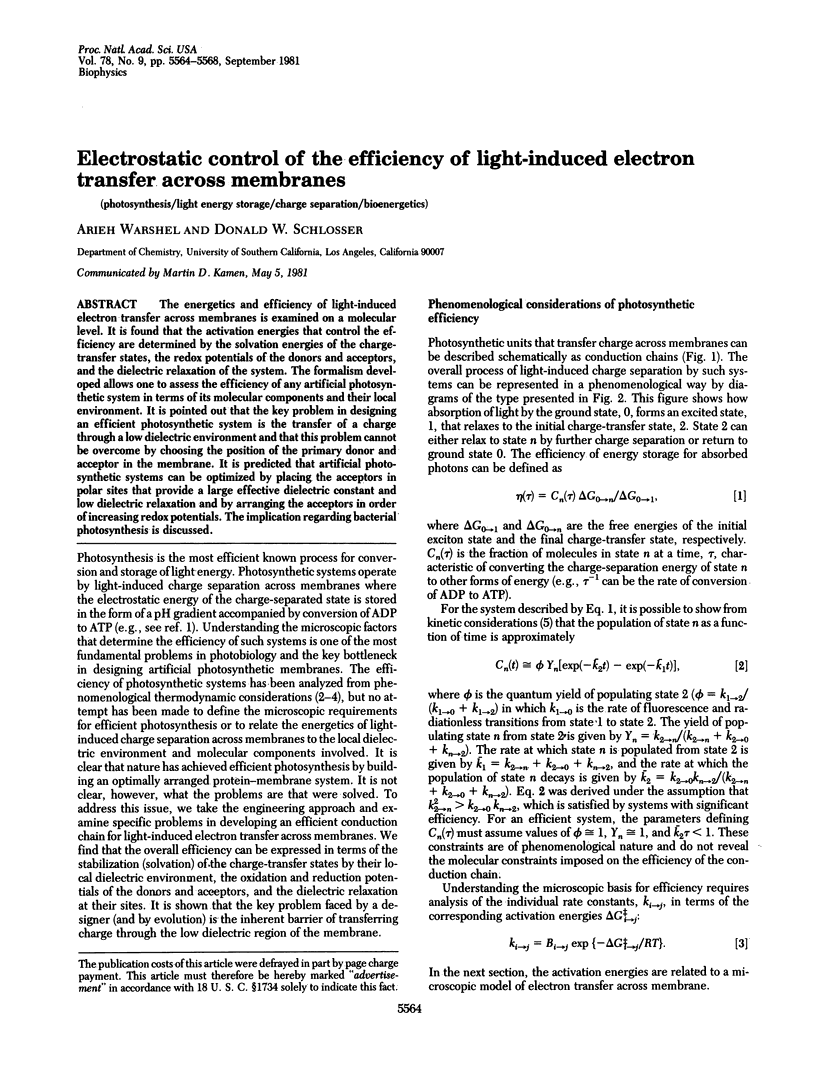
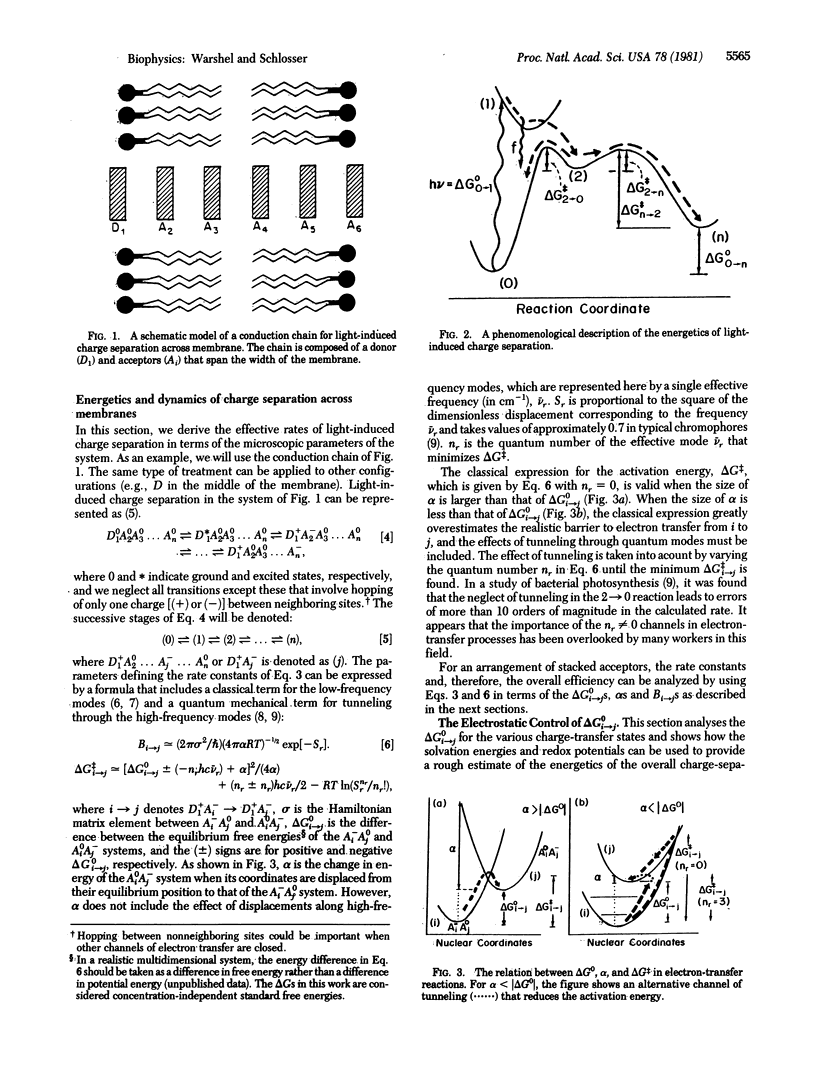
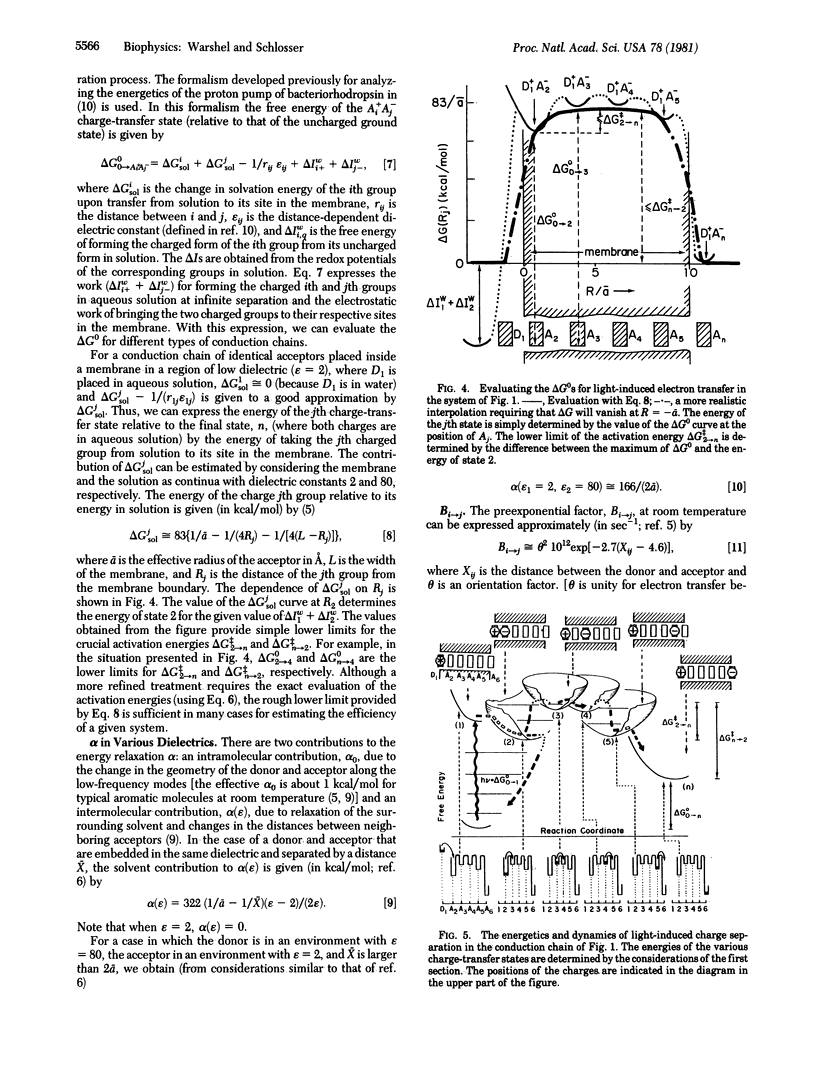
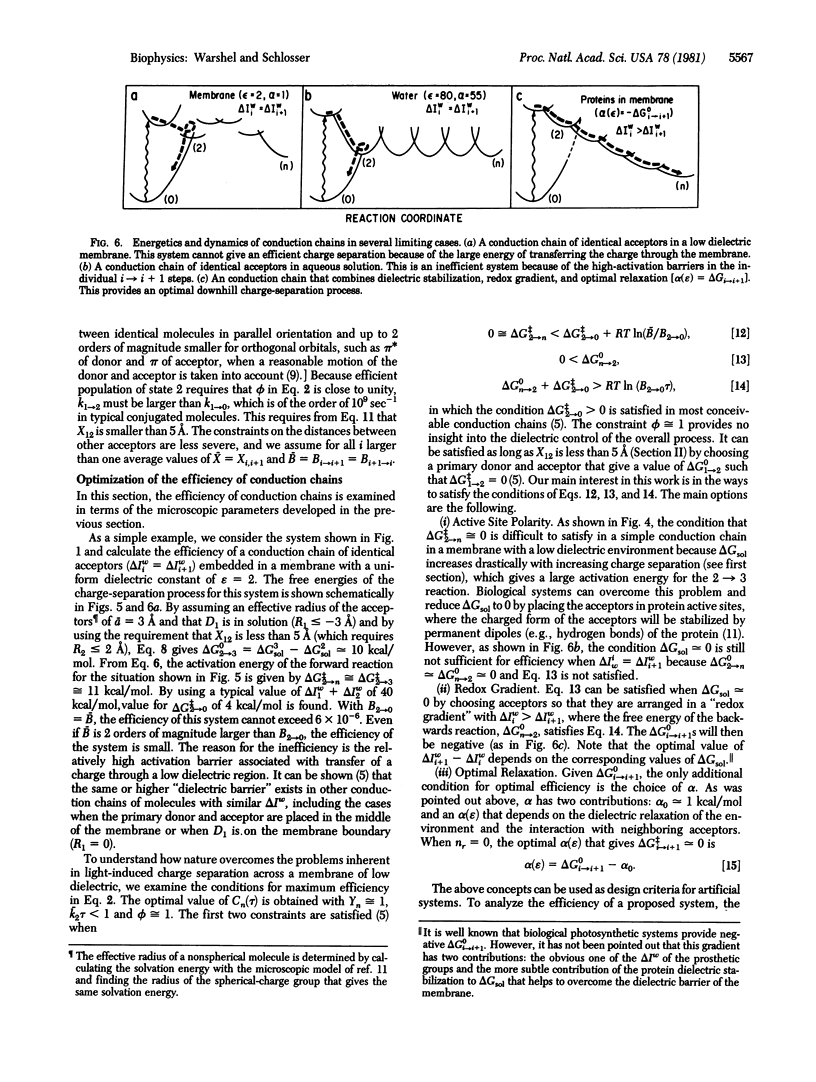
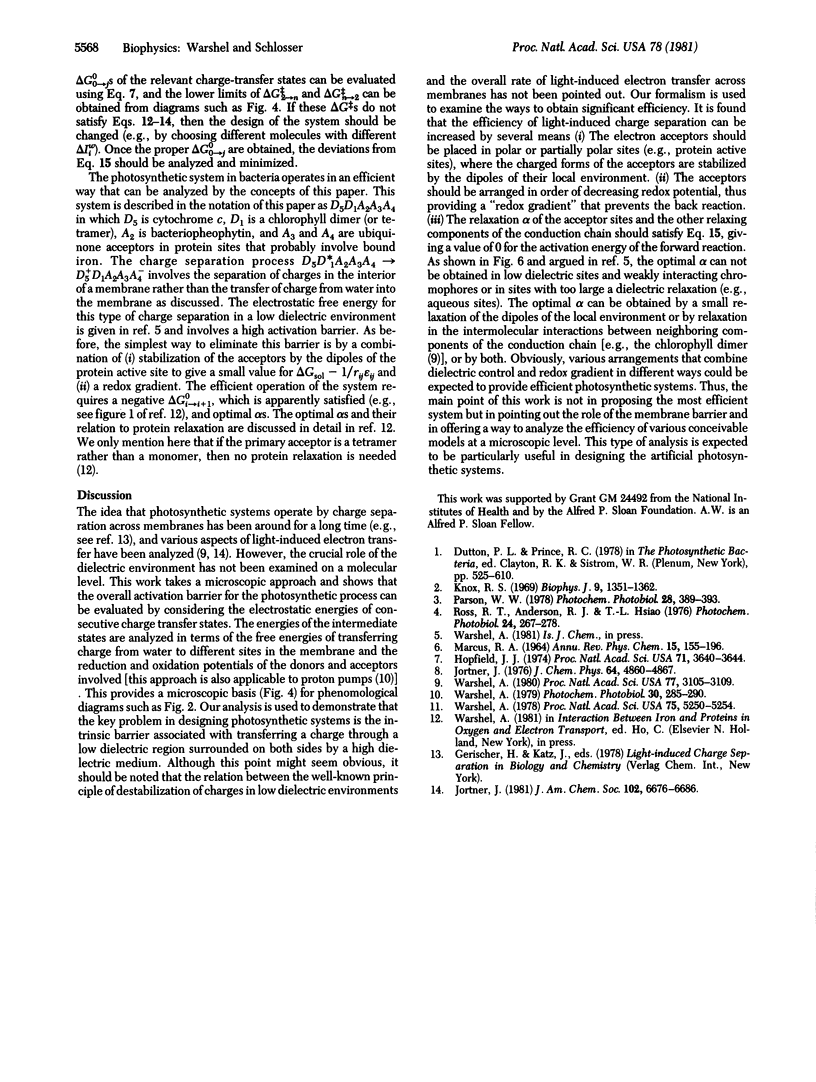
Selected References
These references are in PubMed. This may not be the complete list of references from this article.
- Hopfield J. J. Electron transfer between biological molecules by thermally activated tunneling. Proc Natl Acad Sci U S A. 1974 Sep;71(9):3640–3644. doi: 10.1073/pnas.71.9.3640. [DOI] [PMC free article] [PubMed] [Google Scholar]
- Knox R. S. Thermodynamics and the primary processes of photosynthesis. Biophys J. 1969 Nov;9(11):1351–1362. doi: 10.1016/S0006-3495(69)86457-X. [DOI] [PMC free article] [PubMed] [Google Scholar]
- Warshel A. Conversion of light energy to electrostatic energy in the proton pump of Halobacterium halobium. Photochem Photobiol. 1979 Aug;30(2):285–290. doi: 10.1111/j.1751-1097.1979.tb07148.x. [DOI] [PubMed] [Google Scholar]
- Warshel A. Energetics of enzyme catalysis. Proc Natl Acad Sci U S A. 1978 Nov;75(11):5250–5254. doi: 10.1073/pnas.75.11.5250. [DOI] [PMC free article] [PubMed] [Google Scholar]
- Warshel A. Role of the chlorophyll dimer in bacterial photosynthesis. Proc Natl Acad Sci U S A. 1980 Jun;77(6):3105–3109. doi: 10.1073/pnas.77.6.3105. [DOI] [PMC free article] [PubMed] [Google Scholar]